Information
- Author Services

Initiatives
You are accessing a machine-readable page. In order to be human-readable, please install an RSS reader.
All articles published by MDPI are made immediately available worldwide under an open access license. No special permission is required to reuse all or part of the article published by MDPI, including figures and tables. For articles published under an open access Creative Common CC BY license, any part of the article may be reused without permission provided that the original article is clearly cited. For more information, please refer to https://www.mdpi.com/openaccess .
Feature papers represent the most advanced research with significant potential for high impact in the field. A Feature Paper should be a substantial original Article that involves several techniques or approaches, provides an outlook for future research directions and describes possible research applications.
Feature papers are submitted upon individual invitation or recommendation by the scientific editors and must receive positive feedback from the reviewers.
Editor’s Choice articles are based on recommendations by the scientific editors of MDPI journals from around the world. Editors select a small number of articles recently published in the journal that they believe will be particularly interesting to readers, or important in the respective research area. The aim is to provide a snapshot of some of the most exciting work published in the various research areas of the journal.
Original Submission Date Received: .
- Active Journals
- Find a Journal
- Proceedings Series
- For Authors
- For Reviewers
- For Editors
- For Librarians
- For Publishers
- For Societies
- For Conference Organizers
- Open Access Policy
- Institutional Open Access Program
- Special Issues Guidelines
- Editorial Process
- Research and Publication Ethics
- Article Processing Charges
- Testimonials
- Preprints.org
- SciProfiles
- Encyclopedia
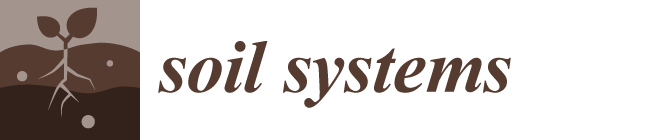
Article Menu
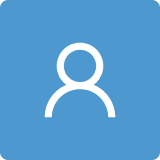
- Subscribe SciFeed
- Recommended Articles
- Google Scholar
- on Google Scholar
- Table of Contents
Find support for a specific problem in the support section of our website.
Please let us know what you think of our products and services.
Visit our dedicated information section to learn more about MDPI.
JSmol Viewer
Soil health assessment and management: recent development in science and practices.
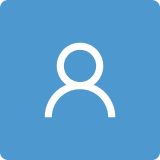
1. Introduction
2. the evolution of the scientific soil health system, 3. soil health assessment methods, 3.1. farmer perceptions of soil health, 3.2. soil health card methods, 3.3. solvita soil health tests, 3.4. haney soil health test, 3.5. comprehensive assessment of soil health (cash), 4. soil health management, 4.1. soil health principles, 4.2. best soil health management practices, 4.2.1. proper land use, 4.2.2. crop rotation, 4.2.3. cover crops, 4.2.4. conservation tillage, 4.2.5. soil organic amendment, 4.2.6. crop-range-livestock integration and rotational grazing, 5. summary and conclusions, institutional review board statement, informed consent statement, data availability statement, conflicts of interest.
- Weil, R.R.; Brady, N.C. The Nature and Properties of Soils ; Prentice Hall: Upper Saddle River, NJ, USA, 2017. [ Google Scholar ]
- USDA-NRCS. Soil Health ; Natural Resources Conservation Service, U.S. Department of Agriculture: Washington, DC, USA, 2019. Available online: https://www.nrcs.usda.gov/wps/portal/nrcs/main/soils/health/ (accessed on 11 August 2021).
- Doran, J.W.; Zeiss, M.R. Soil health and sustainability: Managing the biotic component of soil quality. Appl. Soil Ecol. 2000 , 15 , 3–11. [ Google Scholar ] [ CrossRef ] [ Green Version ]
- UNFAO. FAO Soils Portal-Degradation/Restoration ; Food and Agriculture Organization of the United Nations: Rome, Italy, 2021; Available online: http://www.fao.org/soils-portal/soil-degradation-restoration/en/ (accessed on 12 August 2021).
- Nunes, F.C.; Alves, L.J.; Carvalho, C.C.N.; Gross, E.; Majeti, P.; de Marchi Soares, T. Soil as a complex ecological system for meeting food and nutritional security. In Climate Change and Soil Interactions ; Prasad, M.N.V., Pietrzykowski, M., Eds.; Elsevier: Amsterdam, The Netherlands, 2020; pp. 229–269. [ Google Scholar ]
- Williams, H.; Colombi, T.; Keller, T. The influence of soil management on soil health: An on-farm study in southern Sweden. Geoderma 2020 , 360 , 114010. [ Google Scholar ] [ CrossRef ]
- Pereira, P.; Brevik, E.C.; Munoz-Rojas, M.; Miller, B.A. (Eds.) Soil mapping and processes modeling for sustainable land management. In Soil Mapping and Process Modeling for Sustainable Land Use Management ; Elsevier: Amsterdam, The Netherlands, 2017; pp. 29–60. [ Google Scholar ]
- Guo, M. The 3R principles for applying biochar to improve soil health. Soil Syst. 2020 , 4 , 9. [ Google Scholar ] [ CrossRef ] [ Green Version ]
- Kibblewhite, M.G.; Ritz, K.; Swift, M.J. Soil health in agricultural systems. Phil. Trans. R. Soc. B 2008 , 363 , 685–701. [ Google Scholar ] [ CrossRef ] [ Green Version ]
- Allen, D.E.; Singh, B.P.; Dalal, R.C. Soil health indicators under climate change: A review of current knowledge. In Soil Health and Climate Change ; Singh, B.P., Cowie, A.L., Chan, K.Y., Eds.; Springer: Berlin, Germany, 2011; pp. 25–45. [ Google Scholar ]
- Van Bruggena, A.H.C.; Semenov, A.M. In search of biological indicators for soil health and disease suppression. Appl. Soil Ecol. 2000 , 15 , 13–24. [ Google Scholar ] [ CrossRef ]
- Negueira Cardoso, E.J.B.; FigueiredoVasconcellos, R.L.; Bini, D.; Horta Miyauchi, M.Y.; Alcantara, C.; Alves, P.; de Paula, A.; Shigueyoshi Nakatani, A.; de Moraes, J.; Nogueira, M. Soil health: Looking for suitable indicators. What should be considered to assess the effects of use and management on soil health? Sci. Agric. 2013 , 70 , 274–289. [ Google Scholar ] [ CrossRef ] [ Green Version ]
- Moebius-Clune, B.N.; Moebiue-Clune, D.J.; Gugino, B.K.; Idowu, O.; Schindelbeck, R.; Rostow, A.; van Es, H.; Thies, J.; Shyler, H.; McBride, M.; et al. Comprehensive Assessment of Soil Health , 3rd ed.; Cornell University: Ithaca, NY, USA, 2017; pp. 19–101. [ Google Scholar ]
- USDA-NRCS. Soil Health Assessment ; Natural Resources Conservation Service, U.S. Department of Agriculture: Washington, DC, USA, 2019. Available online: https://www.nrcs.usda.gov/wps/portal/nrcs/main/soils/health/assessment (accessed on 15 August 2021).
- USDA-NRCS. Soil Health Card ; Natural Resources Conservation Service, U.S. Department of Agriculture: Washington, DC, USA, 2019. Available online: https://www.nrcs.usda.gov/wps/portal/nrcs/detail/soils/health/assessment/?cid=nrcs142p2_053871 (accessed on 15 August 2021).
- Woods End Laboratories. Solvita Soil Health Tests ; Woods End Laboratories, Inc.: Mount Vernon, ME, USA, 2021; Available online: https://solvita.com/soil/ (accessed on 15 August 2021).
- Ward Laboratories. Haney Test ; Ward Laboratories, Inc.: Kearney, NE, USA, 2021; Available online: https://www.wardlab.com/haney-test/ (accessed on 15 August 2021).
- Norris, C.E.; Congreves, K.A. Alternative management practices improve soil health indices in intensive vegetable cropping systems: A review. Front. Environ. Sci. 2018 , 6 , 50. [ Google Scholar ] [ CrossRef ]
- Idowu, J.; Ghimire, R.; Flynn, R.; Ganguli, A. Soil Health—Importance, Assessment, and Management. Circular 694B ; New Mexico State University Cooperative Extension Service: Las Cruces, NM, USA, 2019; Available online: https://ucanr.edu/sites/harecBETA/files/321673.pdf (accessed on 17 August 2021).
- PennState Extension. Managing Soil Health: Concepts and Practices ; Pennsylvania State University Cooperative Extension Service: University Park, PA, USA, 2017; Available online: https://extension.psu.edu/managing-soil-health-concepts-and-practices (accessed on 17 August 2021).
- USDA-NRCS. Soil Health Management ; Natural Resources Conservation Service, U.S. Department of Agriculture: Washington, DC, USA, 2021. Available online: https://www.nrcs.usda.gov/wps/portal/nrcs/main/soils/health/mgnt/ (accessed on 17 August 2021).
- Miner, G.L.; Delgado, J.A.; Ippolito, J.A.; Stewart, C.E. Soil health management practices and crop productivity. Agric. Environ. Lett. 2020 , 5 , e20023. [ Google Scholar ] [ CrossRef ]
- Lehmann, J.; Bossio, D.A.; Kogel-Knabner, I.; Rillig, M.C. The concept and future prospects of soil health. Nat. Rev. Earth Environ. 2020 , 1 , 544–553. [ Google Scholar ] [ CrossRef ]
- Bunemann, E.K.; Bongiorno, G.; Bai, Z.; Creamer, R.; Deyn, G.; de Goede, R.; Fleskens, L.; Geissen, V.; Kuyper, T.; Mader, P.; et al. Soil quality-A critical review. Soil Biol. Biochem. 2018 , 120 , 105–125. [ Google Scholar ] [ CrossRef ]
- Brevik, E.C. A Brief History of the Soil Health Concept ; Soil Science Society of America: Madison, WI, USA, 2018; Available online: https://profile.soils.org/files/soil-communication/documents/95_document1_d50a8524-dbf4-4856-8c62-4a03ede6c3d4.pdf (accessed on 17 August 2021).
- Wallace, H.A. Relation Between Livestock Farming and the Fertility of the Land. Retrospective Theses and Dissertations. 98. Iowa State University, Ames, IA, USA, 1910. Available online: https://lib.dr.iastate.edu/rtd/98 (accessed on 2 October 2021).
- Romig, D.E.; Garlynd, M.J.; Harris, R.F.; McSweeney, K. How farmers assess soil health and quality. J. Soil Water Cons. 1995 , 50 , 229–236. [ Google Scholar ]
- Doran, J.W.; Parkin, T.B. Defining and assessing soil quality. In Defining Soil Quality for a Sustainable Environment ; SSSA Spec. Pub. 35; Doran, J.W., Coleman, D.C., Bezdicek, D.F., Stewart, B.A., Eds.; Soil Science Society of America: Madison, WI, USA, 1994; pp. 3–21. [ Google Scholar ]
- Doran, J.W.; Sarrantonio, M.; Liebig, M.A. Soil health and sustainability. Adv. Agron. 1996 , 56 , 1–54. [ Google Scholar ]
- Sojka, R.E.; Upchurch, D.R. Reservations regarding the soil quality concept. Soil Sci. Soc. Am. J. 1999 , 63 , 1039–1054. [ Google Scholar ] [ CrossRef ] [ Green Version ]
- European Commission. Causes of the 2007–2008 Global Food Crisis Identified ; European Commission DG Environment News Alert Service: Bristol, UK, 2011; Available online: https://ec.europa.eu/environment/integration/research/newsalert/pdf/225na1_en.pdf (accessed on 19 August 2021).
- Lal, R. Soil carbon sequestration impacts on global climate change and food security. Science 2004 , 304 , 1623–1627. [ Google Scholar ] [ CrossRef ] [ Green Version ]
- McBratney, A.; Field, D.J.; Koch, A. The dimensions of soil security. Geoderma 2014 , 213 , 203–213. [ Google Scholar ] [ CrossRef ] [ Green Version ]
- Norris, C.E.; Bean, G.C.; Cappellazzi, S.B.; Cope, M.; Greub, K.; Liptzin, D.; Rieke, E.; Tracy, P.; Morgan, C.; Honeycutt, C.W. Introducing the North American project to evaluate soil health. Agron. J. 2020 , 112 , 3195–3215. [ Google Scholar ] [ CrossRef ]
- Karlen, D.L.; Venum, K.S.; Sudduth, K.A.; Obrycki, J.F.; Nunes, M.R. Soil health assessment: Past accomplishments, current activities, and future opportunities. Soil Till. Res. 2019 , 195 , 104365. [ Google Scholar ] [ CrossRef ]
- USDA-NRCS. Soil Quality Indicator Sheets ; Natural Resources Conservation Service, U.S. Department of Agriculture: Washington, DC, USA, 2015. Available online: https://www.nrcs.usda.gov/wps/portal/nrcs/detail/soils/health/assessment/?cid=stelprdb1237387 (accessed on 20 August 2021).
- SHI. North American Project to Evaluate Soil Health Measurements ; Soil Health Institute: Morrisville, NC, USA, 2021; Available online: https://soilhealthinstitute.org/north-american-project-to-evaluate-soil-health-measurements/ (accessed on 15 August 2021).
- SHI. National Soil Health Measurements to Accelerate Agricultural Transformation ; Soil Health Institute: Morrisville, NC, USA, 2021; Available online: https://soilhealthinstitute.org/national-soil-health-measurements-accelerate-agricultural-transformation/ (accessed on 21 August 2021).
- USDA-NRCS. Recommended Soil Health Indicators and Associated Laboratory Procedures ; Soil Health Technical Note No. 450-03; Natural Resources Conservation Service, U.S. Department of Agriculture: Washington, DC, USA, 2019.
- Nunes, M.R.; Veum, K.S.; Parker, P.A.; Holan, S.; Karlen, D.; Amsili, J.; van Es, H.; Wills, S.; Seybold, C.; Moorman, T. The soil health assessment protocol and evaluation applied to soil organic carbon. Soil Sci. Soc. Am. J. 2021 , 85 , 1–18. [ Google Scholar ] [ CrossRef ]
- Paz-Ferreiro, J.; Fu, S. Biological indices for soil quality evaluation: Perspectives and limitations. Land Degrad. Dev. 2016 , 27 , 14–25. [ Google Scholar ] [ CrossRef ]
- Wander, M.M.; Cihacek, L.J.; Coyne, M.; Drijber, R.; Grossman, J.; Gutknecht, J.; Horwath, W.; Jagamma, S.; Olk, D.; Ruark, M.; et al. Developments in agricultural soil quality and health: Reflections by the research committee on soil organic matter management. Front. Environ. Sci. 2019 , 7 , 109. [ Google Scholar ] [ CrossRef ] [ Green Version ]
- Andrews, S.S.; Karlen, D.L.; Cambardella, C.A. The soil management assessment framework: A quantitative soil quality evaluation method. Soil Sci. Soc. Am. J. 2004 , 68 , 1945–1962. [ Google Scholar ] [ CrossRef ]
- Veum, K.S.; Sudduth, K.A.; Kremer, R.J.; Kitchen, N.R. Sensor data fusion for soil health assessment. Geoderma 2017 , 305 , 53–61. [ Google Scholar ] [ CrossRef ]
- Jian, J.; Du, X.; Stewart, R.D. Quantifying cover crop effects on soil health and productivity. Data Brief 2020 , 29 , 105376. [ Google Scholar ] [ CrossRef ] [ PubMed ]
- SQI. Interpreting Soil Conditioning Index: A Tool for Measuring Soil Organic Matter Trends ; Soil Quality-Agronomy Technical Note No. 16; Soil Quality Institute: Auburn, AL, USA, 2003. Available online: https://www.nrcs.usda.gov/Internet/FSE_DOCUMENTS/nrcs142p2_053273.pdf (accessed on 22 August 2021).
- Wienhold, B.J.; Pikul, J.L.; Liebig, M.A.; Mikha, M.M.; Varvel, G.E.; Doran, J.W.; Andrews, S.S. Cropping system effects on soil quality in the Great Plains: Synthesis from a regional project. Renew. Agric. Food Syst. 2006 , 21 , 49–59. [ Google Scholar ] [ CrossRef ]
- USDA-NRCS. Soil Health Contacts ; Natural Resources Conservation Service, U.S. Department of Agriculture: Washington, DC, USA, 2021. Available online: https://www.nrcs.usda.gov/wps/portal/nrcs/detailfull/soils/health/?cid=stelprdb1237522 (accessed on 20 August 2021).
- SHI. About the Soil Health Institute ; Soil Health Institute: Morrisville, NC, USA, 2021; Available online: https://soilhealthinstitute.org/about-us/ (accessed on 22 August 2021).
- EC. Proposal for a Soil Health Mission: Caring for Soil is Caring for Life ; European Commission: Berlin, Germany, 2020; Available online: https://ec.europa.eu/jrc/en/science-update/proposal-soil-health-mission-caring-soil-caring-life (accessed on 22 August 2021).
- HAS. About Healthy Soil Australia ; Healthy Soil Australia: Dubbo, NSW, Australia, 2021; Available online: https://www.healthysoils.com.au/about (accessed on 22 August 2021).
- Maharjan, B.; Das, S.; Acharya, B.S. Soil health gap: A concept to establish a benchmark for soil health management. Glob. Ecol. Conserv. 2020 , 23 , e01116. [ Google Scholar ] [ CrossRef ]
- Jian, J.; Du, X.; Stewart, R.D. A database for global soil health assessment. Sci. Data 2020 , 7 , 16. [ Google Scholar ] [ CrossRef ] [ Green Version ]
- Karlen, D.L.; Goeser, N.J.; Veum, K.S.; Yost, M.A. On-farm soil health evaluations: Challenges and opportunities. J. Soil Water Conserv. 2017 , 72 , 26A–31A. [ Google Scholar ] [ CrossRef ] [ Green Version ]
- Veum, K.S.; Sudduth, K.E.; Kremer, R.J.; Kitchen, N.R. Estimating a soil quality index with VNIR reflectance spectroscopy. Soil Sci. Soc. Am. J. 2015 , 79 , 637–649. [ Google Scholar ] [ CrossRef ]
- Cho, Y.; Sheridan, A.H.; Sudduth, K.A.; Veum, K.S. Comparison of field and laboratory VNIR spectroscopy for profile soil property estimation. Trans. ASABE 2017 , 60 , 1503–1510. [ Google Scholar ] [ CrossRef ]
- Gruver, J.B.; Weil, R.R. Farmer perceptions of soil quality and their relationship to management-sensitive soil parameters. Renew. Agric. Food Syst. 2006 , 24 , 271–281. [ Google Scholar ] [ CrossRef ] [ Green Version ]
- Rekik, F.; van Es, H.; Hernandez-Aguilera, J.N.; Gomez, M.I. Understanding soil health and associated farmers’ perceptions in Columbian coffee systems. J. Soil Water Conserv. 2020 , 75 , 499–504. [ Google Scholar ] [ CrossRef ]
- USDA-NRCS. Soil Quality Card Design Guide ; Natural Resources Conservation Service, U.S. Department of Agriculture: Washington, DC, USA, 1999. Available online: https://www.nrcs.usda.gov/Internet/FSE_DOCUMENTS/nrcs142p2_051879.pdf (accessed on 24 August 2021).
- MDSHC. Maryland Soil Health Card ; Maryland Department of Agriculture: Annapolis, MD, USA, 2018. Available online: https://mda.maryland.gov/resource_conservation/counties/SoilHealthCard.pdf (accessed on 24 August 2021).
- India DACFW. Soil Health Card ; Department of Agriculture, Cooperation, and Farmers Welfare: New Delhi, India, 2021. Available online: https://www.india.gov.in/spotlight/soil-health-card#tab=tab-1 (accessed on 24 August 2021).
- Woods End Laboratories. Soil Health and Nutrient Test Quick Guide ; Woods End Laboratories, Inc.: Mount Vernon, ME, USA, 2021; Available online: https://www.woodsend.com/wp-content/uploads/2021/05/Soil-Health-Quick-Guide-Vers-4.4.pdf (accessed on 15 August 2021).
- Six, J.; Elliott, E.T.; Paustian, K. Soil structure and soil organic matter. II. A normalized stability index and the effect of mineralogy. Soil Sci. Soc. Amer. J. 2000 , 64 , 1042–1049. [ Google Scholar ] [ CrossRef ]
- Carter, M.R.; Gregorich, E.G. Soil Sampling and Methods of Analysis , 2nd ed.; CRC Press: Boca Raton: FL, USA, 2007. [ Google Scholar ]
- Chahal, I.; Hooker, D.C.; Deen, B.; Janovicek, K.; van Eerd, L.L. Long-term effects of crop rotation, tillage, and fertilizer nitrogen on soil health indicators and crop productivity in a temperate climate. Soil Till. Res. 2021 , 213 , 105121. [ Google Scholar ] [ CrossRef ]
- Sullivan, D.M.; Granatstein, D. Are “Haney Tests” Meaningful Indicators of Soil Health and Estimators of Nitrogen Fertilizer Credits? Nutr. Dig. 2015 , 7 , 3, Western Extension/Education Region Activities Nutrient Management and Water Quality Committee (WERA-103): Moscow, ID, USA. Available online: https://www.vineyardteam.org/files/resources/Are%20Haney%20Tests%20Meaningful.pdf (accessed on 25 August 2021).
- Yost, M.A.; Veum, K.S.; Kitchen, N.R.; Sawyer, J.; Camberato, J.; Carter, P.; Ferguson, R.; Fernandez, F.; Franzen, D.; Laboski, C.; et al. Evaluation of the Haney soil health tool for corn nitrogen recommendations across eight Midwest states. J. Soil. Water Conserv. 2018 , 73 , 587–592. [ Google Scholar ] [ CrossRef ] [ Green Version ]
- Chu, M.; Singh, S.; Walker, F.R.; Buschermohle, M.; Jagadamma, S.; Singh, S.; Eash, N.; Funcan, L. Soil health and soil fertility assessment by the Haney soil health test in an agricultural soil in west Tennessee. Commun. Soil Sci. Plant Anal. 2019 , 50 , 1123–1131. [ Google Scholar ] [ CrossRef ]
- Congreves, K.A.; Hayes, A.; Verhallen, E.A.; van Eerd, L.L. Long-term impact of tillage and crop rotation on soil health at four temperate agroecosystems. Soil Till. Res. 2015 , 152 , 17–28. [ Google Scholar ] [ CrossRef ]
- Ye, R.; Parajuli, B.; Szogi, A.A.; Sigua, G.C.; Ducey, T.F. Soil health assessment after 40 years of conservation and conventional tillage management in Southeastern Coastal Plain soils. Soil Sci. Soc. Am. J. 2021 , 85 , 20246. [ Google Scholar ] [ CrossRef ]
- USDA-NRCS. Soil Health: Principle 1 of 5—Soil Armor ; Natural Resources Conservation Service, U.S. Department of Agriculture: Washington, DC, USA, 2016. Available online: https://www.nrcs.usda.gov/wps/portal/nrcs/detailfull/nd/soils/health/?cid=nrcseprd1300631 (accessed on 26 August 2021).
- USDA-NRCS. Honoring 86 Years of NRCS—A Brief History ; Natural Resources Conservation Service, U.S. Department of Agriculture: Washington, DC, USA, 2020. Available online: https://www.nrcs.usda.gov/wps/portal/nrcs/detail/national/about/history/?cid=nrcs143_021392 (accessed on 27 August 2021).
- Troeh, F.R.; Donahue, R.L.; Hobbs, J.A. Soil and Water Conservation for Productivity and Environmental Production ; Person Education: Upper Saddle River, NJ, USA, 2004. [ Google Scholar ]
- Berendsen, R.L.; Pieterse, C.M.J.; Bakker, P.A.H.M. The rhizosphere microbiome and plant health. Trends Plant Sci. 2012 , 17 , 478–486. [ Google Scholar ] [ CrossRef ]
- USDA-NRCS. Rotations for Pest Management ; Natural Resources Conservation Service, U.S. Department of Agriculture: Washington, DC, USA, 2009. Available online: https://www.nrcs.usda.gov/Internet/FSE_DOCUMENTS/nrcs142p2_017827.pdf (accessed on 28 August 2021).
- Chowdhury, S.; Bolan, N.; Farrell, M.; Sarkar, B.; Sarker, J.; Kirkham, M.; Hossain, Z.; Kim, G.-H. Role of cultural and nutrient management practices in carbon sequestration in agricultural soil. Adv. Agron. 2021 , 166 , 131–169. [ Google Scholar ]
- Van Eerd, L.L.; Congreves, K.A.; Hayes, A.; Verhallen, A.; Hooker, D.C. Long-term tillage and crop rotation effects on soil quality, organic carbon, and total nitrogen. Can. J. Soil Sci. 2014 , 94 , 303–315. [ Google Scholar ] [ CrossRef ]
- Hartwig, N.L.; Ammon, H.U. Cover crops and living mulches. Weed Sci. 2002 , 50 , 688–699. [ Google Scholar ] [ CrossRef ]
- Magdoff, F.; van Es, H. Cover crops. In Building Soils for Better Crops—Ecological Management for Healthy Soils , 4th ed.; Magdoff, F., van Es, H., Eds.; Sustainable Agriculture Research and Education: College Park, MD, USA, 2021; pp. 137–158. [ Google Scholar ]
- Lawson, A.; Cogger, C.; Bary, A.; Fortuna, A.M. Influence of seeding ratio, planting date, and termination date on rye-hairy vetch cover crop mixture performance under organic management. PLoS ONE 2015 , 10 , e0129597. [ Google Scholar ] [ CrossRef ]
- Vukicevich, E.; Lowery, T.; Bowen, P.; Úrbez-Torres, J.R.; Hart, M. Cover crops to increase soil microbial diversity and mitigate decline in perennial agriculture. A review. Agron. Sustain. Dev. 2016 , 36 , 48. [ Google Scholar ] [ CrossRef ] [ Green Version ]
- Sharma, P.; Singh, A.; Kahlon, C.; Brar, A.; Grover, K.; Dia, M.; Steiner, R. The role of cover crops towards sustainable soil health and agriculture—A review paper. Am. J. Plant Sci. 2018 , 9 , 1935–1951. [ Google Scholar ] [ CrossRef ] [ Green Version ]
- Finney, D.M.; Buyer, J.S.; Kaye, J.P. Living cover crops have immediate impacts on soil microbial community structure and function. J. Soil Water. Conserv. 2017 , 72 , 361–373. [ Google Scholar ] [ CrossRef ] [ Green Version ]
- Kumar, S.; Reitsma, K. Soil tillage. In iGrow Corn: Best Management Practices ; Clay, D.E., Carlson, C.G., Clay, S.A., Byamukama, E., Eds.; South Dakota State University: Brookings, SD, USA, 2019; Available online: https://extension.sdstate.edu/sites/default/files/2019-09/S-0003-11-Corn.pdf (accessed on 28 August 2021).
- Nunes, M.; Karlen, D.L.; Veum, K.S.; Moorman, T.B. Biological soil health indicators respond to tillage intensity: A US meta analysis. Geoderma 2020 , 369 , 114335. [ Google Scholar ] [ CrossRef ]
- Fangueiro, D.; Becerra, D.; Albarrán, A.; Pena, D.; Sanchez-llerena, J.; Nunes, J.M.; Lopez Pineiro, A. Effect of tillage and wáter management on GHG emissions from Mediterranean rice growing ecosystems. Atmos. Environ. 2017 , 150 , 303–312. [ Google Scholar ] [ CrossRef ]
- Pieper, J.R.; Brown, R.N.; Amador, J.A. Effects of three conservation tillage strategies on yields and soil health in a mixed vegetable production system. HortScience 2015 , 50 , 1770–1776. [ Google Scholar ] [ CrossRef ]
- Zahid, A.; Ali, S.; Ahmed, M.; Iqbal, N. Improvement of soil health through residue management and conservation tillage in rice-wheat cropping system of Punjab, Pakistan. Agronomy 2020 , 10 , 1844. [ Google Scholar ] [ CrossRef ]
- Cooper, R.J.; Hama-Aziz, Z.Q.; Hiscock, K.M. Conservation tillage and soil health: Lessons from a 5-year UK farm trial (2013-2018). Soil Till. Res. 2020 , 202 , 104648. [ Google Scholar ] [ CrossRef ]
- Courtney, R.G.; Mullen, G.J. Soil quality and barley growth as influenced by the land application of two compost types. Bioresour. Technol. 2008 , 99 , 2913–2918. [ Google Scholar ] [ CrossRef ]
- Guo, M.; Chorover, J.; Fox, R.; Rosario, R. Leachate chemistry of field-weathered spent mushroom substrate. J. Environ. Qual. 2001 , 30 , 1699–1709. [ Google Scholar ] [ CrossRef ] [ Green Version ]
- Guo, M.; Tongtavee, N.; Labreveux, M. Nutrient dynamics of field-weathered Delmarva poultry litter: Implications for land application. Biol. Fertil. Soils 2009 , 45 , 829–838. [ Google Scholar ] [ CrossRef ]
- Guo, M.; Song, W.; Kazda, R. Fertilizer value of lime-stabilized biosolids as a soil amendment. Agron. J. 2012 , 104 , 1679–1686. [ Google Scholar ] [ CrossRef ] [ Green Version ]
- Song, W.; Guo, M. Quality variations of poultry litter biochars generated at different pyrolysis temperatures. J. Anal. Appl. Pyrolysis 2012 , 94 , 138–145. [ Google Scholar ] [ CrossRef ]
- Sekaran, U.; Kumar, S.; Gonzalez-Hernandez, J.L. Integration of crop and livestock enhanced soil biochemical properties and microbial community structure. Geoderma 2020 , 381 , 114686. [ Google Scholar ] [ CrossRef ]
- Undersander, D.; Albert, B.; Cosgrove, D.; Johnson, D.; Peterson, P. Pastures for Profit: A Guide to Rotational Grazing ; A3529; University of Wisconsin Cooperative Extension: Madison, WI, USA, 2002. Available online: https://www.nrcs.usda.gov/Internet/FSE_DOCUMENTS/stelprdb1097378.pdf (accessed on 28 August 2021).
Click here to enlarge figure
Soil Health Indicator | Soil Function | ||||
---|---|---|---|---|---|
Sustain Biological Diversity, Activity, and Productivity | Regulate and Partition Water and Solute Flow | Filter, Buffer, Degrade, Detoxify Organic and Inorganic Materials | Store and Cycle Nutrients and Carbon | Physical Stability and Support for Plants and Engineering Structures | |
SAS | ++++ | ++++ | ++++ | +++++ | |
AWC | ++++++ | +++++ | ++++ | ||
Bulk density | +++++ | ++++++ | ++ | ++++++ | |
Earthworms | ++++++ | ++++++ | ++++++ | ++++++ | |
Infiltration | ++++ | ++ | |||
POM | ++++++ | ++++++ | ++++++ | ++++++ | ++++++ |
PMN | ++++++ | ++++++ | |||
Reactive C | ++++ | ++ | ++++++ | ++++ | ++++ |
Slaking | ++ | +++++ | |||
Soil crusts | +++++ | ||||
Soil EC | ++++++ | ||||
Soil enzymes | ++++++ | ++++++ | |||
Soil nitrate | ++ | ++ | |||
Soil pH | ++++ | ++++++ | ++++++ | ++++++ | |
Soil respiration | ++++++ | ++ | ++++++ | ++++ | |
Soil structure | +++ | +++ | ++ | ++ | ++++ |
TOC | ++++++ | ++++++ | ++++++ | ++++++ | ++++++ |
Soil Health Indicators | Criteria | Examples |
---|---|---|
Tier 1 | Soil texture Soil bulk density Soil aggregate stability Available water-holding capacity Saturated hydraulic conductivity Soil pH Soil electrical conductivity Cation exchange capacity Base saturation Extractable P, Ca, Mg, K, Fe, Mn, Cu, Zn Extractable Al, As, B, Ba, Cd, Co, Cr, Mo, Ni, Pb, Si, Sr Soil total nitrogen content Nitrogen mineralization rate Soil organic carbon content Short-term carbon mineralization Crop yield | |
Tier 2 | Soil sodium adsorption ratio Macro-aggregate stability Soil stability index Soil active carbon Soil protein index Soil β-glucosidase Soil N-acetyl-β-D glucosaminidase Soil phosphomonoesterase Soil arylsulfatase Soil phospholipid fatty acid (PLFA) profile Soil fatty acid methyl ester (FAME) profile Soil microbial genomics Soil reflectance | |
Tier 3 | Soil microbial community structure Soil microbial DNA extraction and sequencing |
Tillage System | Soil Conditions Prior to Planting | Operation | Weed Control Methods |
---|---|---|---|
Ridge till | Undisturbed; ridge scalp at planting | Till land using a sweep cultivator to form 10–15 cm height ridges; seeds are planted in ridges | Combined herbicide application and soil cultivation |
Strip till | Undisturbed; strip till at planting | Narrow and shallow tillage using a rotary tiller or an in-row chisel planter; ~1/3 surface tilled at planting | Combined herbicide application and soil cultivation |
Stubble mulch till | Tilled—residues ≥ 30% | Use a chisel tiller, a blade plow, or a sweep cultivator to till stubble-covered land | Combined herbicide application and soil cultivation |
Reduced till | Tilled—residues ≥ 30% | Reduce till of cropland using a chisel tiller, a disc plow, or a blade plow to prepare seed beds | Combined herbicide application and soil cultivation |
No till | Undisturbed | Use a drill planter (no-till seeder) to sow seeds | Herbicides |
MDPI stays neutral with regard to jurisdictional claims in published maps and institutional affiliations. |
Share and Cite
Guo, M. Soil Health Assessment and Management: Recent Development in Science and Practices. Soil Syst. 2021 , 5 , 61. https://doi.org/10.3390/soilsystems5040061
Guo M. Soil Health Assessment and Management: Recent Development in Science and Practices. Soil Systems . 2021; 5(4):61. https://doi.org/10.3390/soilsystems5040061
Guo, Mingxin. 2021. "Soil Health Assessment and Management: Recent Development in Science and Practices" Soil Systems 5, no. 4: 61. https://doi.org/10.3390/soilsystems5040061
Article Metrics
Article access statistics, further information, mdpi initiatives, follow mdpi.
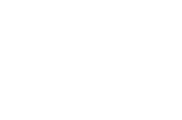
Subscribe to receive issue release notifications and newsletters from MDPI journals
Advertisement
Promoting soil health in organically managed systems: a review
- Published: 21 December 2019
- Volume 10 , pages 339–358, ( 2020 )
Cite this article
- Katherine L. Tully ORCID: orcid.org/0000-0002-6190-2679 1 &
- Cullen McAskill 1
3041 Accesses
49 Citations
12 Altmetric
Explore all metrics
Soil health is an old concept receiving renewed attention. Defined as a soil’s capacity to function, soil health is composed of physical, chemical, and biological attributes. The improvement and maintenance of soil health is considered a cornerstone of organic agriculture. Although there are numerous studies that compare organic systems with conventional systems, fewer studies compare organic systems with each other to determine how best to improve soil health metrics. In this review, we focused on nine indicators of soil health (aggregate stability, water holding capacity, infiltration/porosity, erosion/runoff, nutrient cycling, organic carbon, microbial biomass, macrofauna abundance, and weed seed bank). We found 153 peer-reviewed, published studies that measured these soil health indicators in two or more organic treatments. Overall, published research focused on four key practices: (1) cover crops, (2) organic amendments, (3) rotation diversity and length, and (4) tillage. Of these, 26 studies focused on cover crops, 77 on organic amendments, 32 on crop rotations, 40 on tillage, and 22 included more than one practice. Eighty percent of the studies were conducted in the USA and Europe. We found strong agreement in the literature that roll-killed cover crops suppressed weeds better than disking and that weed suppression required high levels of cover crop biomass. Combinations of organic amendments such as composts, manures, and vermicomposts improved soil health metrics compared to when applied alone. Including a perennial crop, like alfalfa, consistently improved soil carbon (C), nitrogen (N), and aggregate stability. Soil health metrics were improved under shallow, non-inversion tillage strategies compared with conventional tillage. Despite their importance for climate change mitigation and adaptation, the effect of practices on aggregate stability and water dynamics were under-studied compared with other soil health metrics. There is a great deal of variety and nuance to organic systems, and future research should focus on how to optimize practices within organic systems to improve and maintain soil health.
This is a preview of subscription content, log in via an institution to check access.
Access this article
Subscribe and save.
- Get 10 units per month
- Download Article/Chapter or eBook
- 1 Unit = 1 Article or 1 Chapter
- Cancel anytime
Price includes VAT (Russian Federation)
Instant access to the full article PDF.
Rent this article via DeepDyve
Institutional subscriptions
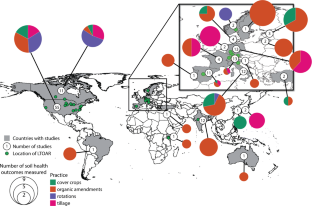
Similar content being viewed by others

Knowledge gaps in organic research: understanding interactions of cover crops and tillage for weed control and soil health
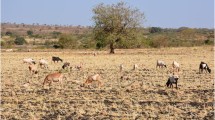
Soil functions and ecosystem services in conventional, conservation, and integrated agricultural systems. A review
Soil Indicators and Management Strategies for Environmental Sustainability
Explore related subjects.
- Environmental Chemistry
Adams PR III, Orr DB, Arellano C, Cardoza YJ (2017) Soil and foliar arthropod abundance and diversity in five cropping systems in the Coastal Plains of North Carolina. Environ Entomol 46:771–783. https://doi.org/10.1093/ee/nvx081
Article PubMed Google Scholar
Almagro M, Vente J, Boix-Fayos C, et al (2016) Sustainable land management practices as providers of several ecosystem services under rainfed Mediterranean agroecosystems. Mitig Adapt Strat Glob Change 1–15. https://doi.org/10.1007/s11027-013-9535-2
Anderson RL (2015) Integrating a complex rotation with no-till improves weed management in organic farming. A review. Agron Sust Dev 35:967–974. https://doi.org/10.1007/s13593-015-0292-3
Article Google Scholar
Armengot L, José-María L, Chamorro L, Sans FX (2013) Weed harrowing in organically grown cereal crops avoids yield losses without reducing weed diversity. Agron Sust Dev 33:405–411. https://doi.org/10.1007/s13593-012-0107-8
Badgley C, Moghtader J, Quintero E et al (2007) Organic agriculture and the global food supply. Renew Agric Food Syst 22:86–108. https://doi.org/10.1017/S1742170507001640
Baldivieso-Freitas P, Blanco-Moreno JM, Armengot L et al (2018) Crop yield, weed infestation and soil fertility responses to contrasted ploughing intensity and manure additions in a Mediterranean organic crop rotation. Soil Tillage Res 180:10–20. https://doi.org/10.1016/j.still.2018.02.006
Barbieri P, Pellerin S, Nesme T (2017) Comparing crop rotations between organic and conventional farming. Sci Rep 7:1–10. https://doi.org/10.1038/s41598-017-14271-6
Article CAS Google Scholar
Battany MC, Grismer ME (2000) Rainfall runoff and erosion in Napa Valley vineyards: effects of slope, cover and surface roughness. Hydrol Process 14:1289–1304. https://doi.org/10.1002/(sici)1099-1085(200005)14:7<1289::aid-hyp43>3.0.co;2-r
Benaragama D, Shirtliffe SJ, Gossen BD et al (2016) Long-term weed dynamics and crop yields under diverse crop rotations in organic and conventional cropping systems in the Canadian prairies. Field Crop Res 196:357–367. https://doi.org/10.1016/j.fcr.2016.07.010
Bengtsson J, Ahnstrom J, Weibull A-C (2005) The effects of organic agriculture on biodiversity and abundance: a meta-analysis. J Appl Ecol 42:261–269
Berner A, Hildermann I, Fliessbach A et al (2008) Crop yield and soil fertility response to reduced tillage under organic management. Soil Tillage Res 101:89–96. https://doi.org/10.1016/j.still.2008.07.012
Berti A, Morari F, Ferro ND et al (2016) Organic input quality is more important than its quantity: C turnover coefficients in different cropping systems. Eur J Agron 77:138–145. https://doi.org/10.1016/j.eja.2016.03.005
Bilalis DJ, Karamanos AJ (2010) Organic maize growth and mycorrhizal root colonization response to tillage and organic fertilization. J Sustain Agric 34:836–849. https://doi.org/10.1080/10440046.2010.519197
Blanco-Canqui H, Francis CA, Galusha TD (2017) Does organic farming accumulate carbon in deeper soil profiles in the long term? Geoderma 288:213–221. https://doi.org/10.1016/j.geoderma.2016.10.031
Bonnin I, Bonneuil C, Goffaux R et al (2014) Explaining the decrease in the genetic diversity of wheat in France over the 20th century. Agric Ecosyst Environ 195:183–192. https://doi.org/10.1016/j.agee.2014.06.003
Bradshaw B, Dolan H, Smit B (2004) Farm-level adaptation to climatic variability and change: crop diversification in the Canadian prairies. Clim Chang 67:119–141. https://doi.org/10.1007/s10584-004-0710-z
Braman S, Tenuta M, Entz MH (2016) Selected soil biological parameters measured in the 19th year of a long term organic-conventional comparison study in Canada. Agric Ecosyst Environ 233:343–351. https://doi.org/10.1016/j.agee.2016.09.035
Brush SB (1995) In situ conservation of landraces in centers of crop diversity. Crop Sci 35:346–354
Bullock DG (1992) Crop rotation. Crit Rev. Plant Sci 11:309–326
Bünemann EK, Bongiorno G, Bai Z et al (2018) Soil quality—a critical review. Soil Biol Biochem 120:105–125. https://doi.org/10.1016/j.soilbio.2018.01.030
Canali S, Campanelli G, Ciaccia C et al (2013) Conservation tillage strategy based on the roller crimper technology for weed control in Mediterranean vegetable organic cropping systems. Eur J Agron 50:11–18. https://doi.org/10.1016/j.eja.2013.05.001
Cavigelli MA, Teasdale JR, Conklin AE (2008) Long-term agronomic performance of organic and conventional field crops in the mid-Atlantic region. Agron J 100:785–710. https://doi.org/10.2134/agronj2006.0373
Cavigelli MA, Mirsky SB, Teasdale JR et al (2013a) Organic grain cropping systems to enhance ecosystem services. Renew Agric Food Syst 28:145–159. https://doi.org/10.1017/S1742170512000439
Cavigelli MA, Teasdale JR, Spargo JT (2013b) Increasing crop rotation diversity improves agronomic, economic, and environmental performance of organic grain cropping systems at the USDA-ARS Beltsville Farming Systems Project. Crop Manag. https://doi.org/10.1094/CM-2013-0429-02-PS
Cesarano G, De Filippis F, La Storia A et al (2017) Organic amendment type and application frequency affect crop yields, soil fertility and microbiome composition. Appl Soil Ecol 120:254–264. https://doi.org/10.1016/j.apsoil.2017.08.017
Chirinda N, Olesen JE, Porter JR (2008) Effects of organic matter input on soil microbial properties and crop yields in conventional and organic cropping systems. Modena, Italy, pp 1–4
Google Scholar
Chirinda N, Olesen JE, Porter JR, Schjønning P (2010) Soil properties, crop production and greenhouse gas emissions from organic and inorganic fertilizer-based arable cropping systems. Agric Ecosyst Environ 139:584–594. https://doi.org/10.1016/j.agee.2010.10.001
Chongtham IR, Bergkvist G, Watson CA et al (2016) Factors influencing crop rotation strategies on organic farms with different time periods since conversion to organic production. Biol Agric Hortic 33:14–27. https://doi.org/10.1080/01448765.2016.1174884
Christianson R, Christianson L, Wong C et al (2018) Beyond the nutrient strategies: common ground to accelerate agricultural water quality improvement in the upper Midwest. J Environ Manag 206:1072–1080. https://doi.org/10.1016/j.jenvman.2017.11.051
Ciaccia C, Canali S, Campanelli G et al (2015a) Effect of roller-crimper technology on weed management in organic zucchini production in a Mediterranean climate zone. Renew Agric Food Syst 31:111–121. https://doi.org/10.1017/S1742170515000046
Ciaccia C, Montemurro F, Campanelli G et al (2015b) Legume cover crop management and organic amendments application: effects on organic zucchini performance and weed competition. Sci Hortic 185:48–58. https://doi.org/10.1016/j.scienta.2015.01.011
Clark AJ, Decker AM, Meisinger JJ, McIntosh MS (1997) Kill date of vetch, rye, and a vetch-rye mixture: II. Soil moisture and corn yield. Agron J 89:434–441. https://doi.org/10.2134/agronj1997.00021962008900030011x
Colbach N, Tschudy C, Meunier D et al (2013) Weed seeds in exogenous organic matter and their contribution to weed dynamics in cropping systems. A simulation approach. Eur J Agron 45:7–19. https://doi.org/10.1016/j.eja.2012.10.006
Cooper J, Baranski M, Stewart G, Nobel-de Lange M, Bàrberi P, Fließbach A, Peigné J, Berner A, Brock C, Casagrande M, Crowley O, David C, Vliegher A, Döring TF, Dupont A, Entz M, Grosse M, Haase T, Halde C, Hammerl V, Huiting H, Leithold G, Messmer M, Schloter M, Sukkel W, Heijden MGA, Willekens K, Wittwer R, Mäder P (2016) Shallow non-inversion tillage in organic farming maintains crop yields and increases soil C stocks: a meta-analysis. Agron Sust Dev 36:1–20. https://doi.org/10.1007/s13593-016-0354-1
Crittenden SJ, de Goede RGM (2016) Integrating soil physical and biological properties in contrasting tillage systems in organic and conventional farming. Eur J Soil Biol 77:26–33. https://doi.org/10.1016/j.ejsobi.2016.09.003
Crittenden SJ, Poot N, Heinen M et al (2015) Soil physical quality in contrasting tillage systems in organic and conventional farming. Soil Tillage Res 154:136–144. https://doi.org/10.1016/j.still.2015.06.018
Dao TH, Schomberg HH, Cavigelli MA (2015) Tillage and rotational effects on exchangeable and enzyme-labile phosphorus forms in conventional and organic cropping systems. Nutr Cycl Agroecosyst 101:153–165. https://doi.org/10.1007/s10705-014-9665-2
Das A, Patel DP, Kumar M et al (2014) Influence of cropping systems and organic amendments on productivity and soil health at mid altitude of North East India. Indian J Agric Sci 84:1525–1530. https://doi.org/10.1007/978-3-319-23075-7_3
Dawoe EK, Quashie-Sam JS, Oppong SK (2013) Effect of land-use conversion from forest to cocoa agroforest on soil characteristics and quality of a Ferric Lixisol in lowland humid Ghana. Agrofor Syst 88:87–99. https://doi.org/10.1007/s10457-013-9658-1
de Cima DS, Tein B, Eremeev V et al (2016) Winter cover crop effects on soil structural stability and microbiological activity in organic farming. Biol Agric Horticu 32:1–12. https://doi.org/10.1080/01448765.2015.1130646
de Ponti T, Rijk B, van Ittersum MK (2012) The crop yield gap between organic and conventional agriculture. Agric Syst 108:1–9. https://doi.org/10.1016/j.agsy.2011.12.004
Delate K, Cambardella CA (2004) Agroecosystem performance during transition to certified organic grain production. Agron J 96:1288–1211. https://doi.org/10.2134/agronj2004.1288
Edgell J, Osmond DL, Line DE et al (2015) Comparison of surface water quality and yields from organically and conventionally produced sweet corn plots with conservation and conventional tillage. J Environ Qual 44:1861–1810. https://doi.org/10.2134/jeq2015.02.0074
Article CAS PubMed Google Scholar
Eltun R, Korsaeth A, Nordheim O (2002) A comparison of environmental, soil fertility, yield, and economical effects in six cropping systems based on an 8-year experiment in Norway. Agric Ecosyst Environ 90:155–168
Emmerling C (2007) Reduced and conservation tillage effects on soil ecological properties in an organic farming system. Biol Agric Hortic 24:363–377. https://doi.org/10.1080/01448765.2007.9755033
Evans R, Lawley Y, Entz MH (2016) Fall-seeded cereal cover crops differ in ability to facilitate low-till organic bean (Phaseolus vulgaris) production in a short-season growing environment. Field Crop Res 191:91–100. https://doi.org/10.1016/j.fcr.2016.02.020
Evanylo G, Sherony C, Spargo J et al (2008) Soil and water environmental effects of fertilizer-, manure-, and compost-based fertility practices in an organic vegetable cropping system. Agric Ecosyst Environ 127:50–58. https://doi.org/10.1016/j.agee.2008.02.014
Feaga JB, Selker JS, Dick RP, Hemphill DD (2010) Long-term nitrate leaching under vegetable production with cover crops in the Pacific northwest. Soil Sci Soc Am J 74:186–110. https://doi.org/10.2136/sssaj2008.0178
Feiziene D, Feiza V, Povilaitis V et al (2016) Soil sustainability changes in organic crop rotations with diverse crop species and the share of legumes. Acta Agriculturae Scandinavica, Section B - Soil & Plant Science 66:36–51. https://doi.org/10.1080/09064710.2015.1063683
Fernandez AL, Sheaffer CC, Wyse DL, Staley C, Gould TJ, Sadowsky MJ (2016) Associations between soil bacterial community structure and nutrient cycling functions in long-term organic farm soils following cover crop and organic fertilizer amendment. Sci Total Environ 566-567:949–959. https://doi.org/10.1016/j.scitotenv.2016.05.073
Ferro ND, Charrier P, Morari F (2013) Dual-scale micro-CT assessment of soil structure in a long-term fertilization experiment. Geoderma 204-205:84–93. https://doi.org/10.1016/j.geoderma.2013.04.012
FiBL-IFOAM (2017) The world of organic agriculture: statistics and emerging trends 2017. Research Institute for Organic Agriculture (FiBL) and Organics International (IFOAM), Bonn
Fliessbach A, Oberholzer H-R, Gunst L, Mäder P (2007) Soil organic matter and biological soil quality indicators after 21 years of organic and conventional farming. Agric Ecosyst Environ 118:273–284. https://doi.org/10.1016/j.agee.2006.05.022
Francis GS, Feller C, Harmand JM, Guibert H (2002) Management of soil organic matter in semiarid Africa for annual cropping systems. In: Managing organic matter in tropical soils: scope and limitations. Bonn, Germany
Franzluebbers AJ (2002) Water infiltration and soil structure related to organic matter and its stratification with depth. Soil Tillage Res 66:197–205. https://doi.org/10.1016/s0167-1987(02)00027-2
Frison EA, Cherfas J, Hodgkin T (2011) Agricultural biodiversity is essential for a sustainable improvement in food and nutrition security. Sustainability 3:238–253. https://doi.org/10.3390/su3010238
Gadermaier F, Berner A, Fliessbach A et al (2011) Impact of reduced tillage on soil organic carbon and nutrient budgets under organic farming. Renew Agric Food Syst 27:68–80. https://doi.org/10.1017/S1742170510000554
Gao S, Hoffman-Krull K, Bidwell AL, DeLuca TH (2016) Locally produced wood biochar increases nutrient retention and availability in agricultural soils of the San Juan Islands, USA. Agric Ecosyst Environ 233:43–54. https://doi.org/10.1016/j.agee.2016.08.028
Gao S, Hoffman-Krull K, DeLuca TH (2017) Soil biochemical properties and crop productivity following application of locally produced biochar at organic farms on Waldron Island, WA. Biogeochemistry 136:31–46. https://doi.org/10.1007/s10533-017-0379-9
Gattinger A, Muller A, Haeni M (2012) Enhanced top soil carbon stocks under organic farming. Proc Natl Acad Sci U S A 109(44):18226–18231
Gaudin ACM, Janovicek K, Deen B, Hooker DC (2015a) Wheat improves nitrogen use efficiency of maize and soybean-based cropping systems. Agric Ecosyst Environ 210:1–10. https://doi.org/10.1016/j.agee.2015.04.034
Gaudin ACM, Tolhurst TN, Ker AP et al (2015b) Increasing crop diversity mitigates weather variations and improves yield stability. PLoS One 10:e0113261–e0113220. https://doi.org/10.1371/journal.pone.0113261
Article CAS PubMed PubMed Central Google Scholar
Gliessman S (2014) Agroecology: the ecology of sustainable food systems, 3rd edn. Taylor & Francis Group, Boca Raton
Book Google Scholar
Gomiero T, Pimentel D, Paoletti MG (2011) Environmental impact of different agricultural management practices: conventional vs. organic agriculture. Crit Rev. Plant Sci 30:95–124. https://doi.org/10.1080/07352689.2011.554355
González-Peñaloza FA, Cerdà A, Zavala LM et al (2012) Do conservative agriculture practices increase soil water repellency? A case study in citrus-cropped soils. Soil Tillage Res 124:233–239. https://doi.org/10.1016/j.still.2012.06.015
Grandy AS, Robertson GP (2007) Land-use intensity effects on soil organic carbon accumulation rates and mechanisms. Ecosystems 10:59–74. https://doi.org/10.1007/s10021-006-9010-y
Gray LC, Morant P (2003) Reconciling indigenous knowledge with scientific assessment of soil fertility changes in southwestern Burkina Faso. Geoderma 111:425–437. https://doi.org/10.1016/s0016-7061(02)00275-6
Gruber S, Claupein W (2009) Effect of tillage intensity on weed infestation in organic farming. Soil Tillage Res 105:104–111. https://doi.org/10.1016/j.still.2009.06.001
Gurr GM, Wratten SD, Luna JM (2003) Multi-function agricultural biodiversity: pest management and other benefits. Basic Appl Ecol 4:107–116. https://doi.org/10.1078/1439-1791-00122
Hadas A, Kautsky L, Goek M, Erman Kara E (2004) Rates of decomposition of plant residues and available nitrogen in soil, related to residue composition through simulation of carbon and nitrogen turnover. Soil Biol Biochem 36:255–266. https://doi.org/10.1016/j.soilbio.2003.09.012
Halde C, Bamford KC, Entz MH (2015) Crop agronomic performance under a six-year continuous organic no-till system and other tilled and conventionally-managed systems in the northern Great Plains of Canada. Agric Ecosyst Environ 213:121–130. https://doi.org/10.1016/j.agee.2015.07.029
Higashi T, Yunghui M, Komatsuzaki M et al (2014) Tillage and cover crop species affect soil organic carbon in Andosol, Kanto, Japan. Soil Tillage Res 138:64–72. https://doi.org/10.1016/j.still.2013.12.010
Hijmans RJ, Choe H, Perlman J (2016) Spatiotemporal patterns of field crop diversity in the United States, 1870–2012. Agricultural and Environmental Letters 1–6. https://doi.org/10.2134/ael2016.05.0022
Holland JM (2004) The environmental consequences of adopting conservation tillage in Europe: reviewing the evidence. Agric Ecosyst Environ 103:1–25. https://doi.org/10.1016/j.agee.2003.12.018
Howard SA (1947) The soil and health: a study of organic agriculture. University of Kentucky Press
Jacobsen KL, Jordan CF (2009) Effects of restorative agroecosystems on soil characteristics and plant production on a degraded soil in the Georgia Piedmont, USA. Renew Agric Food Syst 24:186–196. https://doi.org/10.1017/S1742170509002592
Jokela W, Posner J, Hedtcke J et al (2011) Midwest cropping system effects on soil properties and on a soil quality index. Agron J 103:1552–1511. https://doi.org/10.2134/agronj2010.0454
Karlen DL (2012) Soil health: the concept, its role, and strategies for monitoring. In: Wall DH, Bardgett RD, Behan-Pelletier V et al (eds) Soil ecology and ecosystem services. Oxford University Press, Oxford, pp 331–336
Chapter Google Scholar
Kauer K, Tein B, de Cima DS et al (2015) Soil carbon dynamics estimation and dependence on farming system in a temperate climate. Soil Tillage Res 154:53–63. https://doi.org/10.1016/j.still.2015.06.010
Kirchmann H, Kätterer T, Bergström L et al (2016) Flaws and criteria for design and evaluation of comparative organic and conventional cropping systems. Field Crop Res 186:99–106. https://doi.org/10.1016/j.fcr.2015.11.006
Krauss M, Ruser R, Müller T, Hansen S, Mäder P, Gattinger A (2017) Impact of reduced tillage on greenhouse gas emissions and soil carbon stocks in an organic grass-clover ley—winter wheat cropping sequence. Agric Ecosyst Environ 239:324–333. https://doi.org/10.1016/j.agee.2017.01.029
Kremen C, Williams NM, Thorp RW (2002) Crop pollination from native bees at risk from agricultural intensification. Proc Natl Acad Sci 99:16812–16816. https://doi.org/10.1073/pnas.262413599
Kuntz M, Berner A, Gattinger A et al (2013) Influence of reduced tillage on earthworm and microbial communities under organic arable farming. Pedobiol Int J Soil Biol 56:251–260. https://doi.org/10.1016/j.pedobi.2013.08.005
Larsen E, Grossman J, Edgell J et al (2014) Soil biological properties, soil losses and corn yield in long-term organic and conventional farming systems. Soil Tillage Res 139:37–45. https://doi.org/10.1016/j.still.2014.02.002
Lawes JB, Gilbert JH (1895) The Rothamsted Experiments. Blackwood, Edinburgh
Lee S-M, Yun H-B, Gu M et al (2014) Effects of hairy vetch, rye, and alternating cultivation of rye-vetch cover crops on soil nutrient concentrations and the production of red pepper (Capsicum annuum L.). J Hortic Sci Biotechnol 89:245–252. https://doi.org/10.1080/14620316.2014.11513075
Lehman RM, Osborne SL, McGraw K (2019) Stacking agricultural management tactics to promote improvements in soil structure and microbial activities. Agronomy 9:539–514. https://doi.org/10.3390/agronomy9090539
Leifeld J, Reiser R, Oberholzer H-R (2009) Consequences of conventional versus organic farming on soil carbon: results from a 27-year field experiment. Agron J 101:1204–1216. https://doi.org/10.2134/agronj2009.0002
Lewis DB, Kaye JP, Jabbour R, Barbercheck ME (2011) Labile carbon and other soil quality indicators in two tillage systems during transition to organic agriculture. Renew Agric Food Syst 26:342–353. https://doi.org/10.1017/S1742170511000147
Li C, He X, Zhu S et al (2009) Crop diversity for yield increase. PLoS One 4:e8049–e8046. https://doi.org/10.1371/journal.pone.0008049
Lotter DW (2003) Organic agriculture. J Sustain Agric 21:59–128. https://doi.org/10.1300/J064v21n04_06
Lotter DW, Seidel R, Liebhardt W (2003) The performance of organic and conventional cropping systems in an extreme climate year. Am J Altern Agric 18:146–154. https://doi.org/10.1079/ajaa200345
Luna JM, Mitchell JP, Shrestha A (2012) Conservation tillage for organic agriculture: evolution toward hybrid systems in the western USA. Renew Agric Food Syst 27:21–30. https://doi.org/10.1017/S1742170511000494
Mader P, Fliessbach A, Dubois D et al (2002) Soil fertility and biodiversity in organic farming. Science 296:1694–1697. https://doi.org/10.1126/science.1071148
Mairura FS, Mugendi DN, Mwanje JI et al (2007) Integrating scientific and farmers’ evaluation of soil quality indicators in Central Kenya. Geoderma 139:134–143. https://doi.org/10.1016/j.geoderma.2007.01.019
Malik RK, Green TH, Brown GF, Mays D (2000) Use of cover crops in short rotation hardwood plantations to control erosion. Biomass Bioenergy 18:479–487. https://doi.org/10.1016/s0961-9534(00)00016-7
Marshall CB, Lynch DH (2018) No-till green manure termination influences soil organic carbon distribution and dynamics. Agron J 110:2098–2099. https://doi.org/10.2134/agronj2018.01.0063
Maryland Department of Agriculture (MDA) (2018) Maryland Cover Crop Program. Available from http://mda.maryland.gov/resource_conservation/pages/cover_crop.aspx . Accessed 20 Jan 2019
Masilionyte L, Maiksteniene S, Kriauciuniene Z et al (2017) Effect of cover crops in smothering weeds and volunteer plants in alternative farming systems. Crop Prot 91:74–81. https://doi.org/10.1016/j.cropro.2016.09.016
McDaniel MD, Tiemann LK, Grandy AS (2014) Does agricultural crop diversity enhance soil microbial biomass and organic matter dynamics? A meta-analysis. Ecol Appl 24:560–570. https://doi.org/10.1890/13-0616.1
Meisinger JJ, Hargrove WL, Mikelsen RL et al (1991) Effects of cover crops on groundwater quality. In: Hargrove WL (ed) Cover crops for clean water. Ankeny, IA, pp 57–68
Metzke M, Potthoff M, Quintern M et al (2007) Effect of reduced tillage systems on earthworm communities in a 6-year organic rotation. Eur J Soil Biol 43:S209–S215. https://doi.org/10.1016/j.ejsobi.2007.08.056
Moebius-Clune BN, Moebius-Clune BK, Gugino BK et al (2016) Comprehensive assessment of soil health—the Cornell framework manual, edition 3.1 edn. Cornell University, Geneva, NY
Monaci E, Polverigiani S, Neri D et al (2016) Effect of contrasting crop rotation systems on soil chemical, biochemical properties and plant root growth in organic farming: first results. Ital J Agron 11:364–374. https://doi.org/10.4081/ija.2017.831
Mondelaers K, Aertsens J, Van Huylenbroeck G (2009) A meta-analysis of the differences in environmental impacts between organic and conventional farming. Br Food J 111:1098–1119. https://doi.org/10.1108/00070700910992925
Moos JH, Schrader S, Paulsen HM (2017) Reduced tillage enhances earthworm abundance and biomass in organic farming: a meta-analysis. Appl Agric For Res 67:123–128. https://doi.org/10.3220/LBF1512114926000
Natural Resources Conservation Services (NRCS) (2012) Soil health. Available from http://www.nrcs.usda.gov/wps/portal/nrcs/main/soils/health/ . Accessed 20 Jan 2019
Nissen TM, Wander MM (2003) Management and soil-quality effects on fertilizer-use efficiency and leaching. Soil Sci Soc Am J 67:1524–1529. https://doi.org/10.2136/sssaj2003.1524
Noble R, Roberts SJ (2004) Eradication of plant pathogens and nematodes during composting: a review. Plant Pathol 53:548–568. https://doi.org/10.1111/j.1365-3059.2004.01059.x
Organic Farming Research Foundation (OFRF) (1998) Final results of the third biennial national organic farmers’ survey. Santa Cruz, CA, USA
Organic Farming Research Foundation (OFRF) (2004) Final results of the fourth national organic farmers’ survey: sustaining organic farms in a changing organic marketplace. Santa Cruz, CA, USA
Otte B, Mirsky S, Schomberg H et al (2019) Effect of cover crop termination timing on pools and fluxes of inorganic nitrogen in no-till corn. Agron J 0:0–11. https://doi.org/10.2134/agronj2018.10.0699
Patel DP, Das A, Kumar M et al (2014) Continuous application of organic amendments enhances soil health, produce quality and system productivity of vegetable-based cropping systems in subtropical eastern Himalayas. Ex Agric 51:85–106. https://doi.org/10.1017/S0014479714000167
Patel DP, Das A, Kumar M et al (2015) Continuous application of organic amendments enhances soil health, produce quality and system productivity of vegetable-based cropping systems in subtropical Eastern Himalayas. Ex Agric 51:85–106. https://doi.org/10.1017/S0014479714000167
Peigné J, Cannavaciuolo M, Gautronneau Y et al (2009) Earthworm populations under different tillage systems in organic farming. Soil Tillage Res 104:207–214. https://doi.org/10.1016/j.still.2009.02.011
Peigné J, Vian J-F, Payet V, Saby NPA (2018) Soil fertility after 10 years of conservation tillage in organic farming. Soil Tillage Res 175:194–204. https://doi.org/10.1016/j.still.2017.09.008
Pfeiffer EE (1943) Biodynamic farming and gardening: soil fertility renewal and preservation, 3rd edn. Anthroposophic Press, New York
Pieters AJ (1927) Green manuring principles and practices. John Wiley & Sons, Inc., New York, NY
Pimentel D, Hepperly P, Hanson J et al (2005) Environmental, energetic, and economic comparisons of organic and conventional farming systems. BioScience 55:573. https://doi.org/10.1641/0006-3568(2005)055[0573:EEAECO]2.0.CO;2
Pittelkow CM, Liang X, Linquist BA, van Groenigen K, Lee J, Lundy ME, van Gestel N, Six J, Venterea RT, van Kessel C (2015) Productivity limits and potentials of the principles of conservation agriculture. Nature 517:365–368. https://doi.org/10.1038/nature13809
Podolsky K, Blackshaw RE, Entz MH (2016) A comparison of reduced tillage implements for organic wheat production in Western Canada. Agron J 108:2003–2012. https://doi.org/10.2134/agronj2016.01.0032
Poeplau C, Don A (2015) Carbon sequestration in agricultural soils via cultivation of cover crops—a meta-analysis. Agric Ecosyst Environ 200:33–41. https://doi.org/10.1016/j.agee.2014.10.024
Poffenbarger HJ, Mirsky SB, Weil RR et al (2015) Legume proportion, poultry litter, and tillage effects on cover crop decomposition. Agron J 107:2083–2014. https://doi.org/10.2134/agronj15.0065
Ponisio LC, M'Gonigle LK, Mace KC et al (2014) Diversification practices reduce organic to conventional yield gap. Proc R Soc B Biol Sci 282:1–7. https://doi.org/10.1098/rspb.2014.1396
Ramesh P, Raten Panwar N, Bahadur Singh A et al (2009) Impact of organic-manure combinations on the productivity and soil quality in different cropping systems in Central India. J Plant Nutr Soil Sci 172:577–585. https://doi.org/10.1002/jpln.200700281
Ranells NN, Wagger MG (1996) Nitrogen release from grass and legume cover crop monocultures and bicultures. Agron J 88:777–882. https://doi.org/10.2134/agronj1996.00021962008800050015x
Reganold JP, Wachter JM (2016) Organic agriculture in the twenty-first century. Nat Plants 2:1–8. https://doi.org/10.1038/nplants.2015.221
Remešová I (2000) The viability of weed seeds in farmyard manure. Rostlinná Výroba 46:515–520
Ryals R, Silver WL (2013) Effects of organic matter amendments on net primary productivity and greenhouse gas emissions in annual grasslands. Ecol Appl 23:46–59. https://doi.org/10.1890/12-0620.1
Sacco D, Moretti B, Monaco S, Grignani C (2015) Six-year transition from conventional to organic farming: effects on crop production and soil quality. Eur J Agron 69:10–20. https://doi.org/10.1016/j.eja.2015.05.002
Säle V, Aguilera P, Laczko E et al (2015) Impact of conservation tillage and organic farming on the diversity of arbuscular mycorrhizal fungi. Soil Biol Biochem 84:38–52. https://doi.org/10.1016/j.soilbio.2015.02.005
Sanchez PA (2002) Soil fertility and hunger in Africa. Science 295:2019–2020
de Cima DS, Luik A, Reintam E (2015) Organic farming and cover crops as an alternative to mineral fertilizers to improve soil physical properties. Int Agrophys 29:137–138. https://doi.org/10.1515/intag-2015-0056
Schulz F, Brock C, Schmidt H et al (2014) Development of soil organic matter stocks under different farm types and tillage systems in the organic arable farming experiment Gladbacherhof. Arch Agron Soil Sci 60:313–326. https://doi.org/10.1080/03650340.2013.794935
Seufert V, Ramankutty N, Foley JA (2012) Comparing the yields of organic and conventional agriculture. Nature 485:229–232. https://doi.org/10.1038/nature11069
Sharma P, Laor Y, Raviv M et al (2017) Compositional characteristics of organic matter and its water-extractable components across a profile of organically managed soil. Geoderma 286:73–82. https://doi.org/10.1016/j.geoderma.2016.10.014
Sharpley A, Jarvie HP, Buda A, May L, Spears B, Kleinman P (2013) Phosphorus legacy: overcoming the effects of past management practices to mitigate future water quality impairment. J Environ Qual 42:1308–1319. https://doi.org/10.2134/jeq2013.03.0098
Silva EM (2014) Screening five fall-sown cover crops for use in organic no-till crop production in the upper Midwest. Agroecol Sustain Food Syst 38:748–763. https://doi.org/10.1080/21683565.2014.901275
Smith AN, Reberg-Horton SC, Place GT et al (2011) Rolled rye mulch for weed suppression in organic no-tillage soybeans. Weed Sci 59:224–231. https://doi.org/10.1614/WS-D-10-00112.1
Smolik JD, Dobbs TL, Rickerl DH (1995) The relative sustainability of alternative, conventional, and reduced-till farming systems. Am J Altern Agric 10:25–35. https://doi.org/10.1017/S0889189300006081
Snapp S, Swinton SM, Labarta R et al (2005) Evaluating cover crops for benefits, costs and performace within cropping system niches. Agron J 97:322–332. https://doi.org/10.2134/agronj2008.0045N
Soil Health Institute (2018) National Soil Health measurements to accelerate agricultural transformation. Available from https://soilhealthinstituteorg/national-soil-health-measurements-accelerate-agricultural-transformation/ . Accessed 20 Jan 2019
Sooby J, Landeck J, Lipson M (2007) National organic research agenda: outcomes from the scientific congress on organic agricultural research. Santa Cruz, CA
Soriano M-A, Álvarez S, Landa BB, Gómez JA (2012) Soil properties in organic olive orchards following different weed management in a rolling landscape of Andalusia, Spain. Renew Agric Food Syst 29:83–91. https://doi.org/10.1017/S1742170512000361
Spargo JT, Cavigelli MA, Mirsky SB et al (2016) Organic supplemental nitrogen sources for field corn production after a hairy vetch cover crop. Agron J 108:1992–1911. https://doi.org/10.2134/agronj2015.0485
Stanhill G (1990) The comparative productivity of organic agriculture. Agric Ecosyst Environ 30:1–26
Suja G, Sundaresan S, John KS, Sreekumar J, Misra RS (2012) Higher yield, profit and soil quality from organic farming of elephant foot yam. Agron Sust Dev 32:755–764. https://doi.org/10.1007/s13593-011-0058-5
Sun H, Koal P, Liu D et al (2016) Soil microbial community and microbial residues respond positively to minimum tillage under organic farming in southern Germany. Appl Soil Ecol 108:16–24. https://doi.org/10.1016/j.apsoil.2016.07.014
Teasdale JR, Cavigelli MA (2010) Subplots facilitate assessment of corn yield losses from weed competition in a long-term systems experiment. Agron Sust Dev 30:445–453. https://doi.org/10.1051/agro/2009048
Teasdale JR, Mirsky SB (2015) Tillage and planting date effects on weed dormancy, emergence, and early growth in organic corn. Weed Sci 63:477–490. https://doi.org/10.1614/ws-d-14-00112.1
Teasdale JR, Mangum RW, Radhakrishnan J, Cavigelli MA (2004) Weed seedbank dynamics in three organic farming crop rotations. Agron J 96:1429–1427. https://doi.org/10.2134/agronj2004.1429
Teasdale JR, Coffman CB, Mangum RW (2007) Potential long-term benefits of no-tillage and organic cropping systems for grain production and soil improvement. Agron J 99:1297–1299. https://doi.org/10.2134/agronj2006.0362
Teasdale JR, Mirsky SB, Spargo JT et al (2012) Reduced-tillage organic corn production in a hairy vetch cover crop. Agron J 104:621–628. https://doi.org/10.2134/agronj2011.0317
Thapa R, Mirsky SB, Tully K (2018a) Cover crops reduce nitrate leaching in agroecosystems: a global meta-analysis. J Environ Qual 47:1400–1411
Thapa R, Poffenbarger H, Tully KL et al (2018b) Biomass production and nitrogen accumulation by hairy vetch–cereal rye mixtures: a meta-analysis. Agron J 110:1197–1208. https://doi.org/10.2134/agronj2017.09.054
Thorup-Kristensen K, Dresbøll DB, Kristensen HL (2012) Crop yield, root growth, and nutrient dynamics in a conventional and three organic cropping systems with different levels of external inputs and N re-cycling through fertility building crops. Eur J Agron 37:66–82. https://doi.org/10.1016/j.eja.2011.11.004
Tittarelli F, Campanelli G, Leteo F et al (2018) Mulch based no-tillage and compost effects on nitrogen fertility in organic melon. Agron J 110:1482–1410. https://doi.org/10.2134/agronj2017.09.0529
Tonitto C, David MB, Drinkwater LE (2006) Replacing bare fallows with cover crops in fertilizer-intensive cropping systems: a meta-analysis of crop yield and N dynamics. Agric Ecosyst Environ 112:58–72. https://doi.org/10.1016/j.agee.2005.07.003
Torstensson G, Aronsson H, Bergstrom L (2006) Nutrient use efficiencies and leaching of organic and conventional cropping systems in Sweden. Agron J 98:603–613. https://doi.org/10.2134/agronj2005.0224
Treadwell DD, Creamer NG, Schultheis JR, Hoyt GD (2008) Cover crop management affects weeds and yield in organically managed sweetpotato systems. Weed Technol 21:1039–1048. https://doi.org/10.1614/wt-07-005.1
Tully K, Ryals R (2017) Nutrient cycling in agroecosystems: balancing food and environmental objectives. Agroecol Sustain Food Syst 41:761–798. https://doi.org/10.1080/21683565.2017.1336149
Tuomisto HL, Hodge ID, Riordan P, Macdonald DW (2012) Does organic farming reduce environmental impacts?—a meta-analysis of European research. J Environ Manag 112:309–320. https://doi.org/10.1016/j.jenvman.2012.08.018
Vakali C, Zaller JG, Köpke U (2011) Reduced tillage effects on soil properties and growth of cereals and associated weeds under organic farming. Soil Tillage Res 111:133–141. https://doi.org/10.1016/j.still.2010.09.003
Wander MM, Traina SJ, Stinner BR, Peters SE (1994) Organic and conventional management effects on biologically active soil organic matter pools. Soil Sci Soc Am J 58:1130–1139. https://doi.org/10.2136/sssaj1994.03615995005800040018x
Wander MM, Yun W, Goldstein WA, Aref S, Khan SA (2007) Organic N and particulate organic matter fractions in organic and conventional farming systems with a history of manure application. Plant Soil 291:311–321. https://doi.org/10.1007/s11104-007-9198-4
Wang Y, Tu C, Cheng L et al (2011) Long-term impact of farming practices on soil organic carbon and nitrogen pools and microbial biomass and activity. Soil Tillage Res 117:8–16. https://doi.org/10.1016/j.still.2011.08.002
Watts DB, Torbert HA, Prior SA, Huluka G (2010) Long-term tillage and poultry litter impacts soil carbon and nitrogen mineralization and fertility. Soil Sci Soc Am J 74:1239–1239. https://doi.org/10.2136/sssaj2008.0415
Welch RY, Behnke GD, Davis AS et al (2016) Using cover crops in headlands of organic grain farms: effects on soil properties, weeds and crop yields. Agric Ecosyst Environ 216:322–332. https://doi.org/10.1016/j.agee.2015.10.014
Welsh C, Tenuta M, Flaten DN et al (2009) High yielding organic crop management decreases plant-available but not recalcitrant soil phosphorus. Agron J 101:1027–1029. https://doi.org/10.2134/agronj2009.0043
Wibberley J (1996) A brief history of rotations, economic considerations and future directions. Asp Appl Biol 47:1–11
Williams A, Hedlund K (2013) Indicators of soil ecosystem services in conventional and organic arable fields along a gradient of landscape heterogeneity in southern Sweden. Appl Soil Ecol 65:1–7. https://doi.org/10.1016/j.apsoil.2012.12.019
Williams DM, Blanco-Canqui H, Francis CA, Galusha TD (2017) Organic farming and soil physical properties: an assessment after 40 years. Agron J 109:600–610. https://doi.org/10.2134/agronj2016.06.0372
Wittwer RA, Dorn B, Jossi W, van der Heijden MGA (2017) Cover crops support ecological intensification of arable cropping systems. Scientific Reports 1–12. https://doi.org/10.1038/srep41911
Wortman SE, Lindquist JL, Haar MJ, Francis CA (2010) Increased weed diversity, density and above-ground biomass in long-term organic crop rotations. Renew Agric Food Syst 25:281–295. https://doi.org/10.1017/S174217051000030X
Wortman SE, Galusha TD, Mason SC, Francis CA (2011) Soil fertility and crop yields in long-term organic and conventional cropping systems in Eastern Nebraska. Renew Agric Food Syst 27:200–216. https://doi.org/10.1017/S1742170511000317
Yagioka A, Komatsuzaki M, Kaneko N (2014) The effect of minimum tillage with weed cover mulching on organic daikon (Raphanus sativus var. longipinnatus cv. Taibyousoufutori) yield and quality and on soil carbon and nitrogen dynamics. Biol Agric Hortic 30:228–242. https://doi.org/10.1080/01448765.2014.922897
Yagioka A, Komatsuzaki M, Kaneko N, Ueno H (2015) Effect of no-tillage with weed cover mulching versus conventional tillage on global warming potential and nitrate leaching. Agric Ecosyst Environ 200:42–53. https://doi.org/10.1016/j.agee.2014.09.011
Zibilske LM, Bradford JM (2007) Soil aggregation, aggregate carbon and nitrogen, and moisture retention induced by conservation tillage. Soil Sci Soc Am J 71:793–710. https://doi.org/10.2136/sssaj2006.0217
Download references
Acknowledgments
We would like to thank Drs. Michel Cavigelli, Andrea Basche, Resham Thapa, and Robert Crystal-Ornelas for their thoughtful reviews of this work.
This research was made possible through a grant awarded to Dr. Kate Tully by the Organic Center ( www.organic-center.org ).
Author information
Authors and affiliations.
Department of Plant Science and Landscape Architecture, University of Maryland, College Park, MD, 20742, USA
Katherine L. Tully & Cullen McAskill
You can also search for this author in PubMed Google Scholar
Corresponding author
Correspondence to Katherine L. Tully .
Ethics declarations
Conflict of interest.
The authors declare that they have no conflict of interest.
Additional information
Publisher’s note.
Springer Nature remains neutral with regard to jurisdictional claims in published maps and institutional affiliations.
Electronic supplementary material
(DOCX 104 kb)
Rights and permissions
Reprints and permissions
About this article
Tully, K.L., McAskill, C. Promoting soil health in organically managed systems: a review. Org. Agr. 10 , 339–358 (2020). https://doi.org/10.1007/s13165-019-00275-1
Download citation
Received : 21 April 2019
Accepted : 28 November 2019
Published : 21 December 2019
Issue Date : September 2020
DOI : https://doi.org/10.1007/s13165-019-00275-1
Share this article
Anyone you share the following link with will be able to read this content:
Sorry, a shareable link is not currently available for this article.
Provided by the Springer Nature SharedIt content-sharing initiative
- Organic farming
- Soil health
- Management practices
- Cover crops
- Crop rotations
- Organic amendments
- Find a journal
- Publish with us
- Track your research

An official website of the United States government
The .gov means it’s official. Federal government websites often end in .gov or .mil. Before sharing sensitive information, make sure you’re on a federal government site.
The site is secure. The https:// ensures that you are connecting to the official website and that any information you provide is encrypted and transmitted securely.
- Publications
- Account settings
Preview improvements coming to the PMC website in October 2024. Learn More or Try it out now .
- Advanced Search
- Journal List
- Elsevier - PMC COVID-19 Collection

Sustainable soil use and management: An interdisciplinary and systematic approach
a School of Environment, Tsinghua University, Beijing 100084, China
Nanthi S. Bolan
b Global Centre for Environmental Remediation, The University of Newcastle, Callaghan, NSW 2308, Australia
Daniel C.W. Tsang
c Department of Civil and Environmental Engineering, The Hong Kong Polytechnic University, Hung Hom, Kowloon, Hong Kong, China
Mary B. Kirkham
d Department of Agronomy, Throckmorton Plant Sciences Center, Kansas State University, Manhattan, KS, United States
David O'Connor
Soil is a key component of Earth's critical zone. It provides essential services for agricultural production, plant growth, animal habitation, biodiversity, carbon sequestration and environmental quality, which are crucial for achieving the United Nations' Sustainable Development Goals (SDGs). However, soil degradation has occurred in many places throughout the world due to factors such as soil pollution, erosion, salinization, and acidification. In order to achieve the SDGs by the target date of 2030, soils may need to be used and managed in a manner that is more sustainable than is currently practiced. Here we show that research in the field of sustainable soil use and management should prioritize the multifunctional value of soil health and address interdisciplinary linkages with major issues such as biodiversity and climate change. As soil is the largest terrestrial carbon pool, as well as a significant contributor of greenhouse gases, much progress can be made toward curtailing the climate crisis by sustainable soil management practices. One identified option is to increase soil organic carbon levels, especially with recalcitrant forms of carbon (e.g., biochar application). In general, soil health is primarily determined by the actions of the farming community. Therefore, information management and knowledge sharing are necessary to improve the sustainable behavior of practitioners and end-users. Scientists and policy makers are important actors in this social learning process, not only to disseminate evidence-based scientific knowledge, but also in generating new knowledge in close collaboration with farmers. While governmental funding for soil data collection has been generally decreasing, newly available 5G telecommunications, big data and machine learning based data collection and analytical tools are maturing. Interdisciplinary studies that incorporate such advances may lead to the formation of innovative sustainable soil use and management strategies that are aimed toward optimizing soil health and achieving the SDGs.
Graphical abstract

- • Soil degradation impedes achieving the United Nations' Sustainable Development Goals.
- • Soil plays a fundamental role for biodiversity conservation.
- • Soil researchers ought to prioritize the multifunctional value of soil health.
- • A framework for interdisciplinary research in soil sustainability is presented.
- • Information management and knowledge sharing may drive sustainable behavior change.
1. Introduction
Soil, commonly viewed as a non-renewable resource due to the extremely slow pace of its regeneration, is under serious threat from modern society ( Amundson et al., 2015 ). Soil degradation occurs due to factors such as water erosion, wind erosion, salinization, and deforestation ( Carlson et al., 2012 ; Celentano et al., 2017 ; Rojas et al., 2016 ). Activities that introduce polluting substances, such as heavy metals, pesticides, polycyclic aromatic hydrocarbons (PAHs), are further causing wide-spread soil degradation. Globally, it is estimated that ~24 billion metric tons of soil are lost through factors such as erosion each year ( UNCCD, 2017 ) and that ~30% of the world's soils are now in a degraded state ( FAO, 2011 ). In China, ~19% of agricultural soil and ~ 16% of all soils exceed national soil quality standards ( MEP, 2014 ). Soil degradation threatens the realization of the United Nations Sustainable Development Goals (SDGs) ( Bouma, 2019 ). To help address soil degradation, the United Nations Food and Agriculture Organization declared 2015–2024 as the International Decade of Soils, aiming to raise public awareness of soil protection. Since then, there has been a burgeoning trend of scientific literature and public debate on soil.
Soil is primarily viewed as a critical component of agricultural production in traditional wisdom. In more recent years, the scientific community has increasingly recognized that soil is also an essential component for environmental protection ( Obrist et al., 2017 ), climate change mitigation ( Le Quere et al., 2018 ), ecosystem services ( Bahram et al., 2018 ), as well as land use and planning ( Gossner et al., 2016 ). There is also a growing recognition that soil health relates not only to the classical biogeophysical processes that are traditionally studied by soil scientists, but also information management, knowledge sharing, and human behavior ( Bampa et al., 2019 ; Bouma et al., 2019 ). Interdisciplinary studies (see Section 2.3 ) are required to understand better the coupling of complex human-nature systems linked to soil management ( Bouma and Montanarella, 2016 ). However, current knowledge on soil processes is scattered across various disciplines, lacking comprehensive views on the sustainable management of soil resources ( Vogel et al., 2018 ).
In 2015, the United Nations General Assembly established 17 goals to be achieved by 2030, which are named the Sustainable Development Goals (SDGs). These include, among others, no poverty, zero hunger, good health and wellbeing, clean water and sanitation and climate action ( UN, 2015 ). The SDGs have become a central theme of global development and international collaboration. Considerable progress has been made in recent years toward reaching the SDGs. For example, the proportion of the global population with access to safe drinking water and the percentage of children receiving vaccinations have both risen considerably. However, many challenges still exist, such as: 821 million people remain undernourished, representing a 5% increase between 2015 and 2017; investment in agriculture from governmental sources and foreign aid has dropped; and, atmospheric concentrations of CO 2 and other greenhouse gases (GHGs) continue to rise ( UN, 2019 ), exacerbating the current climate crisis. Governments from local to national levels need to develop integrated programs addressing these sustainability challenges ( Bryan et al., 2018 ).
In the ongoing actions toward reaching the United Nations SDGs, the soil science community has somewhat underplayed the potential role it could play, partly due to the scattered nature of soil knowledge mentioned above. If researchers from wider disciplines were to collaborate more with soil scientists, it may help progress approaches to achieving the SDGs in a manner more effective than acting alone. Therefore, the profile of the soil science discipline may need to be raised, especially the interdisciplinary components that support food security, climate change mitigation, biodiversity, and public health, in order to better design comprehensive strategies toward realizing the SDGs.
In the present paper, we do not reiterate the importance of the interaction between soil science and agronomy covering crop productivity, which has been discussed in other existing publications ( Sanchez, 2002 ; Tisdale et al., 1985 ). Instead, we focus on the interdisciplinary nature of soil and sustainable soil use and management and linkages with soil science with social science, climate science, ecological science, and environmental science.
2. The interdisciplinary nature of sustainable soil use and management
2.1. sustainable development goals (sdgs).
Soil plays a pivotal role in the United Nations SDGs, most notably SDGs 2, 3, 6, 12, 13, and 15 ( Bouma and Montanarella, 2016 ; Keesstra et al., 2016 ). Most people in poverty live in rural areas where crop production is a vital source of income. In these areas, soil health is a decisive factor for productivity and income levels. Among other roles, soil provides the basis for food production and ecosystem services ( Bender et al., 2016 ; Oliver and Gregory, 2015 ). Moreover, as soil biodiversity is related to lower crop diseases and pests, the ecological services offered by healthy soil systems are important in reducing poverty and ending hunger. Soil also affects water quality, GHG emissions, and other important environmental considerations in regard to the SDGs ( Bharati et al., 2002 ; Franzluebbers, 2005 ). An overview of the identified relationships between soil and the relevant SDGs are illustrated in Fig. 1 .

The relevance of soil to the United Nations' Sustainable Development Goals (SDGs).
It is imperative to disseminate soil science knowledge to policy makers and practitioners who design and implement SDG programs (see Section 3 ). Effective action needs to be taken by the soil science community to help develop suitable indicators that are not only scientifically sound, but also practical for small hold farmers and other stakeholders. Scientific research needs to be specifically directed toward realizing the SDGs, rather than to just understand soil science. The influence of human behavior must be factored into this complex human-nature system. It is also necessary to include the impacts of socio-economic activity on soil health when carrying out sustainability assessments, thus allowing more informed decision making ( Vogel et al., 2018 ).
2.2. The soil health concept
Soils have a wide range of physical, chemical, and biological properties that are attributable to the parent material (e.g., geologic origin and depositional processes), environmental factors (e.g., climate conditions, topography) as well as anthropogenic influences (e.g. farming practice, surface disturbance, pollutant emissions). Because soil plays such a critical role in multiple natural and anthropogenic systems, such soil properties will affect ecosystem services, environmental quality, agricultural sustainability, climate change, and human health. This multi-functional aspect makes traditional soil quality evaluation systems, which have tended to focus on soil fertility and agricultural production ( Doran and Parkin, 1994 ), no longer fully appropriate. Most recently, the “soil health” concept has been the subject of increasing research attention (see Fig. 2 ). This holistic approach accounts for non-linear mechanistic relationships between various physical, chemical, and biological properties. Moreover, the soil health holistic concept is advantageous over traditional soil quality assessments because it considers ecosystem services as well as agricultural production, i.e., both nature and human driven objectives ( Kibblewhite et al., 2008 ).

Number of research articles listed in the Web of Science database ( www.webofknowledge.com ) when soil AND sustainability and “soil health” were searched as topics (searched on 3rd March 2020).
Doran and Zeiss (2000) defined soil health as “the capacity of soil to function as a vital living system, within ecosystem and land-use boundaries, to sustain plant and animal productivity, maintain or enhance water and air quality, and promote plant and animal health” Their definition has been well received by the scientific community, as evidenced by the article being cited ~1500 times according to Google Scholar. The authors argued that soil health is a holistic concept which portrays soil as a living system (i.e., the capacity of soil to function as a living system), while soil quality describes a soil's capacity for a specific use (i.e., fitness for different uses). The outcomes of soil use and management decisions are reflected in soil health ( Doran and Safley, 1997 ).
Assessing soil health involves the selection of indicators, quantification or qualitative scoring, and providing a final index with appropriate weighting and integration ( Rinot et al., 2019 ). Biophysical indicators are particularly relevant for assessing soil health. This is because healthy soil is manifested through a variety of soil functions that are reliant upon biological processes, e.g. carbon transformation, nutrient cycling, maintaining soil structure, and regulating pests and disease ( Kibblewhite et al., 2008 ). Scientists have explored the use of soil microorganisms ( Nielsen et al., 2002 ; Van Bruggen and Semenov, 2000 ), enzyme activities ( Ananbeh et al., 2019 ; Janvier et al., 2007 ), earthworms and nematodes ( Neher, 2001 ), as well as other biological indicators to assess soil health. Similarly, soil structure, compaction and moisture retention have been used as physical indicators of soil health.
2.3. Interdisciplinary research
The sustainability of soil systems is affected by their bio-physico-chemical properties, and the soil use and management decisions made by farmers ( Doran and Zeiss, 2000 ). These two aspects can be broadly categorized into natural and anthropogenic processes. Complex dynamics are involved in the coupled human-nature systems, rendering many challenges for the study of soil systems from any single disciplinary lens. We must develop an interdisciplinary approach to address these challenges ( Totsche et al., 2010 ). It should be noted that interdisciplinary approaches differ from multidisciplinary approaches, in that they integrate insights on a common problem (e.g. climate change) from different disciplines (e.g. soil science and climate science) to construct a comprehensive understanding of the issue. In comparison, multidisciplinary approaches involve gaining separate insights on a common problem from the perspectives of different disciplines ( Repko and Szostak, 2020 ).
As many of the problems surrounding soil sustainability are complex and broad, they cannot be sufficiently addressed by one single discipline, thus interdisciplinary studies are needed ( Klein and Newell, 1997 ). Based on a published framework that interconnected disciplinary lines for another topic ( Hammond and Dubé, 2012 ), here we propose a general framework for developing an interdisciplinary perspective on sustainable soil use and management ( Fig. 3 ). We propose that five broad issues have a root in soil science and are linked to at least one other discipline. The issues themselves are also interconnected. Take management and behavior as an example, which is directly linked to soil science and social science. At the same time, soil fertility and soil pollution are also involved, which are directly linked to agronomy and environmental science, respectively. Another example is soil carbon (or soil organic matter) which is directly linked to both soil science and climate science while also affecting soil biodiversity linked to ecology, and soil fertility linked to agronomy. In a sense, the network shown on Fig. 3 forms a complex six-disciplinary system, which can be used for studying soil sustainability.

A framework for interdisciplinary research in soil sustainability linking soil science with social science, environmental science, ecology, climate science, and agronomy.
3. Soil and social science
3.1. knowledge transfer.
A myriad of scientific knowledge exists regarding best practice for soil management. However, there has been a general lack of adoption by farmers ( Bouma, 2019 ). This can be attributed to obstacles that hinder the distribution of relevant scientific information. Scientific evidence from in-depth studies is often scattered within various disciplines that use technical jargon that is little understood by the social scientists or journalists who are engaged in information transmittal and knowledge sharing. Modern electronic information sharing techniques, including social media tools (e.g., Twitter and Facebook), make mass information distribution easier ( Mills et al., 2019 ), but they can also make it difficult for lay people to distinguish between evidence-based reliable information and inaccurate or even misleading information. A parallel example occurred during the novel coronavirus disease (COVID-19) outbreak, during which large amounts of misinformation were transmitted across social media. Scientists felt the need to publish a joint statement to denounce such rumors ( Calisher et al., 2020 ).
Information management and knowledge sharing may help to fill the gap between knowledge generation and its useful application. This is particularly important for the application of soil science. A variety of soil information management and knowledge sharing mechanisms exist, including training workshops (online or offline), websites, social media, advisory services. In Australia, the New South Wales local government uses webinars to disseminate soil science information to a geographically disperse community of practice (CoP) ( Jenkins et al., 2019 ). Grain advisors, however, were reported to be guiding farmers to historically established “rules of thumb” for calculating nitrogen fertilizer needs, rather than the latest evidence-based science on soil water and nitrogen management ( Schwenke et al., 2019 ). Another Australian local government decided to share soil information and knowledge using a website coupled with training workshops. The type of information shared may include soil properties and landscape characteristics obtained from field assessment studies. Such initiatives show that centralized knowledge sharing can bring significant tangible benefits ( Imhof et al., 2019 ). However, a 10-year follow-up survey showed that while training workshops could be effective in the short term, behavioral change was not sustained in the long term. It was suggested that continuing professional development to upskill farm advisors and the CoP may render a more persistent uptake of knowledge at the farm level ( Andersson and Orgill, 2019 ).
In Europe, both private and public sector advisors, operating on national, provincial or local levels offer science communication to farmers ( Ingram and Mills, 2019 ). In Switzerland, sustainable soil management knowledge was successfully shared among farmers via social learning in a video format ( Fry and Thieme, 2019 ). A study in the English East Midlands suggested that soil advisors ought to incorporate hands-on practical knowledge ( Stoate et al., 2019 ). This concurs with another study in Australia, which showed that establishing a network of senior ex-governmental soil scientists and farmers enabled effective soil knowledge transfer ( Packer et al., 2019 ).
As precision agriculture incentivizes the use of sensing technologies to collect soil data, it becomes increasingly important to form public-private partnerships to collect, store, and use the huge amounts of geographically referenced soil data generated ( Robinson et al., 2019 ). The emerging fifth generation of wireless technology for digital cellular networks (5G), big data, and machine learning offer data collection and analysis techniques that may enable a new generation of soil information sharing tools. Within the 5G system, an internet of things (IoT) can be established with low latency, enabling real time soil measurement and response. For instance, unmanned aerial vehicle (UAV) based remote sensing can be coupled with soil amendment delivery in precision agricultural practice ( Kota and Giambene, 2019 ; Morais et al., 2019 ). Big data applications with machine learning also provide predictive power, facilitating smart farming to save energy, water, and cost, while increasing crop yields ( Wolfert et al., 2017 ).
3.2. Farmer behavior
The sustainability of soil use and management is ultimately reliant on the real-world behavior by practitioners, most particularly farmers. Therefore, there is a growing interest to integrate social components and farmer behavior with the ecological component of soil management ( Amin et al., 2019 ). In modern society, with the fast-growing use of various types of information technology, farmer behavior can be influenced by different network-based approaches. For instance, a study in Europe found that farmers formed a learning network by sharing information and soil knowledge on the microblogging and social networking service, Twitter. This platform has a limited length for each message (280 characters for non-Asian languages), making it easy for time-constrained farmers to follow ( Mills et al., 2019 ). In the US, an integrated network-based approach enabled a quarter of respondents to adopt cover crops for weed control, and respondents also increased their follow-up usage from information shared on Twitter (22%), YouTube (23%), and web sites (21%) ( Wick et al., 2019 ).
Farmer behavior and farming practice is also directly affected by professional advisors. In Australia, farmers apply the recommendations of professional crop advisors to select suitable fertilizer dosages. However, attitudes concerning financial risk, soil heterogeneity, and local climate conditions can affect their perception and adoption of such advice ( Schwenke et al., 2019 ). In Europe, a knowledge gap regarding sustainable soil management was identified as a major issue among both farmers and soil advisors. As the current trend of privatization and decentralization of advisory services continues, there is an increasing need to educate those who provide advisory services, thus enabling effective empowerment of farmers ( Ingram and Mills, 2019 ). Governments ought also to provide workshops that encourage farmers to adopt greater soil testing, so that they can then make informed soil management decisions ( Lobry de Bruyn, 2019 ).
Lack of education and awareness creates an obstacle for sustainable soil use and management, especially in developing countries. For example, it was found that farmer perception strongly correlates to adoption rates for conservation agriculture (r = 0.81; p < 0.05) ( Mugandani and Mafongoya, 2019 ). It has been reported that concerns over soil type, weed control, and weather conditions were the main inhibiting factors when English farmers consider reduced tillage practice. The authors suggested that enhanced adoption of sustainable soil management practice will require improved communication between the soil research community and farmers ( Alskaf et al., 2020 ).
3.3. Stakeholders
The creation, dissemination and usage of soil sustainability knowledge involves a wide range of stakeholders, such as scientists, farmers, land managers, advisory services, commercial product suppliers, regulators, funding agencies, educators, students, as well as the general public ( Knox et al., 2019 ; Tulau et al., 2019 ). Different stakeholders will have different concerns. Farmers and crop advisors are primarily concerned about local soil knowledge, while regulators and scientists are more concerned about policy, scientific solutions and the wider environment ( Bampa et al., 2019 ). There is also a dynamic interaction and potential gap between awareness and perception, i.e., what can be done and what is worth doing ( Krzywoszynska, 2019 ). Based on an analysis in England, Krzywoszynska (2019) argued that interactions between soil researchers and end users are multifaceted and that these actors must work together on both knowledge generation and knowledge sharing to enhance sustainable behavior.
Scientists and governments are pivotal stakeholders in promoting sustainable soil use and management practices. Their action can enhance the robustness of scientific knowledge creation and broaden its applicability by incorporating evidence into policy instruments. In Scotland, soil risk maps are created by scientists, policy makers and industrial representatives working in close collaboration ( Baggaley et al., 2020 ). Similarly, in Australia, soil constraints maps have been produced for site-specific management ( van Gool, 2016 ). Such tools can help mitigate constraints to achieving climate-driven genetic yield potential of agricultural crops. Models that incorporate learnings from stakeholder engagement can also render strong predictive power ( Inam et al., 2017 ). Traditionally, the main channel of soil knowledge generation has been government funded. However, there has been a general decreasing trend in the provision of government funds for soil data collection in many developed countries, while privately funded collection of soil information has increased dramatically ( Robinson et al., 2019 ). Under this situation, it is even more important to bring in additional stakeholders to create and share soil knowledge. The Soil Knowledge Network (SKN) in Australia demonstrated that ex-governmental soil scientists can exert long-lasting positive impacts by coaching new generations of early career soil scientists ( McInnes-Clarke et al., 2019 ).
4. Soil and climate science
4.1. soil organic carbon.
Soil organic carbon (SOC) has been recognized as a critical indicator of soil health, because it reflects the level of soil functionality associated with soil structure, hydraulic properties, and microbial activity, thereby integrating physical, chemical and biological health of soil ( Vogel et al., 2018 ). Recently, increasing attention has been placed on SOC beyond the traditional sphere of soil science. This is because it is a key component of Earth's carbon cycle, thus having huge implications for the current climate crisis ( Kell, 2012 ) and SDG13: Climate action. Soil is the largest terrestrial carbon pool, holding an estimated 1500–2400 GtC and permafrost (i.e. frozen soil) storing 1700 GtC ( Le Quere et al., 2018 ). A global initiative known as ‘4 per 1000’, which aims to increase soil organic carbon by 0.4% per year, would result in an additional carbon storage of 1.2 GtC per year if successful ( Paustian et al., 2016 ; Rumpel et al., 2018 ). In Australia, surface soils provide a significant reservoir of carbon, holding ~19 billion metric tons. However, most of these soils (~75%) contain <1% SOC, suggesting huge additional capacity for carbon sequestration. An annual 0.8% increase in carbon storage across all Australian surface soils would fully offset the nation's GHG emissions ( Baldock et al., 2010 )
Soil properties and vegetation are affected by the climatic condition ( Bond-Lamberty et al., 2018 ). For example, global warming may accelerate soil erosion due to its impact on microorganisms and plant and animal species ( Garcia-Pichel et al., 2013 ). Moreover, different soil types and land use systems are unevenly sensitive to temperature changes. Soil carbon that is normally recalcitrant in semi-arid regions is vulnerable to rising temperature ( Maia et al., 2019 ). Therefore, soil management practice in these areas may have a tremendous effect on carbon cycling.
Organic fertilizer applications can improve soil functionality and significantly increase SOC levels. Thus, applying organic amendments, including biosolids and composts, to agricultural land can increase carbon storage and contribute significantly to offsetting GHG emissions. Studies have shown that manure can potentially increase crop yields and soil organic contents in comparison with mineral fertilizers ( Jing et al., 2019 ). A 37-year field study showed that organic fertilization increased soil carbon input by 25% to 80%, although levels of carbon retention ranged from only 1.6% for green manure to 13.7% for fresh cattle manure ( Maltas et al., 2018 ). Similarly, Bolan et al. (2013) demonstrated that biosolid applications likely result in higher levels of carbon sequestration compared to other management strategies including fertilizer application and conservation tillage. This was attributed to an increased microbial biomass, and Fe and Al oxide-induced immobilization of carbon ( Bolan et al., 2013 ). In comparison with open-air systems, the use of organic fertilizers for indoor greenhouse soils may have a greater positive influence on soil functionality due to its effect on porosity and pore connectivity ( Xu et al., 2019 ). It should be noted that organic fertilizers may not increase crops yields to the levels achievable with inorganic fertilizers. This issue can be overcome by supplementing organic fertilizers with inorganic ones ( Maltas et al., 2018 ).
A variety of conservation farming practices can increase SOC levels, while also increasing crop productivity and decreasing water demand ( Kumar et al., 2019 ; Mehra et al., 2018 ). Crop residue return to surface soils can have a positive effect on soil carbon sequestration ( Chowdhury et al., 2015 ; Li et al., 2019b ). For example, chopping and returning wheat straw and corn stover can increase SOC levels by 14.5% in a double-cropping system ( Zhao et al., 2019 ). Reduced tillage and non-tillage practices can also increase soil SOC levels ( Chatskikh et al., 2008 ; Lafond et al., 2011 ). For example, a 22-year study showed that with no tillage, mulch treatment had a significantly positive effect on carbon retention ( Kahlon et al., 2013 ). Integrated methods have the potential to achieve even more significant increases in SOC levels. For example, SOC data collected over 35 years in a semi-arid region of China showed that carbon levels were enhanced (by 453% to 757%) using a combination of best practice cultivation, mulching, and planting methods ( Guoju et al., 2020 ). Different land uses also affect SOC, not only in terms of concentration, but also the fractions of SOC that are vulnerable to mineralization ( Ramesh et al., 2019 ). For example, labile and humified SOC fractions have been reported to be more prone to mineralization in arable lands than in grasslands ( Ukalska-Jaruga et al., 2019 ).
Accurate quantification of SOC remains a challenge because of high spatial heterogeneity in soils. For instance, features such as hedgerows and fences can influence SOC due to their impact on soil moisture and bulk density ( Ford et al., 2019 ). Soil compaction by agricultural machinery reduces macropores and creates water ponding ( Mossadeghi-Björklund et al., 2019 ), which can affect SOC. There are also discrepancies between SOC estimates using regional versus local parameters, particularly for in woodland soils containing large amounts of decaying organic matter (e.g., Histosols) and low-input high-diversity ecosystems ( Ottoy et al., 2019 ).
4.2. Biochar as a mitigation
Biochar is a carbon rich product that is produced by the burning of biomass with a limited supply of oxygen (i.e., pyrolysis) ( Lehmann and Joseph, 2009 ; Wang et al., 2020c ). It typically possess a stable fixed carbon structure with high porosity, a high specific surface area and a high alkalinity. These characteristics enable biochar to enhance soil moisture content, sorb polluting substances and increase soil pH ( Andrés et al., 2019 ). Moreover, biochar is considered carbon negative because the carbon within its structure, which is captured from the atmosphere during biomass formation, is more recalcitrant in the natural environment than carbon in biomass that has not been pyrolized. Because of its carbon negativity and beneficial properties for soil management, biochar has been proposed as a possible technology to help mitigate climate change ( Woolf et al., 2010 ). Numerous studies have explored the usage of biochar in croplands ( Laird et al., 2010b ), while recent studies have also examined its application in other systems, such as alpine grassland ( Rafiq et al., 2019 ).
At the current carbon price, applying biochar to soil is not commercially viable unless there is an additional benefit to farmers. Therefore, researchers have conducted extensive research on the benefits biochar for agricultural and environmental purposes. One of the most researched areas is the use of biochar to increase crop yields. A recent meta-analysis found that in comparison with inorganic fertilizer alone, biochar can increase crop yields by 11% to 19% (95% confidence intervals) ( Ye et al., 2020 ). Biochar has also been put forward as a sustainable technique for remediating soils degraded by contaminants, especially heavy metals ( Hou, 2020 ; O'Connor et al., 2018c ; Song et al., 2019 ). The sustainability of biochar is increased if the biomass feedstock is a biological waste that would otherwise be burned or discarded at landfill, thus avoiding air pollution or the consumption of landfill space. However, while a myriad of studies have shown biochar applications have positive effects on soil, it should be noted that such effects may diminish after 3– 5 years ( Dong et al., 2019 ). Biochar effectiveness and longevity may be enhanced by the invention of engineered biochars ( O'Connor et al., 2018b ).
4.3. Soil greenhouse gases
Soils act as significant sources of various greenhouse gases (GHGs), including CO 2 , CH 4 , and N 2 O. Reducing the emission of such GHGs is one of the greatest challenges for sustainable farming ( de Araújo Santos et al., 2019 ) and the achievement of SDG13: Climate action. Soil CO 2 emissions are affected by agricultural practice (e.g. tillage and fertilizer application), as well as the soil properties (e.g. soil texture). For sandy soils, greater macroporosity tends to be associated with higher CO 2 emissions, while microporosity is associated with lower emissions, which likely related to their respective tortuosity levels ( Farhate et al., 2019 ; Tavares et al., 2015 ). The use of lime to treat low pH soils may also relate to CO 2 emissions. Therefore, sustainable management of low pH grasslands may involve the use of low liming dosage rates, which provide almost the same result as higher rates ( Bolan et al., 2003 ; Kunhikrishnan et al., 2016 ; Lochon et al., 2019 ). A study in Denmark showed that reduced tillage practice can decrease net GHG emissions by 0.56 Mg CO 2 -eq. ha −1 per year; moreover, the use of disc coulters that minimally disturb soil can reduce net GHG emissions by 1.84 Mg CO 2 -eq. ha −1 per year ( Chatskikh et al., 2008 ).
Atmospheric N 2 O accounts for ~6% of radiative forcing caused by anthropogenic activity, which largely stems from soil systems ( Davidson, 2009 ). Therefore, emission of N 2 O from agricultural soil is particularly concerning. Davidson (2009) estimated that 2% of nitrogen in manures and 2.5% of nitrogen in fertilizers used by farmers over the period of 1860–2005 was converted to atmospheric N 2 O. In China, emissions derived from synthetic nitrogen fertilizers account for ~7% of the nation's annual GHG budget. By implementing new technology and best management practices that minimize nitrogen use in soil management, it is feasible to reduce GHG emissions by 102–357 Tg CO 2 -equivalent in China alone ( Zhang et al., 2013 ). Soil amendment with more sustainable alternatives to synthetic nitrogen (e.g., biochar) may help reduce N 2 O emissions from soil ( Senbayram et al., 2019 ).
Methane emissions from soil represent another major factor for climate change. An early study found that the application of rice straw to paddy fields increased CH 4 emissions by a factor of 1.8 to 3.5 ( Yagi and Minami, 1990 ). Recently, methane emissions from permafrost (permanently frozen soil) has drawn attention from the climate science community, owing to its critical role in carbon cycling ( Schuur et al., 2015 ). As climate change occurs, rising temperature in the polar regions causes permafrost to thaw and microbial activity to increase ( Hollesen et al., 2015 ). This leads to increased methane and CO 2 emissions from organic-rich Arctic soils ( Schuur et al., 2013 ). As these gases are associated with increased global warming potential, their emission increases the levels of permafrost thaw, thus forming a positive feedback loop. It is imperative to understand these processes in a quantitative way. As the climate change crisis worsens, it may be necessary to take mitigating measures involving soil management in areas associated with high methane fluxes.
5. Soil biodiversity and ecology
5.1. soil biodiversity.
Sustainable soil management practice can improve or conserve soil biodiversity, which represent a significant proportion of Earth's total biodiversity ( Bahram et al., 2018 ) and is pertinent to the achievement of the United Nations' SDGs (e.g., SDG15: Life on land). Among other factors, soil microbial communities are affected by the availability of nutrients corresponding to the type of soil management practice ( Bolan et al., 1996 ; Lauber et al., 2009 ; Leff et al., 2015 ). For example, the use of soluble fertilizers (e.g., monocalcium phosphate), less soluble organic fertilizer (e.g., sugarcane filter cake) or nearly insoluble rock phosphate ( Arruda et al., 2019 ) have different impacts on soil microbial communities. Soil management practices also affect soil hydraulics, which affects plant and microbial biodiversity and ecosystem resilience ( Alley et al., 2002 ; Anderegg et al., 2018 ). A study in India reported that integrating crop residue return with green manure application and no-tillage in a rice-wheat double cropping system increased SOC levels by 13%, the microbial biomass by 38%, the basal soil respiration rate by 33%, and the microbial quotient by 30% ( Saikia et al., 2020 ). Certain soil amendments are associated with increased soil biodiversity. For example, biochar amendment of a Mediterranean vineyard soil decreased the mineralization of both SOC and microbial biomass, while the functional microbial diversity and biodiversity of soil micro-arthropods were maintained ( Andrés et al., 2019 ). Soil properties and biodiversity are also affected by plant root systems within the rhizosphere ( Dey et al., 2012 ).
Larger species in soil are also an important aspect of soil biodiversity as well as being influential on soil properties ( Bardgett and van der Putten, 2014 ; Wu et al., 2011 ). Earthworms (Oligochaeta) are a particularly important soil species due to their creation of soil macro-pores (>0.3 mm) and channels (burrows) that increase water and gas infiltration rates ( Bartz et al., 2013 ; Bhadauria and Saxena, 2010 ). Thus earthworm activity can render soil environments that are more amenable to microbial activity and diversity ( Eriksen-Hamel et al., 2009 ). Conservation tillage practices that involve crop residue return to surface soils can increase earthworm numbers by hundreds of thousands per hectare ( Barthod et al., 2018 ; Giannitsopoulos et al., 2020 )
5.2. Ecosystem services
Soils provide vital ecosystem services, rendering both economic and societal benefits ( Adhikari and Hartemink, 2016 ; Dominati et al., 2010 ; Pavan and Ometto, 2018 ; Su et al., 2018 ). Monetary valuation methods have been put forward to account for the natural capital of this resource ( Robinson et al., 2014 ). In this way, a national-scale study in the UK suggested that an additional £18 billion GBP of ecosystem services could be achieved under an optimal policy scenario. This value takes into account major ecosystem services, such as agricultural production, carbon sequestration, recreational usage, and wildlife diversity ( Bateman et al., 2013 ). However, some scholars have argued that systematic monetarization is unnecessary. For example, Bayesian Belief Networks (BBNs) and Multi-Criteria Decision Analysis (MCDA) methods can provide decision makers with semi-quantitative information that takes into account the multifunctionality of soil ecosystem services ( Baveye et al., 2016 ).
Living organisms in soil have a direct impact on agricultural productivity and ecosystem services. For instance, the microbial community is essential for the natural decontamination of polluted soils. Therefore, monitoring biological indicators is necessary for managing soil ecosystems effectively. Some of the most important soil biota indicators include microsymbionts, decomposers, elemental transformers, soil ecosystem engineers, soil-borne pests and diseases, and microregulators ( Barrios, 2007 ). Soil invertebrates also play a significant role in soil ecosystem services ( Lavelle et al., 2006 ).
In Europe, a large number of monitoring programs and field studies have been conducted since the 1990s, to gain data for optimizing ecosystem services ( Pulleman et al., 2012 ). The data shows that spatial heterogeneity within soil systems translates into the uneven distribution of ecosystem services ( Aitkenhead and Coull, 2019 ). Governments may intervene to restore or improve ecological services in limited soil systems. In China, for example, the government has made subsidies available to farmers to protect natural woodlands and convert steep agricultural cropland into other land uses, such as grassland or woodland ( Liu et al., 2008 ). If farmland is degraded to an extent that it is abandoned, soil treatments may help bring about natural revegetation and the recovery of ecosystem services ( Li et al., 2019a ). For example, the recovery of severely degraded land can be facilitated by the use of soil amendments such as biochar ( O'Connor et al., 2018c ).
6. Soil and environmental science
6.1. soil pollution.
Contaminants are an issue for many agricultural sites ( Bolan et al., 2014 ; Khan, 2016 ; O'Connor et al., 2019b ; Wilcke, 2000 ), which hinders efforts toward the achievement of the United Nations' SDGs (e.g., SDG3: Good health and well-being). Soil contaminants include heavy metals, such as cadmium (Cd), copper (Cu), lead (Pb), mercury (Hg) and zinc (Zn), and organic pollutants, such as pesticides and polycyclic aromatic hydrocarbons (PAHs). As an emerging contaminant, microplastics in the soil environment have also drawn attention in recent years ( Bradney et al., 2019 ; Jia et al., 2020 ; O'Connor et al., 2020 ; Wang et al., 2020a ). Assessment of their fate and transport is critical for understanding the environmental risk ( Corradini et al., 2019 ; Wang et al., 2019a ).
A global map of soil pollution is urgently needed to understand better the situation globally, but few countries are investing in national-scale investigations ( Hou and Ok, 2019 ). Elevated levels of soil pollutants can result from a wide variety of anthropogenic activities, ranging from metal mining to fossil fuel burning ( Zhang et al., 2020b ). The spatial redistribution of these pollutants involves inter-phase transfer such as dissolution from soil to water, volatilization from soil to air, and deposition from air to soil ( O'Connor et al., 2019a ; Zhang et al., 2019 ). Anthropogenic soil pollution in under-developed regions where industrial activities are less intensive can also occur due to traffic and mining related emissions, etc. For instance, a recent study in a suburban area of Central Asia showed that Pb, Zn, and Cu can accumulate to high levels in soils because of road traffic up to 200 m away ( Ma et al., 2019 ).
The remediation of contaminated soil is an important research field interlinking soil science and environmental science. Traditionally, remediation practitioners focused on either physical cleanup methods, such as soil excavation and disposal at landfill ( Qi et al., 2020 ), or chemical treatment methods, such as in situ chemical oxidation ( O'Connor et al., 2018a ). In recent years, nature-based solutions, such as phytoremediation and green stabilization, have gained attention among the scientific research community ( Wang et al., 2019b ; Wang et al., 2020b ; Zhang et al., 2020a ). For example, microbial strains from unique natural environments are being harvested, cultured, and exploited to render economic and environmentally friendly solutions for soil decontamination ( Atashgahi et al., 2018 ; Bunge et al., 2003 ).
6.2. Soil erosion
Soil erosion, a major land degradation process, is caused by the weathering effects of water and wind ( Lal, 2003 ). For land covered by native vegetation, natural erosion rates will tend to balance with soil production rates. However, typical agricultural tillage practice can disrupt this balance, causing levels of soil erosion to be one to two orders of magnitude higher than that of soil formation ( Montgomery, 2007b ). Soil systems that experience net soil erosion can suffer the loss of fertile surface soils, removal of soil organic carbon, and reduced agricultural productivity, thus rendering a high environmental and economic cost globally ( Montgomery, 2007a ; Pimentel et al., 1995 ). Because heavy metals tend to bind strongly to eroded soil particles, the widespread distribution of soil pollutants is also often associated with soil erosion ( Xiao et al., 2019 ).
Soil erosion not only causes damage to the land where it occurs, but also jeopardizes local aquatic systems due to excessive sediment loading ( Boardman et al., 2019 ). Soil erosion models have been developed to predict impacts of water quality on a catchment-scale ( Fu et al., 2019 ). It can also cause damage to nearby housing due to increased surface runoff and landslides. Because of such impacts, many governments are taking largescale mitigating action, such as revegetation with native species and woodland restoration ( Teng et al., 2019 ).
6.3. Soil leaching
During heavy rainfall, irrigation, or recharge events, large volumes of water may come into contact with various substances as soil pore spaces fill ( O'Connor and Hou, 2019 ). In this process, there are complex interactions between gaseous, liquid, and solid phases for soil nutrients, potentially toxic elements, and organic pollutants. If soil nutrients or contaminants are leached from surface soils, they can transport into the subsurface via the vertical migration of infiltration water. This can lead to large scale groundwater pollution involving substances such as ammonia ( Jia et al., 2019 ). Leached nutrients in surface runoff may also enter nearby surface water bodies, causing eutrophication ( Maguire and Sims, 2002 ). Soil leaching may be particularly prominent in the autumn-winter season due to reduced plant activity ( Welten et al., 2019 ).
Soil leaching potential is exacerbated by common physical farming practices, including the installation of deep drainage ( Nachimuthu et al., 2019 ). The potential for soil leaching is also affected by soil management practices that alter the chemical composition of soil. For instance, liming is a common farming method to increase soil pH and reduce flocculation. However, recent studies have suggested that soil particle surfaces become more negatively charged as soil pH increases. Therefore, liming activity may lead to soil-bound harmful substances, such as perfluorooctane sulfonic acid (PFOS) and perfluorooctanoic acid (PFOA), leaching from soil and entering groundwater systems ( Oliver et al., 2019 ). In New Zealand, intensified agricultural production on steep landscapes, which is encouraged by the government's policy to significantly increase agricultural exports, has involved the replacement of perennial pastures with winter forage crops. This has increased the use agrochemicals, including glyphosate and diazinon, which not only pose an environmental risk in themselves, but also facilitate the leaching of organic carbon and nitrogen ( Chibuike et al., 2019 ). The reporting of such unintended consequences reinforces the importance of comprehensive assessments for sustainable soil use and management. It should be noted that certain soil amendments, such as biochar, have been shown to reduce soil nutrient leaching potential ( Laird et al., 2010a ).
Soil leaching can increase the spatial heterogeneity of soil nutrients, which makes soil management more difficult. For instance, intensively farmed cropland tends to be subject to high nitrogen input levels. However, plant-animal-soil systems are not efficient in utilizing large amounts of nitrogen, with only 15–35% being embedded in agricultural products. A large percentage of the surplus nitrogen is returned to localized spots via animal urinary excretions, resulting in elevated nitrogen hotspots.
7. Summary, challenges and future directions
The international community's commitment to achieving the United Nations' Sustainable Development Goals (SDGs) hinge on soil health. However, neither the scientific community nor policy makers have paid sufficient attention to soil in their SDG efforts. Soil scientists have not been adequately involved in the discussion on SDG targets and indicators ( Bouma et al., 2019 ). Consequently, while there are four SDG targets that specifically mention soil, and others that indirectly relate to soil, only one explicit soil indicator has been established ( Bouma et al., 2019 ). The lack of involvement by soil scientists may be due to their strong focus on pure soil science, rather than conducting cross-disciplinary and elaborate discussions on big picture soil related issues with other stakeholders. To help provide effective SDG solutions, it is imperative to encourage interdisciplinary soil research among soil scientists and researchers in fields relating to social science, climate science, ecology, and environmental science. When national and local governments form policies according to the United Nations SDGs, soil scientists need to be encouraged to play a more active role, and their advice needs to be sought by decision makers. For instance, by nominating soil scientists to key steering committees.
A big challenge for sustainable soil use and management is the inherent spatial heterogeneity of soil properties, from the micro to the global scale. This makes it difficult to predict non-linear relationships among various soil processes and system behaviors ( Manzoni and Porporato, 2009 ). For example, regional estimates of soil organic carbon stocks have differed by as much as 60% on different scales due to this heterogeneity ( Illiger et al., 2019 ). There is little known about the vertical distribution of organic carbon in the subsurface ( Balesdent et al., 2018 ). As large amounts of carbon are stored in deep soils ( Yu et al., 2019 ), it is essential to understand the status, as well as the mechanisms, of soil carbon cycling across the full extent of the lithosphere.
Spatial heterogeneity also exists in socioeconomic systems. Consider for example the size of typical farm holdings among different countries. In rural China, most farms are smallholdings of <0.5 ha. In Hungary, most farms are also relatively small, with 79% being <2 ha. In contrast, Danish farms tend to quite large, with 55% being larger than 20 ha ( Ingram and Mills, 2019 ). Such differences create challenges for knowledge transfer between countries. For instance, farm size may act as a barrier to the adoption of sustainable farming technology because of financial or technical constraints ( Alskaf et al., 2020 ).
It is important to describe long-term temporal trends in soil system behavior because many prominent issues, such as the climate crisis, require perceptive solutions based on long-term evidence. However, many existing studies, especially studies on emerging issues, are based on short-term findings. For instance, a recent pasture-system study suggested that various species could be planted to control nitrogen leaching associated with cow urine ( Welten et al., 2019 ). This promising finding, however, was based on less than one year of data. Longer-term studies are necessary to verify the effectiveness of such strategies. Greater efforts should be paid on the research and development of accelerated aging techniques ( Shen et al., 2019 )
Progress in sustainable soil use and management relies upon the development of suitable and holistic indicators for soil health that reflect the diverse processes involved, in a concise, quantifiable, reliable and meaningful way. To achieve this goal, soil health needs to be evaluated under site-specific conditions that account for the different processes of different geological, climatic, and societal conditions ( Vogel et al., 2018 ). This would be particularly valuable for aiding farmers with decision making and translating soil science into practical sustainable soil use and management practice. Moreover, to support policy making processes, it is necessary to map soil properties on a regional scale, or even on national and global scales. High resolution mapping and clustering of soil properties would enable targeted recommendations for sustainable soil management ( Donoghue et al., 2019 ). It should also be noted that while many existing soil sustainability studies have focused on the impacts of socioeconomic activities (i.e. soil management) on soil systems (i.e. soil health), studies regarding the impacts of soil systems on socioeconomic systems are less common ( Vogel et al., 2018 ).
Information management and knowledge sharing are critical for building collaborative governance and delivering sustainable solutions ( Bodin, 2017 ). In this new era of information, massive amounts of valuable information (and misinformation) are produced. This poses a challenge to both the knowledge creators, who struggle to make it visible in an ocean of information, and the knowledge users, who struggle to distinguish whether information is valuable or not. Emerging and advanced technologies, such as 5G, big data and machine learning present great opportunities for addressing these challenges. Interdisciplinary studies initiated by, or in collaboration with, communication engineers and computer scientists hold much potential in advancing our capability in sustainable use and management of soil resources.
Declaration of competing interest
The authors declare that they have no known competing financial interests or personal relationships that could have appeared to influence the work reported in this paper.
Acknowledgements
This work was supported by the National Key Research and Development Program of China (Grant No. 2018YFC1801300).
Editor: Jay Gan
- Adhikari K., Hartemink A.E. Linking soils to ecosystem services—a global review. Geoderma. 2016; 262 :101–111. [ Google Scholar ]
- Aitkenhead M.J., Coull M.C. Digital mapping of soil ecosystem services in Scotland using neural networks and relationship modelling. Part 2: mapping of soil ecosystem services. Soil Use Manag. 2019; 35 :217–231. [ Google Scholar ]
- Alley W.M., Healy R.W., LaBaugh J.W., Reilly T.E. Hydrology - flow and storage in groundwater systems. Science. 2002; 296 :1985–1990. [ PubMed ] [ Google Scholar ]
- Alskaf K., Sparkes D.L., Mooney S.J., Sjögersten S., Wilson P. The uptake of different tillage practices in England. Soil Use Manag. 2020; 36 :27–44. [ Google Scholar ]
- Amin M.N., Hossain M.S., Lobry de Bruyn L., Wilson B. A systematic review of soil carbon management in Australia and the need for a social-ecological systems framework. Sci. Total Environ. 2019:135182. [ PubMed ] [ Google Scholar ]
- Amundson R., Berhe A.A., Hopmans J.W., Olson C., Sztein A.E., Sparks D.L. Soil and human security in the 21st century. Science. 2015; 348 [ PubMed ] [ Google Scholar ]
- Ananbeh H., Stojanović M., Pompeiano A., Voběrková S., Trasar-Cepeda C. Use of soil enzyme activities to assess the recovery of soil functions in abandoned coppice forest systems. Sci. Total Environ. 2019; 694 [ PubMed ] [ Google Scholar ]
- Anderegg W.R.L., Konings A.G., Trugman A.T., Yu K., Bowling D.R., Gabbitas R. Hydraulic diversity of forests regulates ecosystem resilience during drought. Nature. 2018; 561 :538. [ PubMed ] [ Google Scholar ]
- Andersson K.O., Orgill S.E. Soil extension needs to be a continuum of learning; soil workshop reflections 10 years on. Soil Use Manag. 2019; 35 :117–127. [ Google Scholar ]
- Andrés P., Rosell-Melé A., Colomer-Ventura F., Denef K., Cotrufo M.F., Riba M. Belowground biota responses to maize biochar addition to the soil of a Mediterranean vineyard. Sci. Total Environ. 2019; 660 :1522–1532. [ PubMed ] [ Google Scholar ]
- de Araújo Santos G.A., Moitinho M.R., de Oliveira Silva B., Xavier C.V., Teixeira D.D.B., Corá J.E. Effects of long-term no-tillage systems with different succession cropping strategies on the variation of soil CO2 emission. Sci. Total Environ. 2019; 686 :413–424. [ PubMed ] [ Google Scholar ]
- Arruda B., Rodrigues M., Gumiere T., Richardson A.E., Andreote F.D., Soltangheisi A. The impact of sugarcane filter cake on the availability of P in the rhizosphere and associated microbial community structure. Soil Use Manag. 2019; 35 :334–345. [ Google Scholar ]
- Atashgahi S., Sanchez-Andrea I., Heipieper H.J., van der Meer J.R., Stams A.J.M., Smidt H. Prospects for harnessing biocide resistance for bioremediation and detoxification. Science. 2018; 360 :743–746. [ PubMed ] [ Google Scholar ]
- Baggaley N., Lilly A., Blackstock K., Dobbie K., Carson A., Leith F. Soil risk maps–interpreting soils data for policy makers, agencies and industry. Soil Use Manag. 2020; 36 :19–26. [ Google Scholar ]
- Bahram M., Hildebrand F., Forslund S.K., Anderson J.L., Soudzilovskaia N.A., Bodegom P.M. Structure and function of the global topsoil microbiome. Nature. 2018; 560 :233. [ PubMed ] [ Google Scholar ]
- Baldock J., Sanderman J., Farquharson R. Proceedings of the 19th World Congress of Soil Science. 2010. Capturing carbon in Australian soils: Potential and realities; pp. 1–6. [ Google Scholar ]
- Balesdent J., Basile-Doelsch I., Chadoeuf J., Cornu S., Derrien D., Fekiacova Z. Atmosphere-soil carbon transfer as a function of soil depth. Nature. 2018; 559 :599. [ PubMed ] [ Google Scholar ]
- Bampa F., O’Sullivan L., Madena K., Sandén T., Spiegel H., Henriksen C.B. Harvesting European knowledge on soil functions and land management using multi-criteria decision analysis. Soil Use Manag. 2019; 35 :6–20. [ Google Scholar ]
- Bardgett R.D., van der Putten W.H. Belowground biodiversity and ecosystem functioning. Nature. 2014; 515 :505–511. [ PubMed ] [ Google Scholar ]
- Barrios E. Soil biota, ecosystem services and land productivity. Ecol. Econ. 2007; 64 :269–285. [ Google Scholar ]
- Barthod J., Rumpel C., Calabi-Floody M., Mora M.-L., Bolan N., Dignac M.-F. Adding worms during composting of organic waste with red mud and fly ash reduces CO2 emissions and increases plant available nutrient contents. J. Environ. Manag. 2018; 222 :207–215. [ PubMed ] [ Google Scholar ]
- Bartz M.L.C., Pasini A., Brown G.G. Earthworms as soil quality indicators in Brazilian no-tillage systems. Appl. Soil Ecol. 2013; 69 :39–48. [ Google Scholar ]
- Bateman I.J., Harwood A.R., Mace G.M., Watson R.T., Abson D.J., Andrews B. Bringing ecosystem services into economic decision-making: land use in the United Kingdom. Science. 2013; 341 :45–50. [ PubMed ] [ Google Scholar ]
- Baveye P.C., Baveye J., Gowdy J. Soil “ecosystem” services and natural capital: critical appraisal of research on uncertain ground. Frontiers in Environmental Science. 2016; 4 :41. [ Google Scholar ]
- Bender S.F., Wagg C., van der Heijden M.G. An underground revolution: biodiversity and soil ecological engineering for agricultural sustainability. Trends Ecol. Evol. 2016; 31 :440–452. [ PubMed ] [ Google Scholar ]
- Bhadauria T., Saxena K.G. Role of earthworms in soil fertility maintenance through the production of biogenic structures. Applied and environmental soil science. 2010; 2010 [ Google Scholar ]
- Bharati L., Lee K.-H., Isenhart T., Schultz R. Soil-water infiltration under crops, pasture, and established riparian buffer in Midwestern USA. Agrofor. Syst. 2002; 56 :249–257. [ Google Scholar ]
- Boardman J., Vandaele K., Evans R., Foster I.D. Off-site impacts of soil erosion and runoff: why connectivity is more important than erosion rates. Soil Use Manag. 2019; 35 :245–256. [ Google Scholar ]
- Bodin O. Collaborative environmental governance: achieving collective action in social-ecological systems. Science. 2017; 357 :659–+. [ PubMed ] [ Google Scholar ]
- Bolan N., Currie L., Baskaran S. Assessment of the influence of phosphate fertilizers on the microbial activity of pasture soils. Biol. Fertil. Soils. 1996; 21 :284–292. [ Google Scholar ]
- Bolan N.S., Adriano D.C., Curtin D. Soil acidification and liming interactions with nutrient and heavy metal transformation and bioavailability. Adv. Agron. 2003; 78 :5–272. [ Google Scholar ]
- Bolan N.S., Kunhikrishnan A., Naidu R. Carbon storage in a heavy clay soil landfill site after biosolid application. Sci. Total Environ. 2013; 465 :216–225. [ PubMed ] [ Google Scholar ]
- Bolan N., Kunhikrishnan A., Thangarajan R., Kumpiene J., Park J., Makino T. Remediation of heavy metal (loid) s contaminated soils–to mobilize or to immobilize? J. Hazard. Mater. 2014; 266 :141–166. [ PubMed ] [ Google Scholar ]
- Bond-Lamberty B., Bailey V.L., Chen M., Gough C.M., Vargas R. Globally rising soil heterotrophic respiration over recent decades. Nature. 2018; 560 :80. [ PubMed ] [ Google Scholar ]
- Bouma J. How to communicate soil expertise more effectively in the information age when aiming at the UN Sustainable Development Goals. Soil Use Manag. 2019; 35 :32–38. [ Google Scholar ]
- Bouma J., Montanarella L. Facing policy challenges with inter-and transdisciplinary soil research focused on the UN Sustainable Development Goals. Soil. 2016; 2 :135. [ Google Scholar ]
- Bouma J., Montanarella L., Evanylo G. The challenge for the soil science community to contribute to the implementation of the UN Sustainable Development Goals. Soil Use Manag. 2019; 35 :538–546. [ Google Scholar ]
- Bradney L., Wijesekara H., Palansooriya K.N., Obadamudalige N., Bolan N.S., Ok Y.S. Particulate plastics as a vector for toxic trace-element uptake by aquatic and terrestrial organisms and human health risk. Environ. Int. 2019; 131 [ PubMed ] [ Google Scholar ]
- Bryan B.A., Gao L., Ye Y., Sun X., Connor J.D., Crossman N.D. China’s response to a national land-system sustainability emergency. Nature. 2018; 559 :193–204. [ PubMed ] [ Google Scholar ]
- Bunge M., Adrian L., Kraus A., Opel M., Lorenz W.G., Andreesen J.R. Reductive dehalogenation of chlorinated dioxins by an anaerobic bacterium. Nature. 2003; 421 :357–360. [ PubMed ] [ Google Scholar ]
- Calisher C., Carroll D., Colwell R., Corley R.B., Daszak P., Drosten C. Statement in support of the scientists, public health professionals, and medical professionals of China combatting COVID-19. Lancet. 2020; 395 (10226):PE42–E43. [ PMC free article ] [ PubMed ] [ Google Scholar ]
- Carlson K.M., Curran L.M., Ratnasari D., Pittman A.M., Soares-Filho B.S., Asner G.P. Committed carbon emissions, deforestation, and community land conversion from oil palm plantation expansion in West Kalimantan, Indonesia. Proc. Natl. Acad. Sci. U. S. A. 2012; 109 :7559–7564. [ PMC free article ] [ PubMed ] [ Google Scholar ]
- Celentano D., Rousseau G.X., Engel V.L., Zelarayán M., Oliveira E.C., Araujo A.C.M. Degradation of riparian forest affects soil properties and ecosystem services provision in eastern Amazon of Brazil. Land Degrad. Dev. 2017; 28 :482–493. [ Google Scholar ]
- Chatskikh D., Olesen J.E., Hansen E.M., Elsgaard L., Petersen B.M. Effects of reduced tillage on net greenhouse gas fluxes from loamy sand soil under winter crops in Denmark. Agric. Ecosyst. Environ. 2008; 128 :117–126. [ Google Scholar ]
- Chibuike G., Burkitt L., Camps-Arbestain M., Bishop P., Bretherton M., Singh R. Effect of forage crop establishment on dissolved organic carbon dynamics and leaching in a hill country soil. Soil Use Manag. 2019; 35 :453–465. [ Google Scholar ]
- Chowdhury S., Farrell M., Butler G., Bolan N. Assessing the effect of crop residue removal on soil organic carbon storage and microbial activity in a no-till cropping system. Soil Use Manag. 2015; 31 :450–460. [ Google Scholar ]
- Corradini F., Meza P., Eguiluz R., Casado F., Huerta-Lwanga E., Geissen V. Evidence of microplastic accumulation in agricultural soils from sewage sludge disposal. Sci. Total Environ. 2019; 671 :411–420. [ PubMed ] [ Google Scholar ]
- Davidson E.A. The contribution of manure and fertilizer nitrogen to atmospheric nitrous oxide since 1860. Nat. Geosci. 2009; 2 :659. [ Google Scholar ]
- Dey R., Pal K.K., Tilak K.V.B.R. Influence of soil and plant types on diversity of rhizobacteria. Proceedings of the National Academy of Sciences India Section B-Biological Sciences. 2012; 82 :341–352. [ Google Scholar ]
- Dominati E., Patterson M., Mackay A. A framework for classifying and quantifying the natural capital and ecosystem services of soils. Ecol. Econ. 2010; 69 :1858–1868. [ Google Scholar ]
- Dong X., Singh B.P., Li G., Lin Q., Zhao X. Biochar has little effect on soil dissolved organic carbon pool 5 years after biochar application under field condition. Soil Use Manag. 2019; 35 :466–477. [ Google Scholar ]
- Donoghue S., Furley P.A., Stuart N., Haggis R., Trevaskis A., Lopez G. The nature and spatial variability of lowland savanna soils: improving the resolution of soil properties to support land management policy. Soil Use Manag. 2019; 35 :547–560. [ Google Scholar ]
- Doran J.W., Parkin T.B. Defining and assessing soil quality. Defining soil quality for a sustainable environment. 1994; 35 :1–21. [ Google Scholar ]
- Doran J., Safley M. CAB International; New York: 1997. Defining and Assessing Soil Health and Sustainable Productivity. Biological Indicators of Soil Health. [ Google Scholar ]
- Doran J.W., Zeiss M.R. Soil health and sustainability: managing the biotic component of soil quality. Appl. Soil Ecol. 2000; 15 :3–11. [ Google Scholar ]
- Eriksen-Hamel N.S., Speratti A.B., Whalen J.K., Légère A., Madramootoo C.A. Earthworm populations and growth rates related to long-term crop residue and tillage management. Soil Tillage Res. 2009; 104 :311–316. [ Google Scholar ]
- FAO . Earthscan; 2011. The State of the World’s Land and Water Resources for Food and Agriculture: Managing Systems at Risk. [ Google Scholar ]
- Farhate C.V., de Souza Z.M., La Scala N., Jr., de Sousa A.C.M., Santos A.P.G., Carvalho J.L.N. Soil tillage and cover crop on soil CO2 emissions from sugarcane fields. Soil Use Manag. 2019; 35 :273–282. [ Google Scholar ]
- Ford H., Healey J.R., Webb B., Pagella T.F., Smith A.R. How do hedgerows influence soil organic carbon stock in livestock-grazed pasture? Soil Use Manag. 2019; 35 :576–584. [ Google Scholar ]
- Franzluebbers A.J. Soil organic carbon sequestration and agricultural greenhouse gas emissions in the southeastern USA. Soil Tillage Res. 2005; 83 :120–147. [ Google Scholar ]
- Fry P., Thieme S. A social learning video method: identifying and sharing successful transformation knowledge for sustainable soil management in Switzerland. Soil Use Manag. 2019; 35 :185–194. [ Google Scholar ]
- Fu B., Merritt W.S., Croke B.F., Weber T.R., Jakeman A.J. A review of catchment-scale water quality and erosion models and a synthesis of future prospects. Environ. Model Softw. 2019; 114 :75–97. [ Google Scholar ]
- Garcia-Pichel F., Loza V., Marusenko Y., Mateo P., Potrafka R.M. Temperature drives the continental-scale distribution of key microbes in topsoil communities. Science. 2013; 340 :1574–1577. [ PubMed ] [ Google Scholar ]
- Giannitsopoulos M.L., Burgess P.J., Rickson R.J. Effects of conservation tillage drills on soil quality indicators in a wheat–oilseed rape rotation: organic carbon, earthworms and water-stable aggregates. Soil Use Manag. 2020; 36 :139–152. [ Google Scholar ]
- van Gool D. 2016. Identifying Soil Constraints That Limit Wheat Yield in the South-west of Western Australia. [ Google Scholar ]
- Gossner M.M., Lewinsohn T.M., Kahl T., Grassein F., Boch S., Prati D. Land-use intensification causes multitrophic homogenization of grassland communities. Nature. 2016; 540 :266–+. [ PubMed ] [ Google Scholar ]
- Guoju X., Yanbin H., Qiang Z., Jing W., Ming L. Impact of cultivation on soil organic carbon and carbon sequestration potential in semiarid regions of China. Soil Use Manag. 2020; 36 :83–92. [ Google Scholar ]
- Hammond R.A., Dubé L. A systems science perspective and transdisciplinary models for food and nutrition security. Proc. Natl. Acad. Sci. 2012; 109 :12356–12363. [ PMC free article ] [ PubMed ] [ Google Scholar ]
- Hollesen J., Matthiesen H., Møller A.B., Elberling B. Permafrost thawing in organic Arctic soils accelerated by ground heat production. Nat. Clim. Chang. 2015; 5 :574–578. [ Google Scholar ]
- Hou D. Elsevier Inc; 2020. Sustainable Remediation of Contaminated Soil and Groundwater: Materials, Processes, and Assessment. [ Google Scholar ]
- Hou D., Ok Y.S. Speed up mapping of soil pollution. Nature. 2019; 566 :455. [ PubMed ] [ Google Scholar ]
- Illiger P., Schmidt G., Walde I., Hese S., Kudrjavzev A.E., Kurepina N. Estimation of regional soil organic carbon stocks merging classified land-use information with detailed soil data. Sci. Total Environ. 2019; 695 [ PubMed ] [ Google Scholar ]
- Imhof M.P., Heemskerk G.E., Cox M.T. Soil information management and knowledge sharing in Victoria, Australia—user perspectives. Soil Use Manag. 2019; 35 :39–51. [ Google Scholar ]
- Inam A., Adamowski J., Prasher S., Halbe J., Malard J., Albano R. Coupling of a distributed stakeholder-built system dynamics socio-economic model with SAHYSMOD for sustainable soil salinity management–part 1: model development. J. Hydrol. 2017; 551 :596–618. [ Google Scholar ]
- Ingram J., Mills J. Are advisory services “fit for purpose” to support sustainable soil management? An assessment of advice in Europe. Soil Use Manag. 2019; 35 :21–31. [ Google Scholar ]
- Janvier C., Villeneuve F., Alabouvette C., Edel-Hermann V., Mateille T., Steinberg C. Soil health through soil disease suppression: which strategy from descriptors to indicators? Soil Biol. Biochem. 2007; 39 :1–23. [ Google Scholar ]
- Jenkins A., Beange L., Morris S. Using webinars to extend the reach of soil learning in New South Wales: a first look. Soil Use Manag. 2019; 35 :52–62. [ Google Scholar ]
- Jia X., O’Connor D., Hou D., Jin Y., Li G., Zheng C. Groundwater depletion and contamination: spatial distribution of groundwater resources sustainability in China. Sci. Total Environ. 2019; 672 :551–562. [ PubMed ] [ Google Scholar ]
- Jia H., Hou D., O’Connor D., Pan S., Zhu J., Bolan N.S. Exogenous phosphorus treatment facilitates chelation-mediated cadmium detoxification in perennial ryegrass (Lolium perenne L.) J. Hazard. Mater. 2020; 389 [ PubMed ] [ Google Scholar ]
- Jing J., Christensen J.T., Sørensen P., Christensen B.T., Rubæk G.H. Long-term effects of animal manure and mineral fertilizers on phosphorus availability and silage maize growth. Soil Use Manag. 2019; 35 :323–333. [ Google Scholar ]
- Kahlon M.S., Lal R., Ann-Varughese M. Twenty two years of tillage and mulching impacts on soil physical characteristics and carbon sequestration in Central Ohio. Soil Tillage Res. 2013; 126 :151–158. [ Google Scholar ]
- Keesstra S.D., Bouma J., Wallinga J., Tittonell P., Smith P., Cerdà A. The significance of soils and soil science towards realization of the United Nations Sustainable Development Goals. Soil. 2016; 2 :111–128. doi: 10.5194/soil-2-111-2016. [ CrossRef ] [ Google Scholar ]
- Kell D.B. Large-scale sequestration of atmospheric carbon via plant roots in natural and agricultural ecosystems: why and how. Philosophical Transactions of the Royal Society B: Biological Sciences. 2012; 367 :1589–1597. [ PMC free article ] [ PubMed ] [ Google Scholar ]
- Khan S.U. Elsevier; 2016. Pesticides in the Soil Environment. [ Google Scholar ]
- Kibblewhite M., Ritz K., Swift M. Soil health in agricultural systems. Philosophical Transactions of the Royal Society B: Biological Sciences. 2008; 363 :685–701. [ PMC free article ] [ PubMed ] [ Google Scholar ]
- Klein J.T., Newell W.H. Handbook of the Undergraduate Curriculum: A Comprehensive Guide to Purposes, Structures, Practices, and Change. 1997. Advancing interdisciplinary studies; pp. 393–415. [ Google Scholar ]
- Knox O.G., Osanai Y., Polain K., Pereg L., Nachimuthu G., Wilson B. Lessons from extension activity related to cotton rotation impacts on soil—a scientist’s perspective. Soil Use Manag. 2019; 35 :141–149. [ Google Scholar ]
- Kota S., Giambene G. Proceedings of the Eleventh International Conference on Advances in Satellite and Space Communications-SPACOMM. 2019. Satellite 5G: IoT use case for rural areas applications; pp. 24–28. [ Google Scholar ]
- Krzywoszynska A. Making knowledge and meaning in communities of practice: what role may science play? The case of sustainable soil management in England. Soil Use Manag. 2019; 35 :160–168. [ Google Scholar ]
- Kumar V., Gathala M.K., Saharawat Y.S., Parihar C.M., Kumar R., Kumar R. Impact of tillage and crop establishment methods on crop yields, profitability and soil physical properties in rice–wheat system of indo-Gangetic Plains of India. Soil Use Manag. 2019; 35 :303–313. [ Google Scholar ]
- Kunhikrishnan A., Thangarajan R., Bolan N., Xu Y., Mandal S., Gleeson D. Functional relationships of soil acidification, liming, and greenhouse gas flux. Adv. Agron. 2016; 139 :1–71. Elsevier. [ Google Scholar ]
- Lafond G.P., Walley F., May W., Holzapfel C. Long term impact of no-till on soil properties and crop productivity on the Canadian prairies. Soil Tillage Res. 2011; 117 :110–123. [ Google Scholar ]
- Laird D., Fleming P., Wang B., Horton R., Karlen D. Biochar impact on nutrient leaching from a Midwestern agricultural soil. Geoderma. 2010; 158 :436–442. [ Google Scholar ]
- Laird D.A., Fleming P., Davis D.D., Horton R., Wang B., Karlen D.L. Impact of biochar amendments on the quality of a typical Midwestern agricultural soil. Geoderma. 2010; 158 :443–449. [ Google Scholar ]
- Lal R. Soil erosion and the global carbon budget. Environ. Int. 2003; 29 :437–450. [ PubMed ] [ Google Scholar ]
- Lauber C.L., Hamady M., Knight R., Fierer N. Pyrosequencing-based assessment of soil pH as a predictor of soil bacterial community structure at the continental scale. Appl. Environ. Microbiol. 2009; 75 :5111–5120. [ PMC free article ] [ PubMed ] [ Google Scholar ]
- Lavelle P., Decaëns T., Aubert M., Sb Barot, Blouin M., Bureau F. Soil invertebrates and ecosystem services. Eur. J. Soil Biol. 2006; 42 :S3–S15. [ Google Scholar ]
- Le Quere C., Andrew R.M., Friedlingstein P., Sitch S., Hauck J., Pongratz J. Global carbon budget 2018. Earth System Science Data. 2018; 10 :2141–2194. [ Google Scholar ]
- Leff J.W., Jones S.E., Prober S.M., Barberan A., Borer E.T., Firn J.L. Consistent responses of soil microbial communities to elevated nutrient inputs in grasslands across the globe. Proc. Natl. Acad. Sci. U. S. A. 2015; 112 :10967–10972. [ PMC free article ] [ PubMed ] [ Google Scholar ]
- Lehmann J., Joseph S. Earthscan London; 2009. Biochar for Environmental Management. [ Google Scholar ]
- Li J., Liu Y., Hai X., Shangguan Z., Deng L. Dynamics of soil microbial C:N:P stoichiometry and its driving mechanisms following natural vegetation restoration after farmland abandonment. Sci. Total Environ. 2019; 693 [ PubMed ] [ Google Scholar ]
- Li X., Han H., Ning T., Shen Y., Lal R. Variations of SOC and MBC observed in an incubated brown loam soil managed under different tillage systems for 12 years. Soil Use Manag. 2019; 35 :585–594. [ Google Scholar ]
- Liu J., Li S., Ouyang Z., Tam C., Chen X. Ecological and socioeconomic effects of China’s policies for ecosystem services. Proc. Natl. Acad. Sci. U. S. A. 2008; 105 :9477–9482. [ PMC free article ] [ PubMed ] [ Google Scholar ]
- Lobry de Bruyn L.A. Learning opportunities: understanding farmers’ soil testing practice through workshop activities to improve extension support for soil health management. Soil Use Manag. 2019; 35 :128–140. [ Google Scholar ]
- Lochon I., Carrère P., Yvin J.-C., Houdusse-Lemenager D., Bloor J.M.G. Impacts of low-level liming on soil respiration and forage production in a fertilized upland grassland in Central France. Sci. Total Environ. 2019; 697 [ PubMed ] [ Google Scholar ]
- Ma L., Abuduwaili J., Li Y., Liu W. Anthropogenically disturbed potentially toxic elements in roadside topsoils of a suburban region of Bishkek, Central Asia. Soil Use Manag. 2019; 35 :283–292. [ Google Scholar ]
- Maguire R.O., Sims J.T. Soil testing to predict phosphorus leaching. J. Environ. Qual. 2002; 31 :1601–1609. [ PubMed ] [ Google Scholar ]
- Maia S.M.F., Gonzaga G.B.M., Silva LkdS, Lyra G.B., Gomes TcdA. Soil organic carbon temperature sensitivity of different soil types and land use systems in the Brazilian semi-arid region. Soil Use Manag. 2019; 35 :433–442. [ Google Scholar ]
- Maltas A., Kebli H., Oberholzer H.R., Weisskopf P., Sinaj S. The effects of organic and mineral fertilizers on carbon sequestration, soil properties, and crop yields from a long-term field experiment under a Swiss conventional farming system. Land Degrad. Dev. 2018; 29 :926–938. [ Google Scholar ]
- Manzoni S., Porporato A. Soil carbon and nitrogen mineralization: theory and models across scales. Soil Biol. Biochem. 2009; 41 :1355–1379. [ Google Scholar ]
- McInnes-Clarke S.K., Jenkins B.R., Rawson A., Murphy B.W. Sharing soil knowledge and evaluating progress in the New South Wales Soil Knowledge Network. Soil Use Manag. 2019; 35 :105–116. [ Google Scholar ]
- Mehra P., Baker J., Sojka R.E., Bolan N., Desbiolles J., Kirkham M.B. A review of tillage practices and their potential to impact the soil carbon dynamics. Adv. Agron. 2018; 150 :185–230. Elsevier. [ Google Scholar ]
- MEP . Ministry of Environmental Protection; Beijing, China: 2014. National Soil Contamination Survey Report. [ Google Scholar ]
- Mills J., Reed M., Skaalsveen K., Ingram J. The use of Twitter for knowledge exchange on sustainable soil management. Soil Use Manag. 2019; 35 :195–203. [ Google Scholar ]
- Montgomery D.R. Soil erosion and agricultural sustainability. Proc. Natl. Acad. Sci. U. S. A. 2007; 104 :13268–13272. [ PMC free article ] [ PubMed ] [ Google Scholar ]
- Montgomery D.R. Soil erosion and agricultural sustainability. Proc. Natl. Acad. Sci. 2007; 104 :13268–13272. [ PMC free article ] [ PubMed ] [ Google Scholar ]
- Morais R., Silva N., Mendes J., Adão T., Pádua L., López-Riquelme J. Mysense: a comprehensive data management environment to improve precision agriculture practices. Comput. Electron. Agric. 2019; 162 :882–894. [ Google Scholar ]
- Mossadeghi-Björklund M., Jarvis N., Larsbo M., Forkman J., Keller T. Effects of compaction on soil hydraulic properties, penetration resistance and water flow patterns at the soil profile scale. Soil Use Manag. 2019; 35 :367–377. [ Google Scholar ]
- Mugandani R., Mafongoya P. Behaviour of smallholder farmers towards adoption of conservation agriculture in Zimbabwe. Soil Use Manag. 2019; 35 (4):561–575. [ Google Scholar ]
- Nachimuthu G., Watkins M.D., Hulugalle N.R., Weaver T.B., Finlay L.A., McCorkell B.E. Leaching of dissolved organic carbon and nitrogen under cotton farming systems in a Vertisol. Soil Use Manag. 2019; 35 :443–452. [ Google Scholar ]
- Neher D.A. Role of nematodes in soil health and their use as indicators. J. Nematol. 2001; 33 :161. [ PMC free article ] [ PubMed ] [ Google Scholar ]
- Nielsen M.N., Winding A., Binnerup S. 2002. Microorganisms as Indicators of Soil Health. [ Google Scholar ]
- Obrist D., Agnan Y., Jiskra M., Olson C.L., Colegrove D.P., Hueber J. Tundra uptake of atmospheric elemental mercury drives Arctic mercury pollution. Nature. 2017; 547 :201. [ PubMed ] [ Google Scholar ]
- O’Connor D., Hou D. More haste, less speed in replenishing China’s groundwater. Nature. 2019; 569 :487. [ PubMed ] [ Google Scholar ]
- O’Connor D., Hou D., Ok Y.S., Song Y., Sarmah A., Li X. Sustainable in situ remediation of recalcitrant organic pollutants in groundwater with controlled release materials: a review. J. Control. Release. 2018; 283 :200–213. [ PubMed ] [ Google Scholar ]
- O’Connor D., Peng T., Li G., Wang S., Duan L., Mulder J. Sulfur-modified rice husk biochar: a green method for the remediation of mercury contaminated soil. Sci. Total Environ. 2018; 621 :819–826. [ PubMed ] [ Google Scholar ]
- O’Connor D., Peng T., Zhang J., Tsang D.C., Alessi D.S., Shen Z. Biochar application for the remediation of heavy metal polluted land: a review of in situ field trials. Sci. Total Environ. 2018; 619 :815–826. [ PubMed ] [ Google Scholar ]
- O’Connor D., Hou D., Ok Y.S., Mulder J., Duan L., Wu Q. Mercury speciation, transformation, and transportation in soils, atmospheric flux, and implications for risk management: a critical review. Environ. Int. 2019; 126 :747–761. [ PubMed ] [ Google Scholar ]
- O’Connor D., Pan S., Shen Z., Song Y., Jin Y., Wu W.-M. Microplastics undergo accelerated vertical migration in sand soil due to small size and wet-dry cycles. Environ. Pollut. 2019; 249 :527–534. [ PubMed ] [ Google Scholar ]
- O’Connor D., Hou D., Ok Y.S., Lanphear B.P. The effects of iniquitous lead exposure on health. Nature Sustainability. 2020; 3 :77–79. [ Google Scholar ]
- Oliver M.A., Gregory P. Soil, food security and human health: a review. Eur. J. Soil Sci. 2015; 66 :257–276. [ Google Scholar ]
- Oliver D.P., Li Y., Orr R., Nelson P., Barnes M., McLaughlin M.J. The role of surface charge and pH changes in tropical soils on sorption behaviour of per- and polyfluoroalkyl substances (PFASs) Sci. Total Environ. 2019; 673 :197–206. [ PubMed ] [ Google Scholar ]
- Ottoy S., Vanierschot L., Dondeyne S., Vancampenhout K., Hermy M., Van Orshoven J. The devil is in the detail: discrepancy between soil organic carbon stocks estimated from regional and local data sources in Flanders, Belgium. Soil Use Manag. 2019; 35 :421–432. [ Google Scholar ]
- Packer I.J., Chapman G.A., Lawrie J.W. On-ground extension of soil information to improve land management. Soil Use Manag. 2019; 35 :75–84. [ Google Scholar ]
- Paustian K., Lehmann J., Ogle S., Reay D., Robertson G.P., Smith P. Climate-smart soils. Nature. 2016; 532 :49–57. [ PubMed ] [ Google Scholar ]
- Pavan A.L.R., Ometto A.R. Ecosystem Services in Life Cycle Assessment: a novel conceptual framework for soil. Sci. Total Environ. 2018; 643 :1337–1347. [ PubMed ] [ Google Scholar ]
- Pimentel D., Harvey C., Resosudarmo P., Sinclair K., Kurz D., McNair M. Environmental and economic costs of soil erosion and conservation benefits. Science. 1995; 267 :1117–1123. [ PubMed ] [ Google Scholar ]
- Pulleman M., Creamer R., Hamer U., Helder J., Pelosi C., Peres G. Soil biodiversity, biological indicators and soil ecosystem services—an overview of European approaches. Curr. Opin. Environ. Sustain. 2012; 4 :529–538. [ Google Scholar ]
- Qi S., Luo J., O’Connor D., Wang Y., Hou D. A numerical model to optimize LNAPL remediation by multi-phase extraction. Sci. Total Environ. 2020; 718 [ PubMed ] [ Google Scholar ]
- Rafiq M.K., Bai Y., Aziz R., Rafiq M.T., Mašek O., Bachmann R.T. Biochar amendment improves alpine meadows growth and soil health in Tibetan plateau over a three year period. Sci. Total Environ. 2019:135296. [ PubMed ] [ Google Scholar ]
- Ramesh T., Bolan N.S., Kirkham M.B., Wijesekara H., Kanchikerimath M., Rao C.S. Soil organic carbon dynamics: impact of land use changes and management practices: a review. Adv. Agron. 2019; 156 :1–107. [ Google Scholar ]
- Repko A.F., Szostak R. SAGE Publications, Incorporated; 2020. Interdisciplinary Research: Process and Theory. [ Google Scholar ]
- Rinot O., Levy G.J., Steinberger Y., Svoray T., Eshel G. Soil health assessment: a critical review of current methodologies and a proposed new approach. Sci. Total Environ. 2019; 648 :1484–1491. [ PubMed ] [ Google Scholar ]
- Robinson D., Fraser I., Dominati E., Davíðsdóttir B., Jónsson J., Jones L. On the value of soil resources in the context of natural capital and ecosystem service delivery. Soil Sci. Soc. Am. J. 2014; 78 :685–700. [ Google Scholar ]
- Robinson N.J., Dahlhaus P.G., Wong M., MacLeod A., Jones D., Nicholson C. Testing the public–private soil data and information sharing model for sustainable soil management outcomes. Soil Use Manag. 2019; 35 :94–104. [ Google Scholar ]
- Rojas R.V., Achouri M., Maroulis J., Caon L. Healthy soils: a prerequisite for sustainable food security. Environ. Earth Sci. 2016; 75 :180. [ Google Scholar ]
- Rumpel C., Amiraslani F., Koutika L.-S., Smith P., Whitehead D., Wollenberg E. Put more carbon in soils to meet Paris climate pledges. Nature. 2018; 564 :32–34. [ PubMed ] [ Google Scholar ]
- Saikia R., Sharma S., Thind H.S., Singh Y. Tillage and residue management practices affect soil biological indicators in a rice-wheat cropping system in north-western India. Soil Use Manag. 2020; 36 :157–172. [ Google Scholar ]
- Sanchez P.A. Soil fertility and hunger in Africa. Science. 2002; 295 :2019–2020. [ PubMed ] [ Google Scholar ]
- Schuur E.A., Abbott B., Bowden W., Brovkin V., Camill P., Canadell J. Expert assessment of vulnerability of permafrost carbon to climate change. Clim. Chang. 2013; 119 :359–374. [ Google Scholar ]
- Schuur E.A., McGuire A.D., Schädel C., Grosse G., Harden J., Hayes D.J. Climate change and the permafrost carbon feedback. Nature. 2015; 520 :171–179. [ PubMed ] [ Google Scholar ]
- Schwenke G., Beange L., Cameron J., Bell M., Harden S. What soil information do crop advisors use to develop nitrogen fertilizer recommendations for grain growers in New South Wales, Australia? Soil Use Manag. 2019; 35 :85–93. [ Google Scholar ]
- Senbayram M., Saygan E.P., Chen R., Aydemir S., Kaya C., Wu D. Effect of biochar origin and soil type on the greenhouse gas emission and the bacterial community structure in N fertilised acidic sandy and alkaline clay soil. Sci. Total Environ. 2019; 660 :69–79. [ PubMed ] [ Google Scholar ]
- Shen Z., Jin F., O’Connor D., Hou D. Solidification/stabilization for soil remediation: an old technology with new vitality. Environmental Science & Technology. 2019; 53 :11615–11617. [ PubMed ] [ Google Scholar ]
- Song Y., Kirkwood N., Maksimovic C., Zhen X., O’Connor D., Jin Y. Nature based solutions for contaminated land remediation and brownfield redevelopment in cities: a review. Sci. Total Environ. 2019; 663 :568–579. [ PubMed ] [ Google Scholar ]
- Stoate C., Jones S., Crotty F., Morris C., Seymour S. Participatory research approaches to integrating scientific and farmer knowledge of soil to meet multiple objectives in the English east midlands. Soil Use Manag. 2019; 35 :150–159. [ Google Scholar ]
- Su C., Liu H., Wang S. A process-based framework for soil ecosystem services study and management. Sci. Total Environ. 2018; 627 :282–289. [ PubMed ] [ Google Scholar ]
- Tavares R.L.M., de Souza Z.M., Siqueira D.S., La Scala Júnior N., Panosso A.R., Campos M.C.C. Soil CO2 emission in sugarcane management systems. Acta Agriculturae Scandinavica, Section B—Soil & Plant Science. 2015; 65 :755–762. [ Google Scholar ]
- Teng M., Huang C., Wang P., Zeng L., Zhou Z., Xiao W. Impacts of forest restoration on soil erosion in the Three Gorges Reservoir area, China. Sci. Total Environ. 2019; 697 [ PubMed ] [ Google Scholar ]
- Tisdale S.L., Nelson W.L., Beaton J.D. Collier Macmillan Publishers; 1985. Soil Fertility and Fertilizers. [ Google Scholar ]
- Totsche K.U., Rennert T., Gerzabek M.H., Kögel-Knabner I., Smalla K., Spiteller M. Biogeochemical interfaces in soil: the interdisciplinary challenge for soil science. J. Plant Nutr. Soil Sci. 2010; 173 :88–99. [ Google Scholar ]
- Tulau M.J., McInnes-Clarke S.K., Yang X., McAlpine R.A., Karunaratne S.B., Zhu Q. The Warrumbungle post-fire recovery project—raising the profile of soils. Soil Use Manag. 2019; 35 :63–74. [ Google Scholar ]
- Ukalska-Jaruga A., Klimkowicz-Pawlas A., Smreczak B. Characterization of organic matter fractions in the top layer of soils under different land uses in Central-Eastern Europe. Soil Use Manag. 2019; 35 :595–606. [ Google Scholar ]
- UN . 2015. Transforming our World: The 2030 Agenda for Sustainable Development A/RES/70/1. [ Google Scholar ]
- UN . 2019. The Sustainable Development Goals Report, New York. [ Google Scholar ]
- UNCCD . 2017. Global Land Outlook. [ Google Scholar ]
- Van Bruggen A.H., Semenov A.M. In search of biological indicators for soil health and disease suppression. Appl. Soil Ecol. 2000; 15 :13–24. [ Google Scholar ]
- Vogel H.-J., Bartke S., Daedlow K., Helming K., Kögel-Knabner I., Lang B. A systemic approach for modeling soil functions. Soil. 2018; 4 :83. [ Google Scholar ]
- Wang J., Liu X., Li Y., Powell T., Wang X., Wang G. Microplastics as contaminants in the soil environment: a mini-review. Sci. Total Environ. 2019; 691 :848–857. [ PubMed ] [ Google Scholar ]
- Wang L., Hou D., Shen Z., Zhu J., Jia X., Ok Y.S. Field trials of phytomining and phytoremediation: a critical review of influencing factors and effects of additives. Crit. Rev. Environ. Sci. Technol. 2019:1–51. [ Google Scholar ]
- Wang L., Hou D., Cao Y., Ok Y.S., Tack F.M., Rinklebe J. Remediation of mercury contaminated soil, water, and air: a review of emerging materials and innovative technologies. Environ. Int. 2020; 134 [ PubMed ] [ Google Scholar ]
- Wang L., Li X., Tsang D.C., Jin F., Hou D. Green remediation of Cd and Hg contaminated soil using humic acid modified montmorillonite: immobilization performance under accelerated ageing conditions. J. Hazard. Mater. 2020:122005. [ PubMed ] [ Google Scholar ]
- Wang L., Ok Y.S., Tsang D.C., Alessi D.S., Rinklebe J., Wang H. New trends in biochar pyrolysis and modification strategies: feedstock, pyrolysis conditions, sustainability concerns and implications for soil amendment. Soil Use Manag. 2020 doi: 10.1111/sum.12592. (In Press) [ CrossRef ] [ Google Scholar ]
- Welten B.G., Ledgard S.F., Judge A.A., Sprosen M.S., McGowan A.W., Dexter M.M. Efficacy of different temperate pasture species to reduce nitrogen leaching from cattle urine applied in different seasons: a soil lysimeter study. Soil Use Manag. 2019; 35 :653–663. [ Google Scholar ]
- Wick A.F., Haley J., Gasch C., Wehlander T., Briese L., Samson-Liebig S. Network-based approaches for soil health research and extension programming in North Dakota, USA. Soil Use Manag. 2019; 35 :177–184. [ Google Scholar ]
- Wilcke W. Synopsis polycyclic aromatic hydrocarbons (PAHs) in soil—a review. J. Plant Nutr. Soil Sci. 2000; 163 :229–248. [ Google Scholar ]
- Wolfert S., Ge L., Verdouw C., Bogaardt M.-J. Big data in smart farming–a review. Agric. Syst. 2017; 153 :69–80. [ Google Scholar ]
- Woolf D., Amonette J.E., Street-Perrott F.A., Lehmann J., Joseph S. Sustainable biochar to mitigate global climate change. Nat. Commun. 2010; 1 :56. [ PMC free article ] [ PubMed ] [ Google Scholar ]
- Wu T., Ayres E., Bardgett R.D., Wall D.H., Garey J.R. Molecular study of worldwide distribution and diversity of soil animals. Proc. Natl. Acad. Sci. 2011; 108 :17720–17725. [ PMC free article ] [ PubMed ] [ Google Scholar ]
- Xiao J., Wang L., Deng L., Jin Z. Characteristics, sources, water quality and health risk assessment of trace elements in river water and well water in the Chinese Loess Plateau. Sci. Total Environ. 2019; 650 :2004–2012. [ PubMed ] [ Google Scholar ]
- Xu L., Wang M., Tian Y., Shi X., Shi Y., Yu Q. Changes in soil macropores: superposition of the roles of organic nutrient amendments and the greenhouse pattern in vegetable plantations. Soil Use Manag. 2019; 35 :412–420. [ Google Scholar ]
- Yagi K., Minami K. Effect of organic matter application on methane emission from some Japanese paddy fields. Soil science and plant nutrition. 1990; 36 :599–610. [ Google Scholar ]
- Ye L., Camps-Arbestain M., Shen Q., Lehmann J., Singh B., Sabir M. Biochar effects on crop yields with and without fertilizer: a meta-analysis of field studies using separate controls. Soil Use Manag. 2020; 36 :2–18. [ Google Scholar ]
- Yu H., Zha T., Zhang X., Ma L. Vertical distribution and influencing factors of soil organic carbon in the Loess Plateau, China. Sci. Total Environ. 2019; 693 [ PubMed ] [ Google Scholar ]
- Zhang W.-F., Z-x Dou, He P., Ju X.-T., Powlson D., Chadwick D. New technologies reduce greenhouse gas emissions from nitrogenous fertilizer in China. Proc. Natl. Acad. Sci. U. S. A. 2013; 110 :8375–8380. [ PMC free article ] [ PubMed ] [ Google Scholar ]
- Zhang Y., Hou D., O’Connor D., Shen Z., Shi P., Ok Y.S. Lead contamination in Chinese surface soils: source identification, spatial-temporal distribution and associated health risks. Crit. Rev. Environ. Sci. Technol. 2019; 49 (15) [ Google Scholar ]
- Zhang J., Hou D., Shen Z., Jin F., O’Connor D., Pan S. Effects of excessive impregnation, magnesium content, and pyrolysis temperature on MgO-coated watermelon rind biochar and its lead removal capacity. Environ. Res. 2020; 183 :109152. [ PubMed ] [ Google Scholar ]
- Zhang Y., O’Connor D., Xu W., Hou D. Blood lead levels among Chinese children: the shifting influence of industry, traffic, and e-waste over three decades. Environ. Int. 2020; 135 [ PubMed ] [ Google Scholar ]
- Zhao H., Ning P., Chen Y., Liu J., Ghaffar S.A., Xiaohong T. Effect of straw amendment modes on soil organic carbon, nitrogen sequestration and crop yield on the North-Central Plain of China. Soil Use Manag. 2019; 35 :511–525. [ Google Scholar ]
REVIEW article
Soil health and its improvement through novel agronomic and innovative approaches.
- 1 Central Agricultural University, Imphal, India
- 2 Division of Agronomy, Indian Council of Agricultural Research-Indian Agricultural Research Institute, New Delhi, India
Soil is an important natural resource providing water, nutrient, and mechanical support for plant growth. In agroecosystem, continuous manipulation of soil is going on due to addition of input, removal of nutrients, changing water balance, and microbial life. These processes affect soil properties (physical, chemical, and biological), and the deviation of these properties from the normal status is controlled by soil buffering capacity and soil resilience. If these changes are beyond the reach of soil resilience, then soil loses its original state, leading to soil degradation. At present, the extent of the degraded area in the world is 1,036 to 1,470 million ha. This urges the need for maintaining soil health rather than the mere addition of input for crop production. Soil health is an integrative property that reflects the capacity of soil to respond to agricultural intervention, so that it continues to support both agricultural production and the provision of other ecosystem services. Maintaining the physical, chemical, and biological properties of soil is needed to keep it healthy, and this is possible through the adoption of different agronomic approaches. The diversification of nutrient sources with emphasis on organic sources, adoption of principles of conservation agriculture, enhancement of soil microbial diversity, efficient resource recycling through the integrated farming system, and amendment addition for correcting soil reactions are potential options for improving soil health, and are discussed in this review. This article reviewed the concept of soil health and its development, issues related to soil health, and indicators of healthy soil. At the same time, the impact of the ill health of the soil on crop productivity and resource use efficiency reported in different parts of the world in recent years are also reviewed. The agro-techniques such as green and brown manuring in arable land and agroforestry on degraded and marginal land were followed on piece meal basis and for economic gain. The potential of these and several other options for maintaining soil need to be recognized, evaluated, and quantified for their wider application on the front of soil health management avenues. The use of crop residue, agro-industrial waste, and untreated mineral or industrial waste (basic slag, phosphogypsum, etc.) as soil amendments has a huge potential in maintaining healthy soil along with serving as sources of crop nutrition. The review emphasizes the evaluation and quantification of present-day followed agro-techniques for their contribution to soil health improvement across agro-climatic regions and for wider implications. Furthermore, emphasis is given to innovative approaches for soil health management rather than mere application of manures and fertilizers for crop nutrition.
Introduction to Soil Health
The soil, supplier of water, nutrient, and mechanical support to crop plants, is explained as four-dimensional, unconsolidated, and dynamic in nature ( Lal, 2016 ). The major components of the soil system consist of mineral matter, which acts as an inherent source of 14 essential mineral plant nutrients and organic matter, which acts as a storehouse (Elixir). Soil also supplies essential mineral plant nutrients along with carbon and pore space occupied by water and air supplying three basic non-mineral plant nutrients viz ., carbon (C), oxygen (O), and hydrogen (H). In the ideal state, the proportions of these factors are 45% mineral matter and 5% organic matter; while the remaining 50% is occupied by pore space. This four-dimensional nature and distinct proportions of solid and pore space give soil distinct physical, chemical, and biological properties that change over time dimensions. Any significant variation in these factors beyond the range of crop tolerance limits makes soil unfit for crop cultivation and will be the most important reason for soil illness. The tolerance limit for plant growth is expressed as the different parameters that express the physical, chemical, and biological properties of the soils; while the soil with all properties in the acceptable range is considered healthy.
Soil health is defined by various authors in different ways because of the involvement of a large number of soil health indicators ( Van Bruggen and Semenov, 2000 ; Nielsen and Winding, 2002 ; Brevik, 2009 ; Katyal et al., 2016 ; Haney et al., 2018 ; Wander et al., 2019 ) and their suitable combination for different land use systems. Definitions given by different authors and organization are shown in Table 1 . The concept of soil health started with the use of the term “soil health” by Wallace (1910) in regard to the capacity of humus to provide a solution to almost all soil-related problems and the major historical development of the concept of soil health ( Brivik, 2018 ) is shown in Table 2 . As different soil properties are considered in explaining the concept of soil health, and act as indicators of soil health, it can also be defined in terms of soil properties viz . soil physical health, soil chemical health, and soil biological health. The soil with the ability to meet plant and ecosystem requirements for water, aeration, and strength over time, and to resist and recover from processes that might diminish this ability is considered as physically healthy ( McKenzie et al., 2011 ; Are, 2019 ). Soil biological health is the ability of soil to support large and diverse microbial communities, suppress pathogens, and support healthy crop development ( Brackin et al., 2017 ); while chemically healthy soil has plant nutrients in optimum quantity, available form, and balanced proportions, and which are available to plants without the hindrance of other chemical compound and properties. Soil chemical health also considers the presence or absence of harmful soil agrochemicals and pollutants.
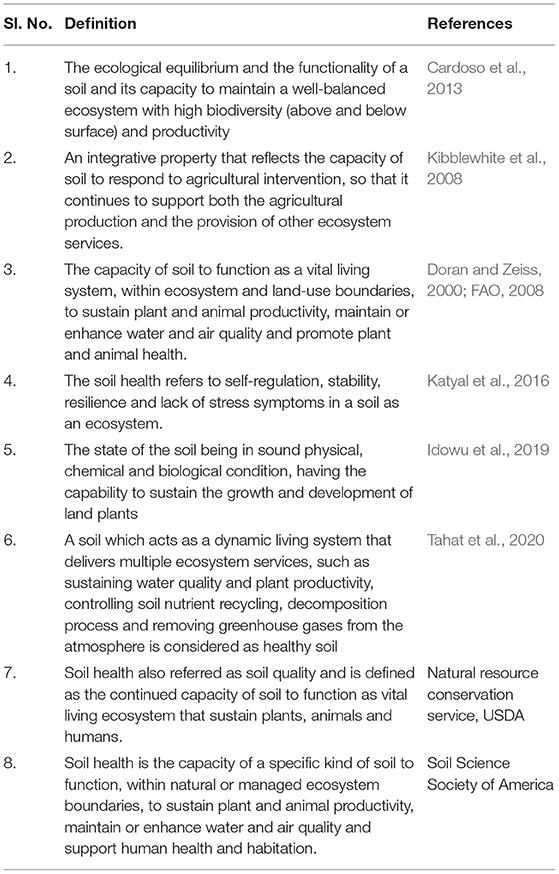
Table 1 . Definitions of soil health given by different authors.
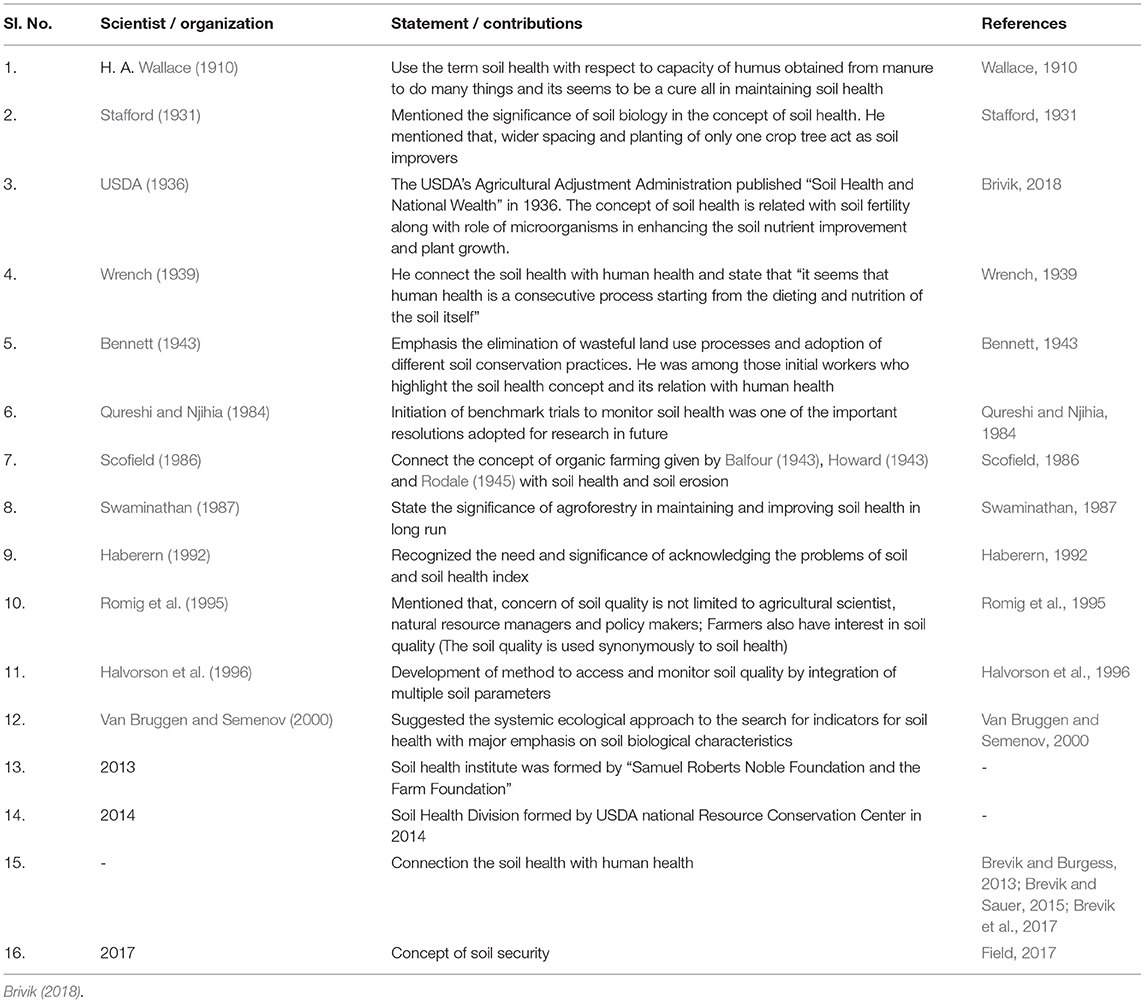
Table 2 . Historical development of the concept of soil health.
Considering the variety of chemical, physical, and biological properties of soils, there were attempts to categorize some soil properties as indicators of soil health ( Magdoff, 2001 ; Brevik, 2009 ; Cardoso et al., 2013 ; Haney et al., 2018 ; Pawlas et al., 2019 ), which are mentioned in Table 3 . Along with soil health indicators, Magdoff (2001) listed the characteristics of healthy soil ( Table 4 ). The importance of soil health in sustaining the agricultural ecosystem is well-recognized ( Wienhold et al., 2008 ; Jat et al., 2015 ; NAAS, 2018 ; Jian et al., 2020 ; Tahat et al., 2020 ), and considering the varied levels of sensitivity of soil health indicators ( Table 3 ), it is imperative to discuss the different issues and concerns of soil health.
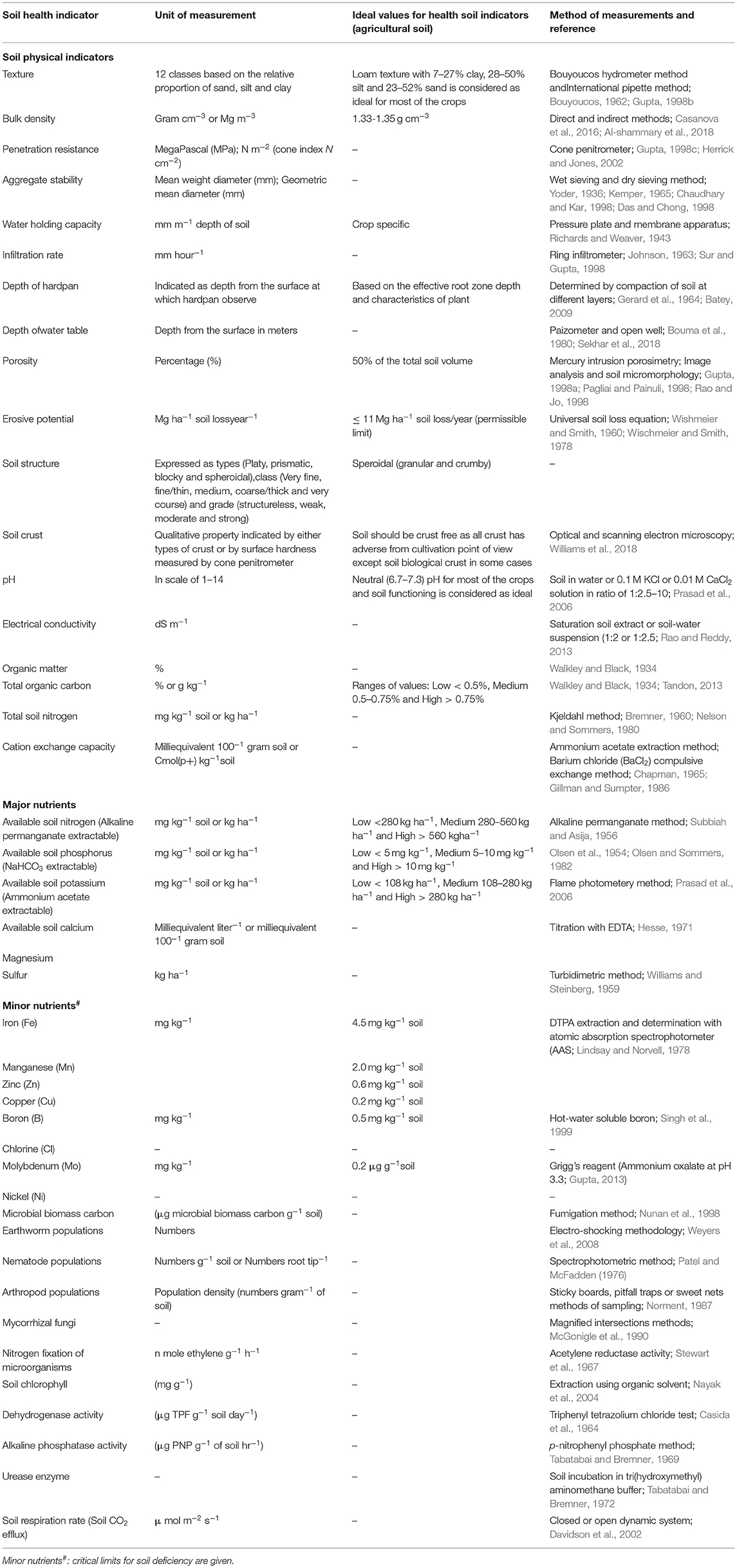
Table 3 . Soil health indicators and their measurements.
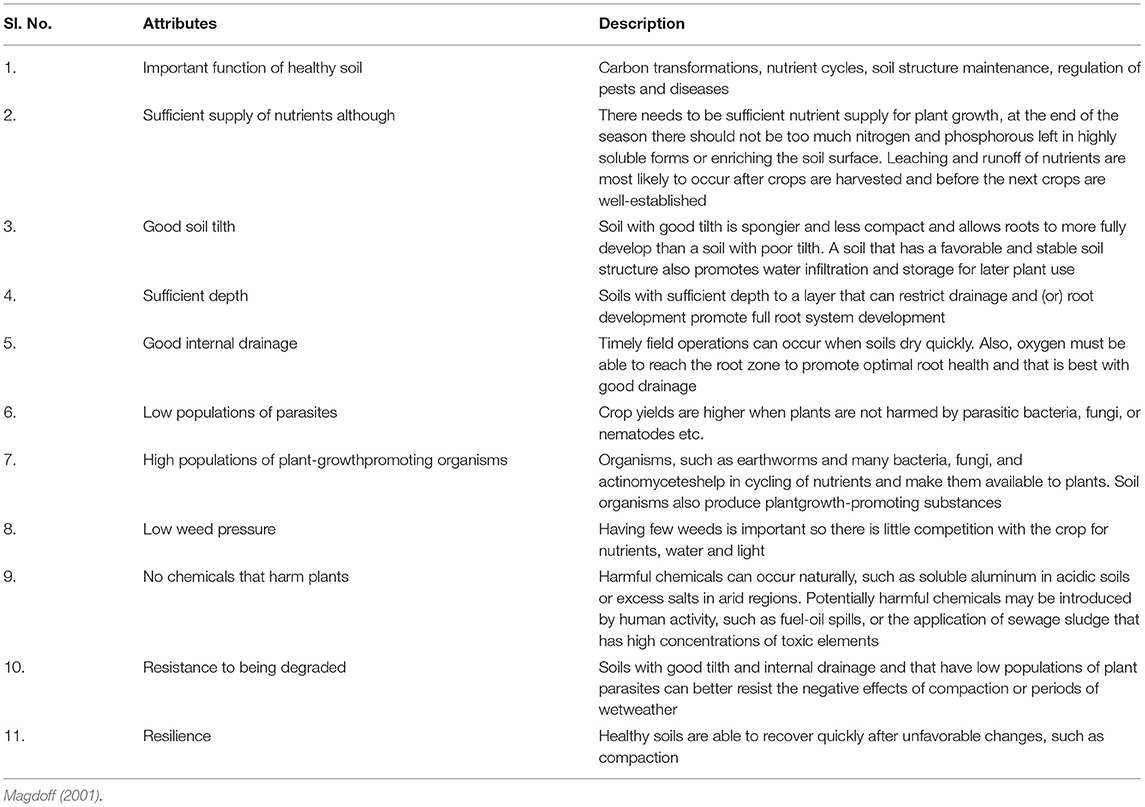
Table 4 . Characteristics of healthy soil.
Issues Related With Soil Health
Factors that cause deviation of healthy soil are issues related with soil health, and the level of impact of these factors on soil health decides their order of significance and make them a concern. Studies on these issues are important because of the following reasons:
• The health of soil has a direct influence on the sustainability of agro-ecosystems, as soil is a feeding substratum for all types of vegetation.
• Healthy soil will be more resilient to extreme weather phenomenon (drought, flood, etc.) and frequency of these phenomenons is expected to increase on the front of climate change ( Mirzabaev et al., 2019 ; Olsson et al., 2019 ).
• A healthy soil should provide more ecosystem services such as biogeochemical cycling of nutrients, enhanced microbial population, and diversity ( Costanza et al., 1997 ; Baveye et al., 2016 ).
• Maintaining soil health contributes to the sustainable development goals of the United Nations, such as alleviating poverty, reducing hunger, improving health, and promoting economic development ( Lal, 2016 ).
• Maintaining soil health is now almost important for enhancing crop productivity because of the occurrence of multi-nutritional deficiency in soil ( Rattan et al., 2009 ), increased soil degradation ( Bhattacharyya et al., 2015 ), and accumulation of harmful pesticide residues in soil that adversely affect soil microorganisms ( Meena et al., 2020 ).
• Maintaining soil health also contributes to carbon sequestration, as soil organic carbon is one of the most important criteria for soil health evaluation ( Lal, 2016 ).
• Intensification of agriculture with imbalance in the use of artificial resources and less attention on the potential of natural resources adversely affects soil health.
Stakeholders, researchers, and policy planners have shown an increased attention for soil health management as proved by an increased rate of the adoption of conservation agriculture ( Kassam et al., 2019 ), emphasis on organic farming, promotion of diversification in agriculture, development and adoption of land use classification ( USDA, 1961 ; Grose, 1999 ), and adoption of farming system-based approach rather than using cropping system alone. Policies/schemes such as soil health card schemes also address one or more soil health-related issues ( Wienhold et al., 2008 ; Anonymous, 2011 ; Islam et al., 2017 ; Reddy, 2017 ). Terms mainly used to describe degraded soil health are land degradation, soil degradation, soil desertification, and soil pollution. Land degradation is the loss of actual or potential productivity or utility as a result of natural or anthropogenic factors. It is a decline in land quality or a reduction in land productivity ( Eswaran et al., 2001 ); while IPCC ( Olsson et al., 2019 ) define land degradation as a native trend in land condition, caused by direct or indirect human-induced processes such as anthropogenic climate change, expressed as long-term reduction or loss in at least one of the following: biological productivity, ecological integrity, or value to humans. Soil degradation is considered as a subset of land degradation ( Olsson et al., 2019 ), which directly affects soil and is defined as a decline in the productivity of soil through adverse changes in nutrient status, soil organic matter, structural attributes, and concentrations of electrolytes and toxic chemicals ( Aulakh and Sidhu, 2015 ). The other term, soil desertification, is mainly related to the physical degradation of soil and is defined as land degradation in arid, semi-arid, and dry sub-humid areas, collectively known as drylands, resulting from many factors, such as human activities and climatic variations ( Mirzabaev et al., 2019 ). The term soil pollution was defined as the build-up of persistence toxic compounds, chemicals, salts, radioactive materials, or disease-causing agents in soils, which have an adverse effect on plant growth and animal health ( Okrent, 1999 ). In this section, issues of soil degradation are discussed separately in three headings viz . physical, chemical, and biological degradation of soil. This will help in addressing the wide variation in factors that needs to be taken into consideration while discussing the issues of soil degradation.
Soil Physical Degradation
Major processes that cause physical degradation in soil include water erosion, wind erosion, wave erosion, coastal erosion, soil crusting, compaction, and hardening ( Saha, 2003 ; Karlen and Rice, 2015 ). At the same time, agricultural practices that cause soil physical degradation include increased tillage intensity, inappropriate timing of tillage, aerobic-anaerobic cycles of soil moisture status in intensive cereal-based cropping systems (rice-wheat cropping system; Chauhan et al., 2012 ), lower addition of bulky organic manures, and removal of all dry matter produced, making soil devoid of vegetation. Soil physical degradation is mainly caused by either loss of soil from the area or modification of soil physical properties without any accountable loss in soil from the area.
Loss of Soil From the Area
Among the above-mentioned processes, soil erosion is the most prominent cause of soil physical degradation. At a global level, the estimated area affected by land degradation is 19.65 million km 2 ( Obalum et al., 2017 ); while in India, the estimated area affected by soil erosion is 31.5 to 166.1 million ha (m ha) ( Bhattacharyya et al., 2015 ) with total soil losses of 5,334 million tons year −1 (16.35 t ha −1 year −1 ) ( Dhruvanarayana and Babu, 1983 ; Aulakh and Sidhu, 2015 ). Bhattacharyya et al. (2015) reported that a 94-mha area is affected by water erosion and 9 mha by wind erosion; while Lal (2001) reported that the area affected by water erosion and wind erosion was 32.8 and 10.8 m ha, respectively. In the process of soil erosion, detachment and transportation of soil particles happen from one place to another. Dhruvanarayana and Babu (1983) reported that 29% of the total displaced soil is lost permanently to the sea. Agents causing soil erosion are water and wind; the erosion caused by the combined action of water and wind that prominently occurs along canals and river banks is called wave erosion. Factors that decide the rate of water erosion are rainfall characteristics (intensity, distribution, and frequency), soil erodibility, steepness and length of the slope, crop cultivation practices, special practices for erosion control, and the erosivity of an agent (water) that causes erosion. The relative significance of these factors are varied over time, and space dimension and soil erosion were calculated from these factors using the Universal Soil Loss Equation (USLE) given by Wishmeier and Smith (1960) ; Wischmeier and Smith (1978) . Factors affecting wind erosion are soil cloddiness, surface cover, surface roughness, soil textural class, local wind factor, wind width factor, wind direction, and wind barrier, while the functional relationship of these factors and the calculation of soil losses by wind erosion were given by Woodruff and Siddoway (1965) . Singh et al. (1992) made an attempt to locate e iso-erosion lines on the map of India and quantify the rate of soil erosion in different areas ( Table 5 ). The loss of soil due to erosion, according to them, ranges from 5 to 80 Mg ha −1 year −1 .
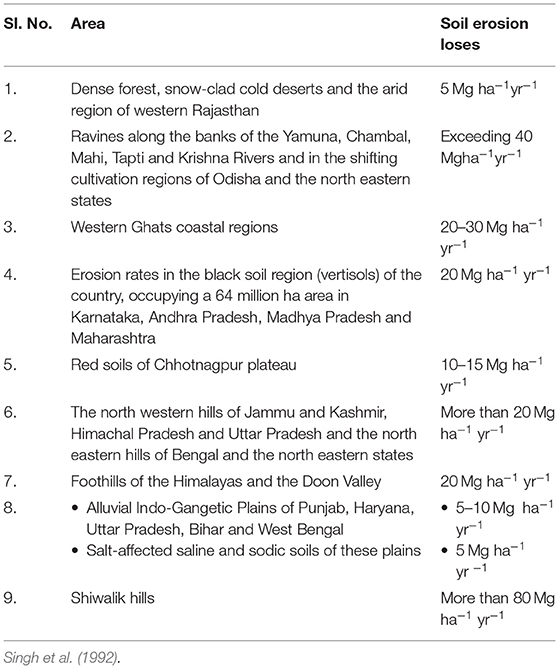
Table 5 . Soil erosion losses in different parts of India.
Modification of Soil Physical Properties
Crusting and compaction : Soil crusting is a surface phenomenon in which a hard thin layer of soil is formed on the surface of the soil. Valentin and Brasson (1997) defined soil crusting as the forming processes and the consequences of a thin layer at the soil surface with reduced porosity and high penetration resistance. In the formation of a soil crust, soil aggregates get broken down and the soil becomes more compact with less porosity ( Manyevere et al., 2015 ). The properties of the soil are modified due to crust formation leading to (a) initiation or increase rate of erosion; (b) adverse effect on plant germination and crop growth and; (c) modification of water entry and movement. Another term used to describe soil crusting is surface sealing, and Morin (1993) defined surface sealing as the orientation and packing of dispersed soil particles that are disintegrated from soil aggregates because of the impact of rain drops. The types of crusts are structural, depositional, erosion, chemical, and biological ( Valentin and Brasson, 1992 ; Morin, 1993 ; Pagliai and Stoops, 2010 ; Williams et al., 2018 ), and are defined as follows ( Valentin and Brasson, 1992 ):
• Structural crusts: These are crusts that formed because of the in-situ arrangement of soil particles/aggregates without any lateral movement, and based on their morphology and formation process they are named as slaking crust, infiltration crust, coalescing crust, and sieving crust. The USDA natural resource conservation service defined soil structural crusts as relatively thin, dense, somewhat continuous layers of non-aggregated soil particles on the surface of tilled and exposed soils.
• Depositional crusts: In this type of crust, an external material is involved, and they are formed when the external material is carried by the flow of water settled after infiltration and evaporation of water.
• Erosion crusts: Erosion crusts consist of only a rigid, thin, and smooth surface layer enriched in fine particles ( Valentin and Brasson, 1992 ).
• Chemical crusts: These are a type of crust formed because of the precipitation of chemicals or salts with surface sealing/hardening properties.
• Biological crusts: Formed because of colonization of different microorganisms forming community all around soil particles/aggregates. The distinctive characteristic of this type of crust is that it protects soil from erosion, and it contributes to soil organic carbon and nutrient accumulation ( Belnap, 2005 ).
Mechanisms of crust formation
• Mechanical destruction of soil surface aggregates by raindrop impact ( Le Bissonnais, 1996 ).
• Leaching of fine particles and their subsequent deposition in underlying pores.
• Compaction of soil surface to form a thin film that restricts both further entry of water and movements of fine particles in soil pores.
• Chemical dispersion of clay particles.
• Soil degradation due to intensive land use.
The formation of soil crust contributes to soil erosion and, ultimately, to soil degradation in one of the following ways:
• decreased hydraulic conductivity and infiltration rate ( Nciizah and Wakindiki, 2015 );
• loosening of soil aggregates, decrease in aggregate stability and, ultimately, disturbance in soil structure;
• increases the rate of runoff;
• and the deposition of eroded materials, which causes surface sealing.
Major soil and climatic conditions that promote soil crust formation:
• medium-textured soil;
• predominance of smectite, illite, and micaceous minerals;
• high exchangeable sodium percentage and low organic matter;
• in arid and semi-arid regions but also commonly occur in cultivated soils in other climates ( Williams et al., 2018 );
• and water content during a rainfall event.
Soil compaction : It is the state of land in which soil porosity decrease is accompanied by an increase in bulk density. Major reasons for soil compaction include: continuous tillage at same soil depths, higher traffic, continuous use of heavy machinery, tillage practices at improper moisture in the soil, and decreased addition of organic amendments. Soil compaction affects soil health similarly as that of soil crust in surface layer; while below soil surface layers, decreased porosity, increased bulk density, reduction in downward and lateral movements of water are the other important effects of soil compaction that negatively affect soil health parameters.
Soil desertification: Soil desertification is another type of land degradation whose impact not only limits soil health assessment but is also important from the point of view of climate change, food security, and economics ( Anonymous, 2018b ; Mirzabaev et al., 2019 ; Wijitkosum, 2020 ). The organization of a conference on desertification by the United Nations in 1977, the constitution of the United Nations Convention to Combat Desertification (UNCCD) in 1994, and soil desertification place in sustainable development goal number 15 also highlight the severity of the problem. The term desertification was used for the first time in a broader sense by Aubreville in 1949 (after Luvauden in 1927). It is defined as the type of land degradation in arid, semi-arid, or dry sub-humid areas caused by human activities and climatic variation; while Sterk and Stoorvogel (2020) considered it as land degradation in dry land areas. The conference on desertification by the United Nations described the phenomenon of desertification as “the diminution or destruction of the biological potential of the land, which can lead ultimately to desert-like conditions. It is an aspect of the widespread deterioration of ecosystems and has diminished or destroyed the biological potential (plant and animal production), for multiple use purposes at a time when increased productivity is needed to support growing populations in quest of development.” The most recent estimate ( Le et al., 2014 ) cited in Sterk and Stoorvogel (2020) indicated that, a 1,470-million-ha area, which is 29% of the total dry land, is affected by one or the other types of desertification; while Sterk and Stoorvogel (2020) had an opinion that a 1,036-million-ha area, which is 20.5% of total dry land, is affected by some form of soil degradation. In India, an 82.34-million-ha area ( Anonymous, 2018b ) is affected by desertification and includes all areas affected by one or the other types of land degradation. The extent of desertification is mainly judged based on the world map of the status of human-induced soil degradation, which was developed by the Global Assessment of Soil Degradation project (GLASOD) and based on expert knowledge of soil degradation processes and their spread in a large number of countries. According to a United Nations environmental program ( Middleton and Thomas, 1997 ), desertification is the outcome of the following activities:
• climatic factors (temperature, rainfall, etc.),
• overgrazing,
• deforestation,
• agricultural activities,
• overexploitation of vegetation for domestic use,
• and bio-industrial activities.
Desertification contributes to the degradation of soil health through the following:
• rapid loss of vegetative cover on the soil surface and decrease in soil organic carbon;
• facilitation of the movement of soil/sand from one place to another, leading to expansion of desert;
• increased susceptibility of soil to wind and water erosion;
• adverse effect on the microbial population and diversity in the soil;
• and variation in soil surface relief and topography due to physical movement of soil.
Waterlogging: It is the state of soil moisture at which soil is saturated with water (all soil pores filled with water) and also used to indicate raising groundwater to the surface level ( Awad and El Fakharany, 2020 ). In India, waterlogging is one of the important reasons for soil degradation, and the area affected by water logging is 11.6 million ha ( Roy Chowdhury et al., 2018 ). The type may be surface waterlogging in which excess water is seen above the soil surface, or a subsurface type in which excess water remains below the soil surface. Soil characteristics, climate (rainfall), and plant cover have a profound influence on waterlogging. The areas and conditions in which waterlogging occurs are listed as follows:
• areas with heavy rainfall (the intensity of rainfall plays a major role);
• over irrigation mainly found in canal command areas in India;
• areas along river banks because of expansion of agricultural land up to riverbanks (mainly during flood situation);
• low elevated land where the collection of water causes waterlogging;
• areas around water reservoirs because of seepage of water;
• low infiltration rate and hardpan formation, and the presence of chemical salts, such as sodium and its compound aggravate the problem of waterlogging.
Water logging affects soil health adversely in one of the following ways:
• disturbing soil physical health through reduced aeration, structural stability, and lowering down of soil temperature;
• reducing soil oxygen level, anaerobically decomposing soil organic matter, and accumulating toxic gases and other products of decomposition;
• Change in soil reaction along with losses in soil nutrients through leaching and overland flow.
• changing soil microbial population from aerobic to anaerobic or facultative aerobic, which leads to adverse effects on several microbial processes and biogeochemical cycling of nutrients;
• and unfavorably affecting soil tillage properties and making soil unsuitable for cultivation of most crops.
Soil Chemical Degradation
Soil chemical health gets more attention from both researchers and stakeholders because of its most direct and significant influence on agricultural productivity, growing need for external addition of amendments and nutrient sources, and the profound influence of soil chemical properties on modification of soil biological and physical health. The chemical degradation of soil is discussed under the following subsections:
• Reduction in soil carbon;
• Changes in soil reaction (acidification and sodification);
• Modification of soil mineral nutrient status (nutrient imbalance, multi-nutrient deficiency);
• Accumulation of toxic compound (agrochemicals);
• and Soil pollution.

Reduction in Soil Organic Matter
Soil is an important carbon pool at the global level, with 1,895–2,530 Pg carbon, which is two times as that of carbon present in the atmosphere and three times as that of biotic carbon pool. Out of total carbon in soil, 695–930 Pg is inorganic and 1,200–1,600 Pg is organic in nature ( Sahoo et al., 2019 ). Among these two fractions, organic carbon is more important from a soil health point of view, and studies on factors that have a significant impact on soil organic carbon are also important considering the significant decrease in soil organic carbon in Indian soil ( Reddy, 2017 ) and in world agricultural production systems ( Song et al., 2005 ; Grace et al., 2006 ; Gardi et al., 2016 ; Wiesmeier et al., 2016 ; Blecourt et al., 2019 ). At the same time, as soil organic carbon serves as a source and storehouse of plant nutrients, it has a great role in crop production improvement along with its significance in soil health. The functions of soil organic carbon in soil health, crop productivity, and ecosystem services are given in Table 6 .
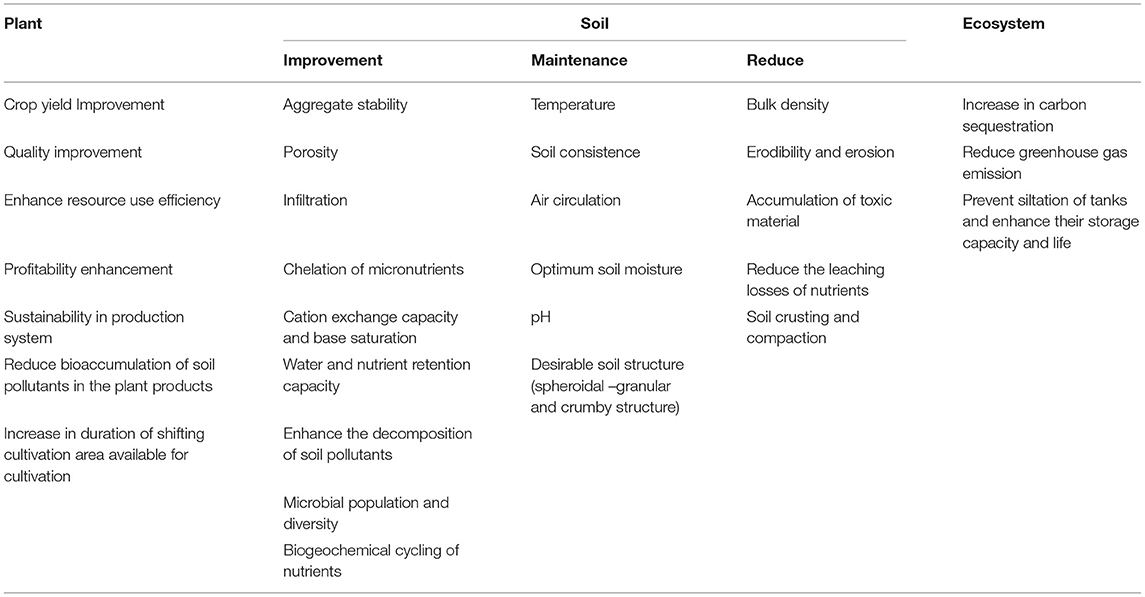
Table 6 . Functions of soil organic carbon.
Factors affecting soil organic carbon status: Wide variations in land use changes and crop husbandry across agricultural production systems, and sensitivity of soil organic carbon to these changes, are responsible for significant variations in the organic carbon content of soil. The soil organic carbon content was affected in three different ways viz ., decrease in soil organic carbon, improvement due to fertility addition, and changes due to crop cultivation. The decrease in soil organic carbon is mainly due to land use changes caused by tillage in arable crops and types of crops grown if considered at the agro-ecosystem level. The impact of tillage on soil organic carbon can be seen by comparing the plow-based conventional tillage system, which is widely followed all over the world, with conservation tillage, which is currently getting momentum because of its several positive impacts on soil, plant, and water ( Table 7 ). In fact, the adverse effect of the conventional plow-based tillage system on soil health was one of the reasons for the origin of conservation tillage. The breaking of soil aggregates, exposure of soil organic carbon to different types of degradation and decomposition, complete removal of dry matter produced by crops, burning of crop residue, dependence on inorganic fertilizers, mono-cropping of few crops, and less addition of organic nutrient sources are factors that intensify the decrease in soil organic carbon; while three principles of conservation agriculture ( Kassam et al., 2019 ) counteract these adverse effects of conventional tillage. At the same time, the availability of a large array of selective herbicides, availability of machinery for sowing and subsoil placement of fertilizer, and increased interest at research and development front in the modification of nutrient release patterns from crop residues through different ways ( Singh et al., 2009b ; Swarnalakshmi et al., 2013 ; Choudhary et al., 2016 ; Gangaiah and Prasad Babu, 2016 ) also promote conservation tillage-based agriculture.
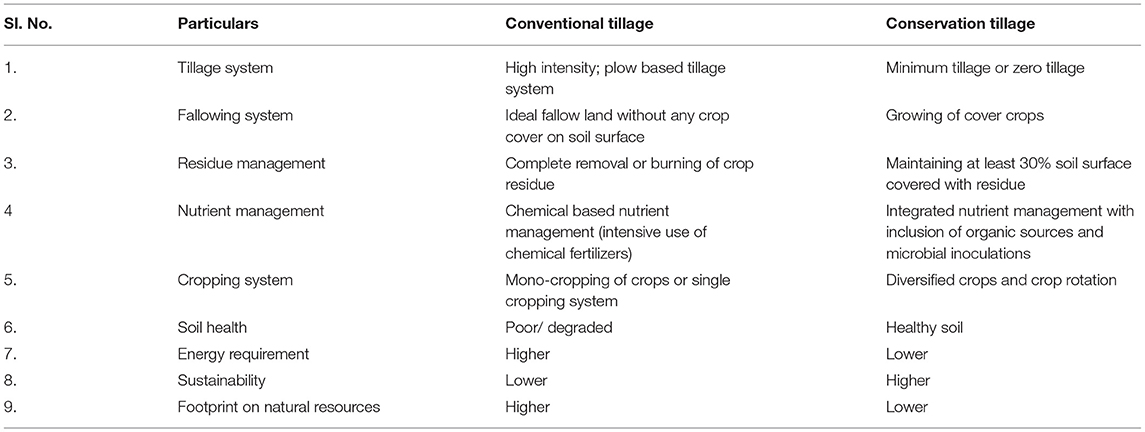
Table 7 . Differences between conventional tillage and conservation tillage.
In regard to the effect of fertility addition and crop cultivation on soil organic carbon, the results from several long-term experiments will be the proof for the same ( Reddy et al., 2017 ). Improvement in soil organic carbon due to the addition of an optimum dose of chemical fertilizers and the combination of chemical fertilizers with organic sources, and a decrease in soil organic carbon over the years due to cultivation of crops ( Mandal et al., 2007 ), are the major findings of long-term experiments in India. The variation in soil organic carbon in permanent agriculture has a different pattern compared with the growing of arable crops ( Bernardi et al., 2007 ; Ganeshamurthy et al., 2020 ) because of variation in the frequency of land disturbances. Along with this, the variation in carbon sequestration potential of crops ( Ghosh et al., 2006 ; Brar et al., 2015 ) is another important factor affecting soil organic carbon in cultivated areas.
In land use change, bringing the marginal land under cultivation has an adverse effect on soil organic carbon; while the utilization of degraded land for agro-forestry or energy plantation will successfully maintain or enhance soil organic carbon. Another land use change that significantly affects soil organic carbon content and most prominent innorth east India is shifting cultivation ( Bhuyan, 2019 ). The clearing of natural vegetation and bringing the land under cultivation reduce soil organic carbon ( Sharma et al., 2019 ).
Change in Soil Reactions (Acidification and Sodification)
The study on soil reactions for their effect on soil chemical health is also important because of the following reasons:
• Mineral nutrient availability is affected by soil reactions.
• Soil properties such as cation exchange capacity, base saturation, chelation of micronutrients, and anion exchange capacity, are responsible for the retention and movement of nutrients in the soil. These properties change with a change in soil reaction.
• Soil physical properties such as aggregation and erodibility are also affected by a change in soil reactions. Mineral elements such as sodium have a significant impact on soil aggregation and their presence in soil is controlled, to a large extent, by soil reactions.
• The relative proportion of different forms of mineral nutrients present in soil and inter-convergence is affected by soil reactions.
• The biogeochemical cycling of nutrients and the role of microorganisms in it are also modified with changes in soil reactions.
A soil reaction near neutral pH is mostly suitable for the cultivation of crops and different properties of soil; while an abnormal change in soil reactions affects soil chemical health. Changes in soil reactions due to human-induced changes in soil, water, and plant are observed at a very slow rate because of buffering capacity of soil and predominance of soil mineral matters (occupying 45% of the total soil volume) in deciding soil reactions. Environmental factors that cause changes in soil reactions include:
• weather factors, mainly rainfall patterns and temperature (causes leaching and erosion of soil mineral and organic matter);
• climatic factors intensify weathering which creates changes in soil parent materials;
• and topographical factors, topography of surface, and presence or absence of vegetation on the soil surface.
Human-induced changes in soil pH are mainly caused by the application of amendments for improving soil properties (liming or gypsum application), fertility addition through organic and inorganic sources of nutrients, and changes in land use ( Mishra et al., 2006b ). The effect of both the natural- and human-induced factors on the pH of the soil is conditioned by time. Major reactions that make soil chemically unfit for agriculture include the following:
• Acidification : It is the process of decreasing soil pH to such an extent that the soil becomes unfit for cultivation, and it is caused by both natural- and human-induced processes. Major natural processes causing acidification include acid rain, application of acid-forming fertilizers, mineralization of organic matter, nutrient uptake by roots, root exudates, and nitrogen fixation by legumes ( Goulding, 2016 ). Soil acidification adversely affects soil health by changing the modification of nutrient availability, soil microbial population, and toxicity to the roots of plants due to increased levels of one or more mineral element concentrations. The area under acidic soil conditions in India is 17.9 million ha ( Anonymous, 2016 ); while in the world 3,950 mha of arable land is affected by soil acidity ( Bian et al., 2013 ), indicating the severity of the problem.
• Sodification : This phenomenon is the opposite of soil acidification, because soil pH is increased by the predominance of carbonates and bicarbonates of sodium. The presence of sodium in soil significantly modifies the soil properties, thereby affecting soil health and productive potential. The major changes in soil due to sodification include dispersion of soil aggregates leading to poor soil physical condition, reduced hydraulic conductivity and infiltration rate, changes in nutrient availability, and toxicity of higher concentration of sodium to plant roots.
Modification of Soil Mineral Nutrient Status (Nutrient Imbalance, Multi-Nutrient Deficiency, and Nutrient Mining)
Among the major input additions in present-day agriculture, nutrient application plays an important role and is mainly due to the increased response of crops to nutrient application, crop and/or cropping system intensification in special and temporal dimensions to feed burgeoning population, and decrease in the level of soil nutrient status. The modification of soil nutritional status is mainly expressed as nutrient imbalance, multi-nutritional deficiency, and nutrient mining. The imbalance arises because of differential nutrient uptake and fertility addition, which does not match the plant uptake; while the present status of multi-nutritional deficiency was increased because of the addition of only primary nutrients (especially N and P) with complete dependence on soil nutrient reserves for other nutrients. Nutrient mining is another term used to indicate the negative balance between nutrient addition and nutrient removed by crops. At present, Indian soils are at negative balance of 8 to 10 million tons per year ( NAAS, 2018 ); while Jones et al. (2013) and Henao and Baanante (2006) reported nutrient mining practices at the global level. The significance of soil mineral nutrient status with respect to soil health and overall agricultural productivity can be explained using the following points:
• increase in the number of nutrients showing deficiency in cultivated soil;
• extent of negative balance of nutrients in the soil;
• responsiveness of crops to the application of nutrients;
• possibility of reducing nutrient mining by utilizing crop by-products as a nutrient source and avoiding their ineffective use such as in-situ burning;
• share and role of organic material addition in meeting the nutrient need of agriculture;
• role of microbes in enhancing the nutritional status of soil;
• long-term effect of application of recommended rate of nutrients on soil nutrient status;
• short and long-term impact of nutrient mining on crop productivity and economics;
• effect of changing soil nutrient supplying capacity due to change in soil organic carbon in arable soils;
• effect of imbalance in the use of chemical fertilizers on soil nutritional status;
• lack of attention for soil and water conservation practices leading to loss of fertile top soil layer rich in plant nutrients;
• and soil fertility changes due to cultivation of crops on marginal and degraded land as well as intensive cereal-based crops/cropping systems with replacement of fertility restorer crops.
Accumulation of Toxic Compound (Agrochemicals)
This is the major source of toxic compound which get accumulated in soil thereby affect the soil health. The use of agrochemicals for plant protection and weed management leads to considerable increase in accumulation of toxic compound in soil. This can be seen from an increase in the use of agro-chemicals from 39,773 to 52,980 metric tons of technical grade material ( Bhardwaj and Sharma, 2013 ; Indira Devi et al., 2017 ). Even with this significant increase in agrochemical consumption, per hectare consumption in India is 291 g ha −1 , which is far lesser than the consumption in developed countries ( Indira Devi et al., 2017 ). The use of pesticides in Japan, China, and Mexico is 18.94, 10.45, and 7.87 kg ha −1 , respectively ( Zhang, 2018 ). Along with that, excessive use of chemical salts to provide nutrition is the other source of toxic compounds. Some organic sources of crop nutrition, such as sewage and sludge and night soil, are also reported to contain a high amount of heavy metals ( Walia and Goyal, 2010 ; Saha et al., 2018 ), causing adverse effects on soil health. The reason for the increasing contribution of agrochemicals to soil chemical degradation is their unregulated and uncontrolled use ( Bhardwaj and Sharma, 2013 ) and lack of proper knowledge and awareness on the use of agrochemicals. The major adverse effects of using agrochemicals on soil health include: (i) adverse effect on the population dynamics of soil microflora and microfauna, (ii) affecting the rate of biogeochemical cycling of nutrients, and (iii) adverse effect on the growth of plants along with bioaccumulation of agrochemicals in plant and animals. Considering the role of agrochemicals in crop production and, overall, in agriculture, their complete elimination is difficult, but following regulations and recommendations in their use can be helpful in minimizing their build-up to an extent that they are causing adverse effects on soil health.
Soil Pollution
Soil pollution in cultivated fields is another emerging problem that is considered as a major outcome of modern agrochemical-based agriculture and lack of accounting of footprint of agricultural activities. Soil pollution is defined as a physical, chemical, biological, or radiological modification of the surface layer of the crust of the earth by the accumulation of a large quantity of natural materials or occurrence of new synthetic materials that disturb the composition of the soil, influence the natural balance of the ecological system, and disable the purification process (self-cleaning) of the soil ( Backovic, 2008 ; Ashraf et al., 2014 ). The causes of soil pollution in agricultural land are:
• inappropriate use of chemical fertilizers especially phosphatic fertilizers, herbicides, and use of agrochemicals for insect-pest and diseases management;
• application of materials rich in pollutants and use of industrial waste;
• use of inferior plastic films;
• use of polluted water for irrigation;
• use of polluted area for agriculture or growing of crops along a city landfill;
• improper disposal of industrial wastes;
• seepage from landfills and percolation of pollutants along with infiltrating water;
• longer persistence of biochemical compounds in wastes and lack of soil flora and fauna for decomposition of agrochemicals;
• and neglecting the significance of soil pollution remediation measures.
These sources have varied effects on soil health and ultimately on agricultural productivity. The effects of soil pollutions are as follows:
• Soil properties such as porosity, base saturation, soil reaction, soil salinity, and nutrient toxicity are affected because of soil pollution ( Backovic, 2008 ).
• Soil pollution caused by industrial waste or sewage-sludge may lead to the accumulation of heavy metals that may enter in the food web, leading to bioaccumulation of these heavy metals in animals or human beings, leading to several health hazards ( Khan et al., 2015 ). The identification of adverse effects of such pollutants on human health sometimes becomes difficult, as they are seen after long exposure and continue across generation.
• The pollutants present in soil may escape and add to groundwater because of leaching or enter into above-ground water reservoirs, thereby causing pollution in these water bodies. This makes the water unsafe for use and also harms aquatic life ( Khanna and Gupta, 2018 ).
• Pollutants that accumulate in soil up to the toxic level may affect the germination and growth of the next crop in succession.
• Soil pollution may adversely affect the population dynamics of soil microorganisms and thereby nutrient cycling.
• In extreme cases, they make soil unfit for normal crop cultivation.
• Pollutants such as heavy metals are non-degradable by any biological or physical means and therefore remain in soil over longer duration ( Selvi et al., 2019 ).
• Heavy metal pollution is one of the hurdles of direct use of nutrient-containing minerals in agriculture and more especially in organic farming ( Mortvedt, 1995 ).
Soil Biological Degradation
The biological properties of soil are the last to get attention. However, they started getting attention when their normal activities and functioning became affected significantly by modern agricultural practices. Soil biological degradation is defined as the impairment or elimination of one or more significant populations of microorganisms in the soil, often with resulting changes in biochemical processing within the associated ecosystem ( Sims, 1990 ). At present, considering their significant role in different soil processes and functional activities, soil microbial properties are studied as rhizosphere dynamics ( Kumar et al., 2013 ) and soil genomics ( Singh et al., 2009a ) level. Soil biological properties that can be used to judge the biologically degraded soil ( Bedano et al., 2011 ; Lehman et al., 2015 ), as given by Mishra and Dhar (2004) , are listed below:
• abnormality in microbial community diversity indicated by viable count (colony forming unit);
• reduction in either species richness or evenness of allocation of individuals among various species or both the above-mentioned characteristics;
• adversely affected major soil processes such as soil respiration, different enzyme activities, nutrient cycling, and degradation of organic compounds;
• symptoms of accumulation of toxic compounds in the soil due to their reduced rate of decomposition;
• and an increase in the population of undesirable microorganisms/pathogens causing diseases or serve as a vector for the transfer of different diseases.
The major difficulties in determining soil biological health and evaluating the indicators of soil biological health mentioned by Brackin et al. (2017) are as follows:
• complex relationships of soil microbial life with soil properties and crop plants;
• highly dynamic and sensitive to changes in soil management (such as tillage and amendment addition, etc.);
• difficulty in the identification and quantification of exact soil microbial life affecting soil health because of their very large diversity ( Nielsen et al., 2016 );
• and use in short-term evaluation because of their higher sensitivity to changes in soil conditions ( Obalum et al., 2017 ).
Soil Ecosystem Services
There are several types of degradation processes acting side by side as discussed in previous sections (Soil Physical Degradation to Soil Ecosystem Services) due to continuous human interferences. All these processes lead to drastic changes in ecosystem services provided by the soil, as listed below:
• reduction in nutrient-supplying capacity of soil with a net negative nutrient balance;
• reduction in the rate of decomposition of soil pollutants due to biological degradation of soil;
• reduction in capacity to act as net carbon sinks because of continuous reduction in soil organic carbon content in most of the agricultural land;
• increasing and decreasing the population diversity of undesirable microbes (pathogen) and useful microbes in the soil;
• increase in areas under salt-affected soil conditions, thereby reducing their productivity potential;
• and reduction in productive potential and future carrying capacity of soil due to the above-mentioned five points.
At the same time, studies on soil ecosystem services are important because of the following points:
• increased level of the human footprint on natural resources;
• faster rate of degradation of natural resources;
• increasing concerns of climate change and its effect on soil ecosystem services;
• increase in the human and animal population, which increases the burden on limited natural resource;
• and economic and global model of development adopted by the world, with less consideration to ecological aspects.
Effect of Soil Degradation on Plant Growth
Considering the level of degradation of soil as discussed in previous sections, the effect of land degradation on soil productivity needs to be quantified. In this section, attempts were made to review the effect of land degradation on plant growth using the study conducted by different researchers from different parts of the world.
Productivity and Profitability
The effect of several land degradation problems on crop productivity can be studied either by accounting for the losses in natural resources due to different processes at the global level, or from the reduced productive potential of degraded soil. In the European Union, Panagos et al. (2018) used microeconomics models and reported that 12 m ha of agricultural areas in the European Union have degraded soil. This led to economic losses in the agricultural sector to be close to €300 million and loss in GDP to be about €155 million. In Senegal, Sonneveld et al. (2016) reported that severe types of land degradation were associated with a decline in crop productivity. Pimentel and Burgess (2013) also reported a significant impact of soil erosion on food production. In the Canadian prairies, Cann et al. (1992) showed a compilation of the significant impacts of soil degradation on different crop yields. In India, Bhattacharyya et al. (2015) reported that the total cost of land degradation varies from US$1,037.94 to 6,191.81 million [1 US dollar ($) = 72.45 Indian rupee (₹)] per annum with the highest cost of land degradation due to soil erosion. This leads to a loss in crop production, which varies between US$93,305 and 4,982.71 million per annum. Zingore et al. (2015) reported different soil quality constraints for crop production in sub-Saharan Africa, and these problems, according to their significance in terms of area affected, are aluminum toxicity > low cation exchange capacity> soil erosion > high phosphorus fixation > vertic properties > salinity > sodicity. They reported that in sub-Saharan Africa the total crop production area affected by these soil constraints was 23 billion ha. These constraints are an indication of degraded soil, and significantly reduce the productivity of the soil. In Australia, Koch et al. (2015 ) reported the significance of soil security in achieving food security and provision of ecosystem services. Mythili and Goedecke (2016) used a total economic value approach for the calculation of the cost of land degradation and reported that the annual cost of land degradation in India in 2009 was US $5,152.46 million. This indicates that land degradation puts a significant economic footprint along with a footprint on natural resources.
Along with these impacts of soil degradation at a large landscape, the effect of different soil ill health on crop productivity and economics, as well as the response of crop grown in such soil to various amendments reported by different authors are summarized in Table 8 . The significant contribution of soil degradation to the reduction in crop productivity can be judged from the accumulation of a large number of such studies ( Frye et al., 1982 ; Lal and Moldenhauer, 1987 ; Pierce and Lal, 1994 ; Mantel and Van Engelen, 1997 ; Wiebe, 2003 ; Rickson et al., 2015 ). These different studies showed that soil physical and chemical degradation had a significant and negative impact on soil health. Along with it, the adverse effect of soil biological degradation was also reported ( Song et al., 2017 ) showing a reduction in the germination of different grasses due to the formation of cyanobacteria-dominated crust.
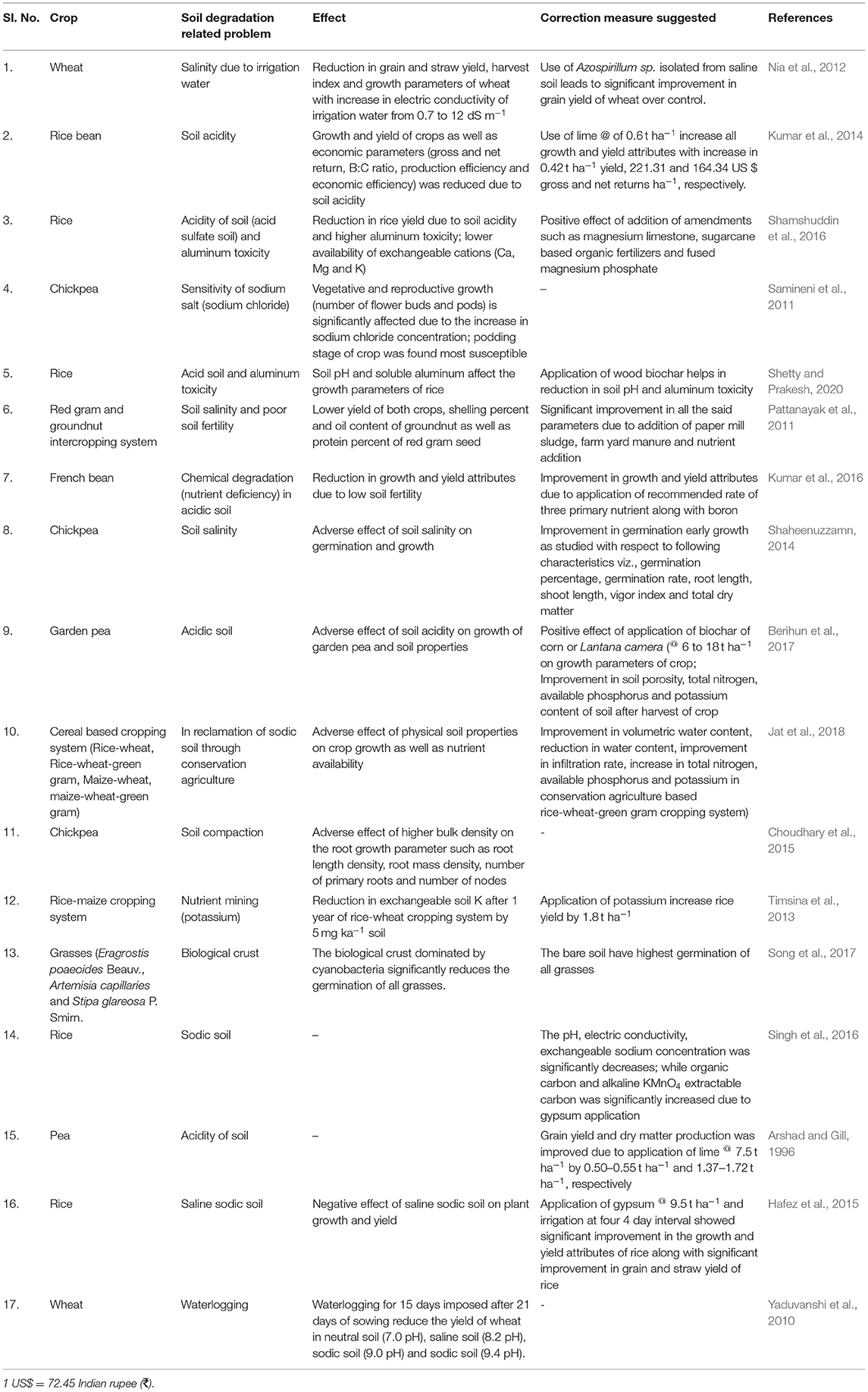
Table 8 . Effects of soil degradation on crop growth and productivity.
Resource Use Efficiency
Soil salinity is one of the most important problems affecting soil health in irrigation command areas. In saline soil, the amount of water required is higher than the water required for raising crops on normal soil in order to maintain salt balance in root zone depth, and because of that, water productivity is lower in saline soil. In Iran, various options for improvement in water productivity under saline soil conditions were reported by Heydari (2019) . He showed that optimum border irrigation and basin irrigation had higher water productivity (1.36 and 1.04 kgm −3 ) over the traditionally followed basin irrigation method. The salinity of irrigation water is also an important problem that leads to the build-up of soil salinity. Pressurized irrigation systems such as drip irrigation are reported to be most effective in improving water use efficiency and productivity; while the use of saline water for irrigation drip systems is a debatable issue due to root zone accumulation of salt and functioning of drip systems (clogging). Tingwu et al. (2003) showed that, use of saline water through drip irrigation on soil with ≤ 75% silt once every 2 days, at 60% of the Chinese pan evaporation had significantly higher yield and quality of watermelon over control even though water use efficiency in control (39.2 kg m −3 ) was significantly higher than treatment with 60% of the Chinese pan evaporation (21.45 kg m −3 ). They also reported that an increase in soil salinity build up averaged over soil profile in irrigation at 60% of the Chinese pan evaporation was very small over original soil salinity. Singh et al. (2018) reported that the application of irrigation water through a sprinkler or low energy water application each at 2 days interval with 4 cm depth at each irrigation significantly improved the water productivity and energy productivity over a surface method of irrigation with a similar level of rice grain yield in all irrigation systems. This finding again reports the successful use of a micro-irrigation system in problematic soil.
Nutrient use efficiency is another major challenge on the front of low nutrient use efficiency of major nutrients and reduction in the partial factor productivity of major nutrients due to multi-nutritional deficiency. Degradation of soil is one of the important reasons for the reduction in nutrient use efficiency and the need of higher fertilization. This can be clear from increasing the number of nutrients showing response to application ( Rattan et al., 2009 ), the status of soil degradation ( Bhattacharyya et al., 2015 ), and increase in the area showing the deficiency of secondary nutrients such as sulfur and micronutrients viz. Zn and Fe ( Tandon, 2013 ). Therefore, the application of amendments for soil improvement may contribute to nutrient use efficiency. Murtaza et al. (2017) reported a significant variation in nitrogen use efficiency in saline-sodic soil in a rice-wheat cropping system. They found that the application of 100 kg N ha −1 with 50% soil gypsum requirement recorded the highest partial factor productivity; and that the application of 130 kg N ha −1 and 100% soil gypsum requirement had the highest agronomic use efficiency of nitrogen in both rice and wheat. Yaduvanshi (2003) reported the positive effect of green manure application and farm yard manure on nitrogen and phosphorus recovery in reclaimed saline sodic soil in a rice-wheat cropping system. They reported that the addition of green manure of Sesbania @ 4.2 t ha −1 with 60 kg N, 13 kg P, and 21 kg K ha −1 had significantly improved N recovery; and that application of Sesbania @ 4.2 t ha −1 with 120 kg N, 26 kg P, and 42 kg K ha −1 had recovery efficiency of 52.8% in wheat. In another study, Barbieri et al. (2006) reported that out of the total nitrogen applied in tall wheatgrass ( Elytrigia elongate ), recovery efficiency was 23–41% in the 1st year and 67–69% in the second year in sodic soil. They suggested the split application of nitrogen and the use of nitrogen sources other than urea as a strategy to reduce losses. At the same time, the response of different treatments for the correction of soil degradation problems in terms of improving nutrient use efficiency and water use efficiency is mentioned in Table 9 . The significance of soil biological degradation in terms of increasing the population of disease-causing pathogens is significantly reducing the efficiency of different resources through their influence on crop growth and yield. Oerke (2006) reported that in the world, crop yield loss due to all major pest and diseases, such as weeds, for wheat, rice, maize, potato, soybean, and cotton was 28.2, 37.4, 31.2, 40.3, 26.3, and 28.8%, respectively, from 2001 to 2003. The loss due to insect–pest in India for cotton, rice, oilseed, pulses, groundnut, and wheat were 30, 25, 20, 15, 15, and 5%, respectively, out of their total production ( Dhaliwal et al., 2010 ). The loss in yield ultimately remains as the natural and artificial resources applied unutilized, which may be lost by one or other pathways thereby reducing their efficiency as well as may cause pollution or degradation of soil and other natural resources.
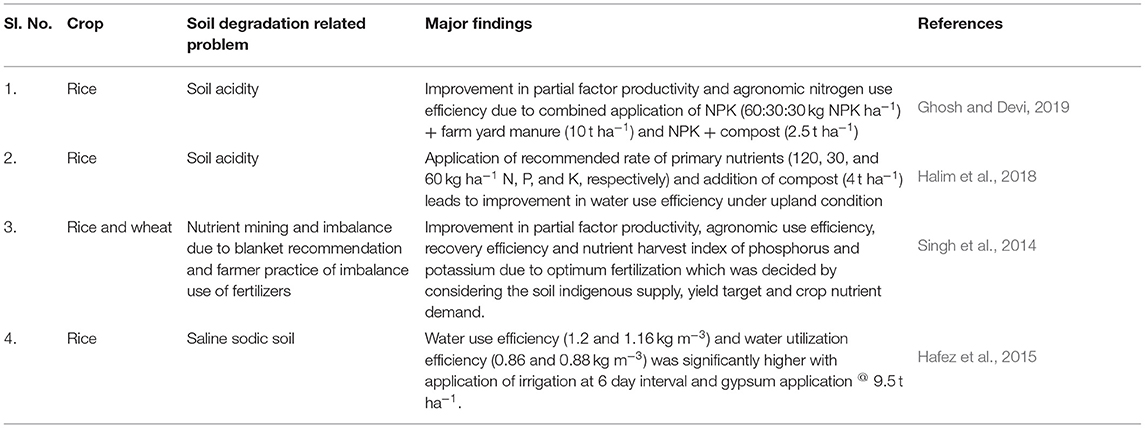
Table 9 . Effects of soil degradation on nutrient and water use efficiency.
Novel Agronomic and Innovative Soil Management Approaches for Improving Soil Health
Diversification of nutrient sources.
The nutrient need of plant is catered by soil inherent supply or externally applied plant nutrients through organic sources, inorganic sources, and microbial inoculants. Along with the supply of nutrients, these externally applied sources of plant nutrition had varied impacts on soil properties, and may be positive or negative. The monotonous use of any one source (especially chemical fertilizers) over a long duration may change soil properties to an extent that leads to making soil ill. The use of chemical fertilizers is getting movement because of quick response, easy availability on subsidized rate, and a significant increase in crop yield, leading to higher economic gain in early year of availability; while during the latter part, the inability of other sources to cater to the need of plant nutrition, intensification of cropping systems to cater to the need of growing human and cattle population and decreasing availability, along with the increased cost of other sources of plant nutrition such as animal waste, are major reasons for the monopoly of chemical fertilizers. These nutrients supplied through chemical fertilizers remain available for a short period of time because of their property of changing chemical nature, and may get lost from the scene along with moving water. The imbalance in the use of these fertilizers and lack of attention for fertilization of secondary nutrients, such as sulfur, and micronutrients, viz . Fe and Zn, lead to their widespread deficiency ( Tandon, 2013 ). This all leads to multi-nutritional deficiency and varied levels of soil degradation (Section Issues Related With Soil Health).
At present, the selection of nutrient source should be such that it provides multiple nutrients for higher yield, has considerable residual effects, and positive influence on soil properties, thereby on soil health and less on environmental footprints. This all will be difficult to achieve through a single source of nutrition. At the same time, the economy of crop nutrition may be improved through partly replacement of chemical fertilizers with other on-farm sources or cost-effective off-farm resources. The sources of crop nutrition, which helps in maintaining or improving soil health along with providing nutrition, are agro-industrial wastes, minerals without processing, green and brown manures, weed manures, and bio-fertilizers. The diversification of nutrient sources toward more responsiveness to soil health is constrained by the availability of highly subsidized chemical fertilizers and their quick, significant, and positive impact on crop production, lack of sufficient organic sources of nutrition, as well as their logistic and on-point availability, less contribution of other sources (microbial inoculation and mineral wastes) to crop nutrition. These sources along with their impact on soil health are discussed below.
Green and Brown Manures
The growth of leguminous plants and their in-situ trampling at the flowering stage by tillage (plowing) or incorporation of leaves and young twigs of plants collected from another area is called green manuring. The significance of using of green manuring crops has been recognized long ago ( Pieters, 1927 ) for its capacity to provide nitrogen ( Yang et al., 2018 ) and enhance soil organic carbon ( Ramesh and Chandrasekaran, 2004 ); while its multifarious effects on crop production ( Fageria, 2007 ; Valadares et al., 2016 ) and their quantification in various crops and locations are getting movement afterward. The use of green manuring is more common in rice-based cropping systems and, again, in lowland or irrigated rice ecosystems ( Pooniya et al., 2012 ). Brown manuring is a co-culture of Sesbania and rice, in which after 40–50 days of sowing, Sesbania is knocked down by a spray of herbicide (2,4-D). It is more common in upland rice and reported for its potential for controlling weeds in direct seeded rice ( Gangaiah and Prasad Babu, 2016 ; Maitra and Zaman, 2017 ). The significance of green and brown manuring in soil health improvement reported by different authors is summarized in Table 10 . All these reports indicate that both green and brown manuring have a significant and positive effect on soil health along with their contribution to yield improvement and saving on the application of chemical fertilizers. At the same time, green manure has an immense potential to be an important source of crop nutrition in organic farming, which is getting momentum in India. Green manure crops occupy the land for 40 to 55 days during which one productive crop can be raised. At the same time, sufficient water in the soil is needed for proper decomposition and release of nutrients for present season crops, which is a major constraint in rainfed agriculture. Additional cost is incurred in the purchase of seeds and the application of nutrients to green manure crops (phosphorus application) and knockdown of brown manure crops. These are the weak points that make green or brown manuring difficult to be adopted by farmers on a large scale.
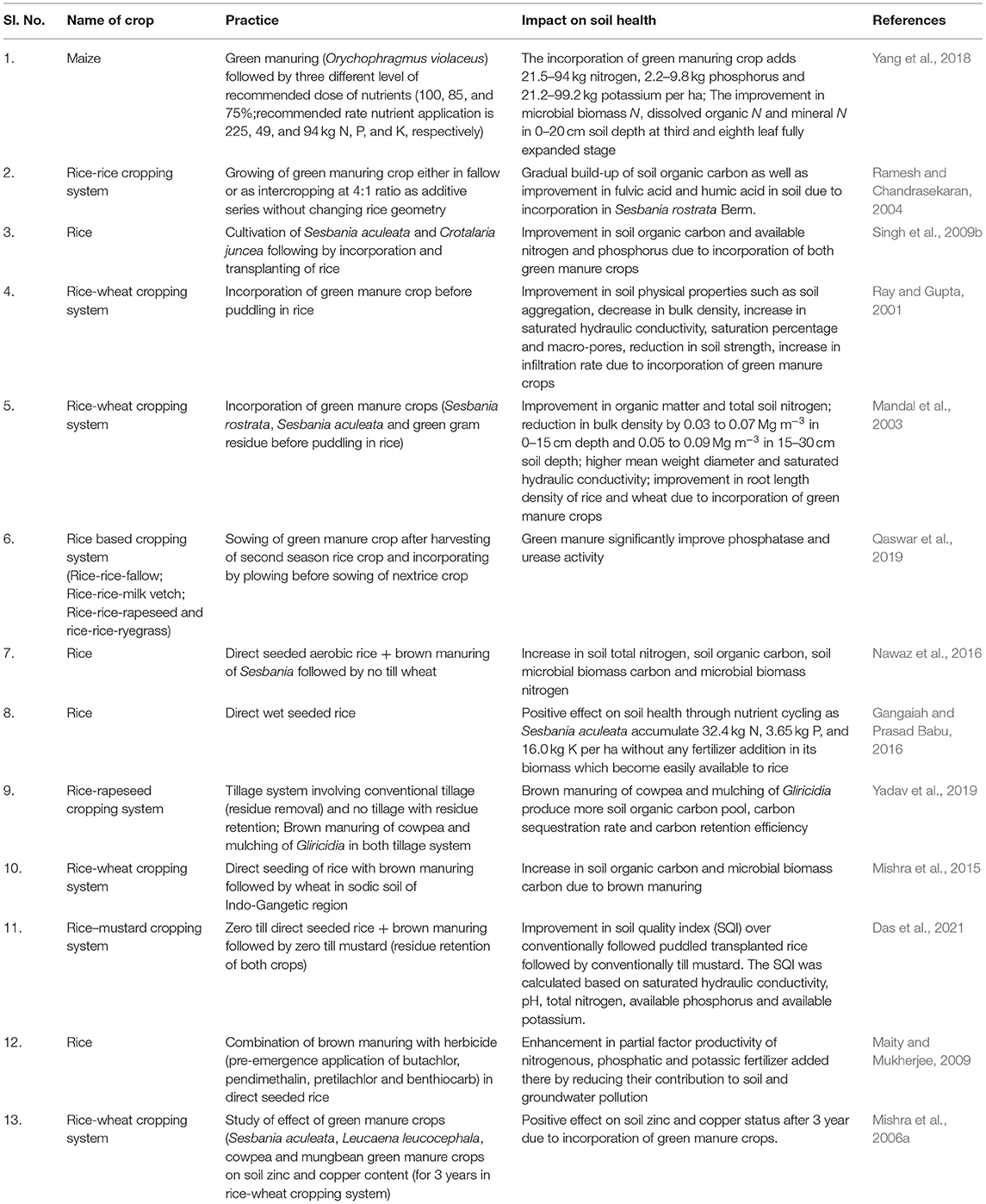
Table 10 . Effects of green and brown manuring on soil health.
Use of Crop Residue and Agro-Industrial Waste
Arable crop production occupies the largest area out of the gross cropped area under cultivation as compared with other crops, such as horticultural crops. Considering the harvest index of arable crops and the nutrient composition of these residues ( Sadh et al., 2018 ), these may be the potential options for the diversification of nutrient sources in agriculture. At the same time, most of the wastes generated from agriculture are voluminous and will add a large amount of organic carbon in soil, which is a backbone of different processes. Another important fact about crop residue is that a large part remains unutilized in cereal-based cropping systems in irrigated areas; while in rainfed farming, due to a large number of competitive uses, it is not available as a nutrient source. The logistic and policy initiative for residue utilization as a source of crop nutrition, blending of different crop residues to enhance nutrient content and faster release of nutrients and location-specific identification, and promotion of cost-effective processes for converting crop residues into suitable forms to be used as a source of nutrition are the thrust area for promoting the use of crop residues as a source of nutrition. The amount of crop residues generated in India from major crops is given in Figure 1 . At the global level, residues produced from six major crops (rice, wheat, barley, sugarcane, maize, and soybean) is 3.7 Pg (billion tons) dry matter year −1 ( Bentsen et al., 2014 ); while Lal (2005) reported 3.8 Pg year −1 residue production. The use of crop residues as a source of crop nutrition will be a win-win situation, as it helps to reduce the unutilized waste for agriculture, and their contribution to pollution and footprint, and success in diversifying chemical fertilizer-dominated nutrient management strategies. The co-culture of legumes in cereal-dominated cropping systems, changing nutrient management strategies by accounting the nitrogen need for in-situ decomposition of high C:N ratio crop residue, increased the availability of seeding machines in residue retention and adapting harvesting techniques that maintain a sufficient amount of residues in the soil at marginal farms need to be considered as options to attract stakeholders toward utilization of crop residues as a potential option for crop nutrition. In the case of organic farming, there is a need for such options as the use of in-situ organic sources of nutrition will be more cost-effective than purchased organic sources of nutrition considering increasing prices of off-farm organic sources of nutrition. Out of the total crop residue generated, the share of cereal crops is highest, which have a higher C:N ratio and takes longer time for decomposition and causes immobilization of soil nitrogen; while other crop residues have competitive uses. Crop residues infected with pests and diseases may increase the inoculums for the infection of the crop in the succeeding growing season.
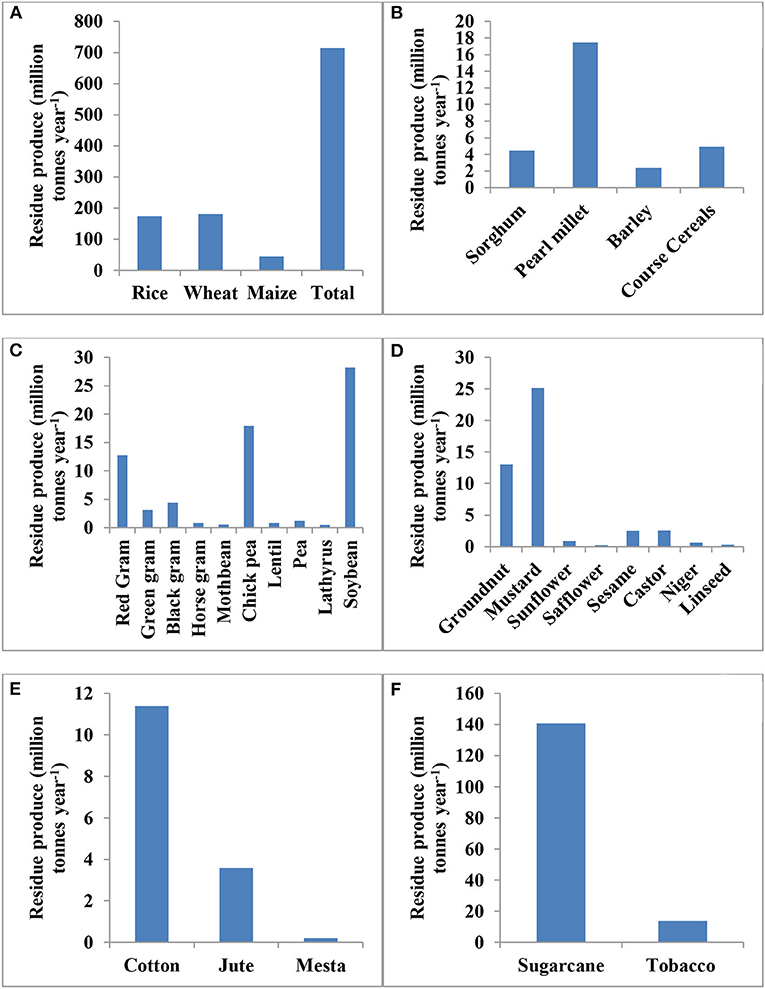
Figure 1 . Residue production from different crops in India (A–F) ( Anonymous, 2019 ).
In addition to the above-discussed unprocessed crop residues, residues are also generated while making crop produce suitable for consumption like processing and value addition of crop produce. Major residues from the processing industry include sugarcane factory waste (bagasse, pressmud, and molasses), waste from rice and wheat milling industry, waste from the fruit and vegetable processing industry, waste from the edible and non-edible oil extraction industry, waste generated during the marketing of perishable commodities, and food wastage. The use of agro industrial waste is constrained by the fact that part residues generated from these agro-industries have more economical competitive uses and therefore remain unavailable to be used as a source of nutrient; while part of unutilized residues needs some treatment before being utilized as a crop nutrient source. Information of such pre-treatments and facilities at the community or individual farmer level will be helpful for enhancing their utilization. Another difficulty is associated with the logistics of such agro-industrial waste on account of their large volume.
The significance of using crop residues and agro-industrial wastes in soil health is listed as follows:
• improvement in soil organic carbon content;
• serves as the food and fuel for microbial diversity, and also help in enriching the population diversity of desirable microbes in soil;
• help in reducing the impact of soil physical degradation processes because of positive impact on soil physical properties such as soil aggregation and infiltration rate;
• soil organic carbon enhances the cation exchange capacity, base saturation, and chelation of micronutrients, buffering pH, thereby enhancing soil chemical health;
• and improves soil chemical health through the process of decomposing soil pollutants, which is also fastening by increasing soil organic carbon.
Use of Minerals and Mineral Waste
The restricted supply of micronutrients is a common constraint for plant growth worldwide, especially in organic farming systems where nutrient supply to crops mostly depends on the mineralization of native soil organic matter, decomposition of applied manures, and crop residues. Based on a laboratory incubation study conducted for 140 days to investigate the potential release of copper (Cu), manganese (Mn), and zinc (Zn) from the rock mineral flour (RMF), the results showed that about 4.6% of Cu added as RMF was released irrespective of the quantity of the RMF applied. Zn release from RMF increased from 5.8 to 15.5%, with an increase in the amount of RMF applied ( Shivay et al., 2010 ). These results showed that RMF could be used to meet Cu, Mn, and Zn requirements of organically grown cereals. The use of minerals as a source of crop nutrition without any chemical processing ( Kulasekaran et al., 2015 ) is getting highlighted, and their significance can be explained as follows:
• inability of organic resource to fulfill the need for crop nutrition at present production requirement;
• identification of microbial processes and availability of microbial cultures for enhancing the nutrient availability from minerals making the mineral matter available form;
• availability of mineral waste generated by different processing industries and problem of their disposal;
• increased cost of processing of minerals to make chemical fertilizers and dependence on import of raw material for preparation of chemical fertilizers;
• availability of large amount of mineral, which is unsuitable to be used as raw material for preparation of chemical fertilizers ( Kumari and Phogat, 2008 );
• suitability of minerals in raw form in specific situation such as suitability of rock phosphate in acidic soil ( Sharma and Prasad, 2003 );
• and despite the above-mentioned positive impacts on soil health, there are several constraints that make the use of mineral waste difficult. The amount of heavy metals present in minerals and their availability to crop, logistics of voluminous raw minerals, and awareness of the processes and conditions making minerals a suitable source of crop nutrition are the primary hurdles that need to be addressed in making use of minerals in agriculture a suitable option for diversification of nutrient sources.
The low nutrient content, slow release of minerals due to longer time required for disintegration, less change of acting as a non-point source of pollution, lower cost of by-product of processing industry such as basic slage and phosphogypsum., and capacity to work as complimentary and supplementary sources of crop nutrition in organic farming are the other points that need to be considered in making mineral and mineral waste a possible alternative for diversification of nutrient sources.
The positive effects of these on soil health include:
• enhance the soil mineral composition from a crop production point of view thereby increasing the soil inherent nutrient supplying capacity;
• enhance the population and diversity of desirable microbes that are needed for biogeochemical cycling of nutrients in soil;
• and reduce the adverse effect of chemical fertilizer-generated abnormalities on soil properties.
Use of Weeds as Manures
The weed being unwanted plants and categorized as biotic stress can be harvested and used as manure. The weed plants as a composite group generally had a higher concentration of nutrients as compared with crop plants. The important points to be considered while using weeds as manure in crop cultivation are as follows:
• Weeding is generally done during early growth, leading to low dry matter accumulation and thereby lower nutrient accumulation in them.
• Not a viable option of nutrient diversification after seed formation, as it again creates a problem in the next year.
• If precautions are taken, such as using pre-emergence herbicides and following proper cultural measures, then the population and dry matter generated would be very minimal.
• The weeds are composite flora and because of the diversity of species with respect to time and space dimension, it becomes difficult to quantify the expected amount of the nutrients added by weeds.
• At the same time, weeds compete with crop plants and absorb nutrients supplied for crop plants, thereby affecting their growth.
• Some species of weeds have an allelopathic effect on crop plants while decomposing their residue. This may affect the growth of crop plants.
• Weeds grown during the fallow period help in conserving soil moisture and also reduce losses in the fertile top soil layer. This will help in maintaining soil fertility.
• Other positive effects of weeds manures on soil health are the same as those of the addition of crop residues through organic matters.
Adaptation of Modern Tillage System (Minimum/ Zero Tillage, Stubble Mulch Tillage)
The conventional plow tillage involves physical manipulation of soil; therefore, it has several implications on soil health that can be seen primarily on soil physical health, soil biological health, and lastly on soil chemical health. The major objective of conventional plow-based tillage is managing weeds along with preparing of seedbeds with required soil physical properties. Due to the availability of an alternative strategy for weed management (herbicides) and maintaining soil physical condition suitable for sowing of crops without tillage, the present plow-based tillage system is molding to a new form, which is collectively called conservation tillage. The other reasons responsible for the emergence and adoption of the conservation tillage system include the adverse effect of plow-based tillage on soil degradation through erosion and fading organic carbon, increasing prices of energy (petroleum) required for tillage operation, government policy orientation in developed countries during early days, problem of disposal of crop residue in intensive cereal-based cropping systems, short time availability for field preparation in intensive cropping systems, availability of tillage equipment for seeding with least disturbance to soil and in layer of crop residue, and positive effect of conservation tillage in various combinations of resource conservation technology.
The conservation tillage system is based on three major principles, viz . continuous or minimal mechanical soil disturbance, maintenance of a permanent biomass soil mulch cover on the ground surface, and diversification of crop species ( Kassam et al., 2019 ). It consists of different forms such as zero tillage, minimum tillage, and stubble mulch tillage. The positive effect of this tillage system on soil health is indicated by the three above-mentioned principles of conservation agriculture, and increasing area under conservation tillage indicates economic gain either in tangible or non-tangible forms by stakeholders. The health improvements achieved by following the conservation tillage system are listed below:
• Reduction in the rate of soil erosion through wind and water action, which can be achieved because of a reduction in erodibility.
• Increase in soil organic carbon as a minimum 30% of surface covered with crop residue is the principle of the conservation tillage system.
• Enhance the microbial population and diversity, soil microbial biomass carbon and nitrogen, and soil microbial enzymatic activities of microorganisms because of the availability of organic matters as their food.
• Improvement in major soil physical parameters such as water holding capacity, soil aggregation, infiltration rate, porosity, bulk density, and soil strength, thereby making soil physically healthy.
• Added crop residues are a source of multiple plant nutrients and therefore enhance the chemical health of soil.
• Soil chemical properties such as temperature moderation, buffering soil pH, nutrient holding capacity, and ion exchange capacity are positively affected by conservation tillage.
• Some hurdles in the adoption of the conservation tillage-based system include competitive uses of crop residues, immobilization of nitrogen during residue decomposition, acting of crop residues as a hibernating material for crop pests and diseases causing pathogen, build-up of termite population, reduced crop germination, and difficulty in manure and fertilizer application.
• The third principle of CA (crop species diversification) can reduce the extraction of nutrients from the same soil layer, and if fertility restorer crops (such as legumes and grasses) are included in the cropping system, then it will have positive and beneficial effects on soil health.
• Maintaining crop residues also helps in correcting soil root zone salinity because of reduced evaporation losses.
Enhancing Soil Microbial Diversity (Use of PGPR and Microbial Consortia, Use of Biocontrol Agents)
There are two possible ways to enhance soil microbial diversity. The first one is the direct addition of microbial culture and the other is the enhancement of the inherent soil microbial population by providing a suitable environment for microbial growth. The direct improvement of soil microbial diversity was started with the use of biofertilizers (microbial inoculation having the capacity of nutrient acquisition/ fixation) for nitrogen fixation. The possible options for enhancing microbial diversity are as follows:
• Use of microbial cultures that have a capacity for nutrient acquisition and fixation.
• Use of microbial cultures that have an antagonistic interaction with disease-causing microorganisms and deleterious rhizobacteria.
• Use of microbial cultures that fasten the rate of organic matter turnover ( Choudhary et al., 2016 ).
• Use of microorganisms that secrete growth-promoting hormones such as auxin ( Zahir et al., 2004 ).
• Use of microbes that have a capacity to fasten the decomposition residue of agrochemicals or soil pollutants (soil security; Nayak et al., 2018 ).
The indirect ways for enhancing microbial population diversity include:
• Using organic sources of crop nutrition as per availability and economic consideration in varied combinations of chemical fertilizers.
• Changing tillage system from conventional plow-based to conservation tillage.
• Use of soil amendments for correcting soil reactions as a pH range near neutral is suitable for different types of microbial growth and processes.
• Crop diversification with place for legumes and forage crops. Legume crops secrete a large amount of carbon material through their roots, and their rhizosphere is rich in microbial diversity ( Kumar et al., 2018 ). Forage crops, such as Napier grass, produce a large amount of root biomass; while growing of berseem was reported to have a positive effect on soil physical and chemical properties.
• Following harvesting methods that maintain at least part of above-ground plants on the soil surface.
• Irrigation management practices for modification of soil microclimates suitable for microbial growth that include drainage of excess water, creation and utilization of irrigation facility from rain water or water from above ground or below ground reservoirs, and irrigation for reducing soil salinity.
• Increasing the use of resource conservation technologies such as green and brown manuring, use of organic mulches and different land configurations such as permanent beds.
There are certain lacunae that make it difficult to adopt the direct methods of enhancing soil microbial population, and include low economic gain and less visibility of crop growth and yield improvement due to their uses, the growth and population build-up is affected by soil environment and weather condition, higher sensitivity to agrochemicals, and their availability in pure form without admixture of any other material. The indirect options of enhancing soil microbial diversity have economic bias; hence, their uniform and wider implications in the favor of enhancing the soil health remain frozen.
Positive Effects of Microbial Enhancement on Soil Health
The impact of improvement in microbial diversity on soil health is overlapped by the impact of diversification of nutrient sources, as both are interdependent on their capacity to improve soil health. The crop residue serves as a raw material for microbial activities; while microbes are important agents for decomposition or turnover of diversified nutrient sources. Some of the additional positive effects of enhancing soil microbial diversity are as follows:
• Enhancing soil microbial diversity fastens the decomposition of agrochemicals and other harmful plant secretions, thereby making soil pollution free.
• Short term storage of plant nutrition through the process of immobilization, thereby reducing losses in plant nutrients.
• Helps in reducing the population of soil-borne disease-causing microorganisms because of antagonistic interaction and competition for same natural resources.
• Improves soil chemical health by increasing the share of fixed forms of nutrients in crop nutrition. This is most prominently seen in the case of phosphorus, as the use efficiency of phosphorus applied through soluble chemical fertilizers hardly exceeds 15–20% ( Roberts and Johnston, 2015 ; Prasad et al., 2018 ); while most of the phosphorus remains in the soil in a fixed form.
Efficient Resource Cycling Through Integrated Farming System
In the urge of an ambitious project of doubling the income of farmers in India, several agricultural interventions have to play an integrated role ( Anonymous, 2018a ). One such option suggested to achieve this target is curtailing the cost of purchased resources through the generation and use of on-farm resources and their recycling or multiple uses in production systems. This is possible through the integrated farming system (IFS) approach involving the integration of more than one enterprise complementing the main enterprise (which is most of the time a cropping system). As this resource cycling through IFS is linked with economic gain, it can be smoothly adopted by farmers, and soil health improvement through this option is complimentary with the involvement of very less monetary inputs.
The possible options for soil health improvement through resource recycling in IFS are:
• Incorporation of small animals and birds (poultry) with higher liquidity of capital (as the investment on feed and space is less and for short time). These animals can be reared on on-farm inputs, and their excreta are a boon to soil health improvement.
• Installation of crop by-product enrichment plants such as vermi-composting unit and composting unit.
• Installation of a biogas unit and use of slurry as manure (it reduces methane emission from direct application of biomass).
• Planting of leguminous plants such as Leucana leucocephala, Gliricidia , which can serve as green manuring crops.
The integrated farming system has a positive effect on soil due to the followings reasons:
• The efficient cycling of by-products reduces wastage and enhances the biogeochemical cycling of plant nutrition, which is the basis of soil chemical health.
• The final by-products after multiple uses (such as use of crop residue for cattle feed or for mushroom production or for vermi-composting) of the resources have a retained and sometimes even enhanced nutritional value, which can be a valuable soil amendment.
• The complementary interaction between natural resources and different enterprises helps in making a closed system of nutrient cycling. This ultimately helps in enhancing the sustainability of the system.
Marginal farm area, difficulty in marking of small produce, complex interactions among enterprises, difficulty at farmer level to have expertise in all enterprises, lack of awareness on the positive interactions among enterprises, low risk-bearing ability, and capital investment are the major bottlenecks of implementing IFS-based systems.
Soil Health Improvement in Problem Soil (Through Use of Soil Amendments, and by Crop Cultivation Practices and Phytoremediation)
Problematic soils in India mainly consist of salt-affected soil and acidic soil with an area extension of 6.73 million ha ( Sharma et al., 2016 ) and 15.93 million ha, respectively. However, at the global level, 0.34 billion and 0.56 billion ha of the area have saline and sodic soil, respectively ( Shahid et al., 2018 ). Along with this, there are soils that are getting polluted because of untreated industrial effluents, sewage water and waste from landfill areas, and seepage of industrial pollutants. These soils have several problems and need special management practices and input addition along with normal management practices for successful crop production. These practices are broadly divided as follows.
Use of Soil Amendments and Its Effect on Soil Health
Soil amendments are mainly added to bring the soil reaction to the desirable range, thereby improving soil health. Considering soil reactions, exchangeable sodium percentage and electrical conductivity of the soil are broadly classified as saline, sodic (alkali), and saline-sodic (alkali) soil. Saline soil is dominated by soluble salts such as sulfate and sodium chloride; while the dominant salt in sodic soil is sodium carbonate. In the case of saline soil, the leaching of soluble salts below the root zone with plenty of fresh water is followed. Along with that, limestone and iron pyrite are chemical soil amendments that can be added. In the case of sodic soil, gypsum, sulfur, iron sulfate, and iron pyrite may be added to improve the soil condition. The improvement for acidic soil is done by liming with calcium oxide, calcium hydrate, dolomite, calcite, or basic slag.
The application of soil amendments for the correction of sodic soil has a significant and positive effect on soil health through improvement in soil properties such as aggregation, porosity, and infiltration rate, replacing exchangeable sodium concentration from exchange complexes and bringing the pH in the neutral range. In acidic soil, the application of liming materials leads to a reduction in the toxic concentration of metal elements such as Fe, Mn, and Al, enhancement of the availability of phosphorus, calcium, magnesium, and potassium, and enhancement of the activity and diversity of microbes in the soil. These improvements in soil health make the soil fit for crop cultivation.
Cultivation Practices
Along with the addition of soil amendments, cultivation practices are also reported to be beneficial for the management of problematic soil. These are as follows:
Soil Tillage
Deep plowing in order to increase infiltration of rainfall moisture to a considerable depth, compartmental bunding, which increases the opportunity time for infiltration of rainwater and opening of a dead furrow, which acts as a drainage channel during an event of heavy rainfall and stores moisture, are suggested modifications.
Land Configuration
Land leveling, which reduces depression spots where water gets collected and there may be an accumulation of salts and different land configurations such as ridges and furrows, and sowing of crop ¾ height of ridges are also suggested for efficient crop cultivation in problematic soils.
Selection of Crops, Mulching, and Irrigation
Crops tolerant of saline soil such as mustard, barley, cotton, and sugar beet ( Jehangir et al., 2013 ) are suggested; while for sodic/alkali soil, Karnal grass, para grass, rhodes grass, rice, sugar beet, and green manure crops such as dhaincha ( Sesbania aculeata ) are suggested ( Chhabra, 1996 ). Other suggested measures are the application of excessive water during pre-sowing irrigation for leaching of salts, frequent and shallow irrigation, use of fresh quality irrigation water, and use of organic mulches to reduce evaporation losses, which will reduce the upward movement of salts.
All these cultivation practices improve soil physical properties and promote soil microbial population and diversity, which ultimately contribute to soil health improvement. The addition of organic matters due to the growing of crops, application of mulches, and suitable microclimate provided by irrigation help in increasing microbial population, thereby improving soil biological health.
Phytoremediation
It is defined as the use of higher plants for the cost effective, environmental-friendly rehabilitation of soil and groundwater contaminated by toxic metals and organic compounds ( Aken, 2011 ). Phytoremediation plays a role in soil health improvement through its capacity to combat soil pollution. It is achieved by phytoextraction (phytoaccumulation), phytovolatilization, phytostabilization, or phytodegradation ( Yan et al., 2020 ). This strategy is important for heavy metal pollutants, organic pollutants, industrial effluents, sewage water, waste for landfills used as manure, etc. Nowadays, phytoremediation is essential as town compost and waste water from cities is increasingly used in agriculture in peri-urban areas mainly for the growing of vegetables and flowers. Therefore, these areas have polluted soil that needs to be reclaimed in a cost-effective way. At the same time, the use of agrochemicals is now a regular practice and is increasing day by day because of changes in the level of biotic stresses and the need to produce more from limited resources. Therefore, soil pollution is going to be an important reason for soil degradation in times to come. Some of such situations are observed in parts of India where soil ground water is becoming polluted because of the excessive use of agrochemicals ( Kaur and Kaur, 2019 ). Considering this, it has become essential to incorporate the phytoremediation strategy in agricultural production systems. Besides pollution in agricultural land, areas for dumping of waste are increasing at an alarming rate ( Kumar et al., 2017 ; Kiran et al., 2020 ), and they will act as a source of contaminants for agriculturally useful land in the future, and these are areas within the scope of phytoremediation. Another important consideration for the phytoremediation technique is that it does not show any significant effect on crop growth and development in the short term, but it helps in improving soil health by reducing the adverse effect of pollutants on human and animal health.
Mimicry of Natural Ecosystem in Agro-Ecosystem for Soil Health Improvement
An agroecosystem is a natural ecosystem modified for the production of different provisional services ( Hodgson, 2012 ), and it is characterized by both planned and unplanned diversities ( Power, 2013 ). It differs from a natural ecosystem in terms of low species and genetic diversity, open system of nutrient cycling, simple and linear tropical interaction, and, most importantly, it heavily depends on human interference for its different functions ( Odum, 1969 ). All of these make an agroecosystem fragile, leading to concern about its sustainability. Along with it, several types of human-induced land degradation (Sections Soil Physical Degradation to Soil Ecosystem Services) add to the instability of agroecosystems. On the other hand, natural ecosystems have several types of self-regulating and self-sustaining functions having the potential to be used in agroecosystems. Studying such functions and identifying the optimum niche of agroecosystems for their successful incorporation in agroecosystems is called mimicking the natural ecosystem. According to Dore et al. (2011) , the incorporation of certain characteristics of natural ecosystems into agroecosystems would improve some properties of agroecosystems, such as productivity, stability, and resilience, and that could be considered as mimicry of agroecosystems. This mimicry of natural ecosystems needs to have an economic bias along with improving long-term sustainability for higher adoption at the used end. For the successful implementation of mimicry of natural ecosystems in agroecosystems, Dore et al. (2011) mentioned certain steps, which are listed below:
• Selection of functions that agronomists wish to improve.
• Identification, in natural ecosystems, of characteristics modifying these functions (diversity, microclimate, soil microbes interaction).
• Definition of qualitative and quantitative relationships linking properties and functions (slash and burn cultivation).
• Transposition of these functions to agricultural conditions.
• Use of these functions for the design of agroecosystems with specified aims.
• Checking that the new agroecosystems express the targeted functions and have no undesirable properties.
Along with this, the concept of ecological intensification ( Tottonell, 2014 ) of agriculture also found a sustainable strategy and had a positive impact on soil health. The options for mimicking natural ecosystems with economic consideration include diversification of cropping systems, crop intensification in space and time dimensions (mixed or inter cropping and crop rotation), residue incorporation, less disturbance to soil (changing tillage system to zero or minimum tillage), multi-storied cropping, and many more. The concept of conservation agriculture, organic farming, integrated farming systems, and groups of resource conservation technologies are parallel with the concept of mimicry of natural ecosystems. Therefore, the positive effect of mimicry of natural ecosystems on soil health will be the same as that of the effect of the above-mentioned technology.
Alternative Agriculture (Agroforestry) for Soil Health Management of Marginal Land
Along with the strategy for reducing the degradation of agricultural land, a suitable strategy for the management of already severely degraded land or marginal land unsuitable for regular cultivation is the need of hours. At the global level, the extent of degraded land has been reported from <1 billion to as high as 6 billion ha ( Gibbs and Salmon, 2015 ). Further, degraded land can be realized by seeing the land use pattern of India, which shows that 17.47 million ha of the area have barren and un-culturable lands and 13.24 million ha of the area have culturable waste lands ( Anonymous, 2019 ). The areas are hardly suitable for regular cultivation of arable crops and if desired, then additional management practices are required, which may not be economical. A suitable economical alternative for restoration of such areas is possible through alternative agriculture such as agroforestry ( Anonymous, 2018b ). The food and agricultural organization define agroforestry as a collective name of land use systems and technologies where woody perennials are deliberately used on the same land management units as agricultural crops and/or animals, in some form of special arrangement or temporal sequence. The system is self-sustaining because of the involvement of diversified components such as arable crops, forage species, tree components, and domestic animals, with three basic systems, viz . agrisilviculture, silvopastoral, and agrisilvopastoral. The positive effects of agroforestry on soil health are as follows:
• The tree component of agroforestry protects soil from erosion through an extensive root network and large canopy. It is also helpful in stabilizing gullies and preventing their spread. At the same time, it produces a large amount of woody matter if retained over a longer duration and can be claimed as carbon credit.
• The grass component involved in agroforestry helps conservation of soil against erosion due to thick cover on ground and also enhances soil organic carbon. This leads to reduction in land degradation.
• Leguminous tree and shrubs species such as Acacia Senegal (L) Willd., Cajanuscajan L., Gliricidia sepium, Sesbania sesban , and Tephrosia spp ., enrich the soil through biologically fixed nitrogen along with the addition of organic matter through leaf fall ( Ribeiro-Barros et al., 2018 ). This will help enhance soil biological health.
• As a self-sustaining system, agroforestry is a cost-effective option for the management of soil health on degraded and waste land, with additional income through wood and fodder produced.
• The areas along field boundaries, farm roads, or canals that remain barren and severely affected by one or other types of land degradation will also be suitable for one or other components of agroforestry. This leads to enhanced biodiversity of cultivated farms, thereby enhancing the soil health of farms as a whole.
• The agroforestry system, as a whole, generates several functions that will help in biogeochemical nutrient cycling with the active involvement of biosphere components such as plants and microorganisms.
Conclusions
In the present day, soil no more remains a medium for plant growth but it turns into a valuable resource for mankind to meet its requirement of provisional services from plants and animals receding in agroecosystems. Considering the present level of land degradation, there is a need to develop and implement novel approaches to maintain soil health with a similar or even higher level of production from agroecosystems. Concepts such as diversification of nutrient sources with emphasis on the use of organic manures and other alternatives to compliment and supplement the chemical fertilizer-based approach will have the potential to contribute significantly to the improvement of soil health. The diversification of production systems through the adoption of conservation agriculture and organic farming is worth considering their role in soil health improvement. The closed system of nutrient cycling achieved through an integrated farming system, will be the self-sustained option of soil health management, along with improvement in resource use efficiency. There is a need to give attention to soil biological health, with the involvement of attempts to enhance soil microbial diversity and curtailment of soil pollution caused by the extensive use of agrochemicals (such as chemical fertilizers).
Author Contributions
AS has prepared the first draft of the manuscript. YS have conceptualized and edited the manuscript. Both authors contributed to the article and approved the submitted version.
Conflict of Interest
The authors declare that the research was conducted in the absence of any commercial or financial relationships that could be construed as a potential conflict of interest.
Publisher's Note
All claims expressed in this article are solely those of the authors and do not necessarily represent those of their affiliated organizations, or those of the publisher, the editors and the reviewers. Any product that may be evaluated in this article, or claim that may be made by its manufacturer, is not guaranteed or endorsed by the publisher.
Aken, B. V. (2011). “Transgenic plants and associated bacteria for phyto-remediation of organic pollutants,” in Comprehensive Biotechnology, 2nd Edn , eds M. Moo-Young (Cambridge, MA: Academic press), 223–237. doi: 10.1016/B978-0-08-088504-9.00330-5
CrossRef Full Text | Google Scholar
Al-shammary, A. A. G., Kouzani, A. Z., Kaynak, A., Khoo, S. Y., Norton, M., and Gates, W. (2018). Soil bulk density estimation methods: a review. Pedosphere 28, 581–596. doi: 10.1016/S1002-0160(18)60034-7
Anonymous (2011). Methods Manual on Soil Testing in India. Department of Agriculture & Cooperation Ministry of Agriculture Government of India, 207 . Available online at: http://agriculture.uk.gov.in (accessed May 23, 2021).
Anonymous (2016). Guidelines for Reclamation of Problem Soils (RPS)- A Sub Scheme of RKVY, Natural Resource Management Division, Ministry of Agriculture and Farmer Welfare, Government of India, New Delhi , 1–12. Available online at: agricoop.nic.in (accessed February 7, 2021).
Anonymous (2018a). Report of the Committee on Doubling Farmers' Income, Volume IX, Farmer Linked Activities and Secondary Agriculture, Department of Agriculture, Cooperation and Farmers' Welfare, Ministry of Agriculture and Farmers' Welfare, 154+v . Available online at: http://agricoop.gov.in/sites/default/files/DFI%20Volume%209.pdf (accessed February 7, 2021).
Anonymous (2018b). Economics of Desertification, Land Degradation and Drought in India, Volume I: Microeconomic Assessment of the Cost of Land Degradation in India . New Delhi: The Energy and Resource Institute, 149+xviii.
Anonymous (2019). Agricultural Statistics at a Glance 2019, Directorate of Economics and Statistics, Department of Agriculture Cooperation and Farmers Welfare, Ministry of Agriculture and Farmers Welfare, Government of India, xviii+315 . Available online at: agricoop.nic.in; http://eands.dacnet.nic.in (accessed December 30, 2020).
Google Scholar
Are, K. S. (2019). “Bioachar and soil physical health,” in Bioachar-An Imperative Amendment for Soil and Environment , eds V. Abrol and P. Sharma [ebook (pdf)]. ISBN, 978–1-83881-989-7.
Arshad, M. A., and Gill, K. S. (1996). Field pea response to liming of an acid soil under two tillage systems. Can. J. Soil Sci. 68, 549–555. doi: 10.4141/cjss96-068
Ashraf, M. A., Maah, M. J., and Yusoff, I. (2014). “Soil contamination, risk assessment and remediation,” in Environmental Risk Assessment of Soil Contamination , ed M. C. Hernandez-Soriano (IntechOpen publication). Available online at: https://www.intechopen.com/books/environmental-risk-assessment-of-soil-contamination/soil-contamination-risk-assessment-and-remediation (accessed January 4, 2021).
PubMed Abstract | Google Scholar
Aulakh, M. S., and Sidhu, G. S. (2015). “Soil degradation in India: causes, major threats, and management options,” in MARCO Symposium 2015 – Next Challenges of Agro-Environmental research in Monsoon Asia . Tsukuba: National Institute for Agro-Environmental Sciences (NIAES), 151–156.
Awad, S. R., and El Fakharany, Z. M. (2020). Mitigation of waterlogging problem in El-Salhiya area, Egypt. Water Sci. 34, 1–12. doi: 10.1080/11104929.2019.1709298
Backovic, D. (2008). “Soil pollution,” in Encyclopaedia of Public Health , ed W. Kirch (Dordrecht: Springer). doi: 10.1007/978-1-4020-5614-7_3280
Balfour, E. B. (1943). The Living Soil (1st Edn.). Faber and Faber publication . Available online at: https://www.pinterest.com/pin/522206519262354047/
Barbieri, P. A., Echeverria, H. E., Rozas, H. R. S., and Picone, L. I. (2006). Nitrogen use efficiency from urea applied to a wheatgrass ( Elytrigia elongate ) prairie is a sodic soil. Austr. J. Exp. Agri. 46, 535–543. doi: 10.1071/EA04180
Batey, T. (2009). Soil compaction and soil management- a review. Soil Use Manag. 25, 335–345. doi: 10.1111/j.1475-2743.2009.00236.x
Baveye, P. C., Baveye, J., and GOwdy, J. (2016). Soil ecosystem services and natural capital: critical appraisal of research on uncertain ground. Front. Environ. Sci. 4:41. doi: 10.3389/fenvs.2016.00041
Bedano, J. C., Dominguez, A., and Arolfo, R. (2011). Assessment of soil biological degradation using mesofauna. Soil Tillage Res. 117, 55–60. doi: 10.1016/j.still.2011.08.007
Belnap, J. (2005). “Crust- biological,” in Encyclopedia of Soils in the Environment , ed D. Hillel (London: Elsevier publication), 339–334. doi: 10.1016/B0-12-348530-4/00131-4
Bennett, H. H. (1943). Adjustment of agriculture to its environment. Ann. Assoc. Am. Geogr. 33, 163–198. doi: 10.1080/00045604309357249
Bentsen, N. S., Felby, C., and Thorsen, B. J. (2014). Agricultural residue production and potential for energy and materials services. Progr. Energy Combust. Sci. 40, 59–73 doi: 10.1016/j.pecs.2013.09.003
Berihun, T., Tolosa, S., Tadele, M., and Kebede, F. (2017). Effect of biochar application on growth of garden pea ( Pisum sativum L.) in acidic soils of Bule Woreda Gedeo Zone Southern Ethiopia. Int. J. Agron. 2017:6827323. doi: 10.1155/2017/6827323
Bernardi, A. C. C., Almeida Machado, P. L. O., Madari, B. E., Tavares, S. R. L., Campos, D. V. B., and Crisostomo, L. A. (2007). Carbon and nitrogen stocks of an arenosol under irrigated fruit orchards in semiarid Brazil. Sci. Agricola 64, 169–175 doi: 10.1590/S0103-90162007000200010
Bhardwaj, T., and Sharma, J. P. (2013). Impact of pesticides application in agricultural industry: an Indian Scenario. Int. J. Agri. Food Sci. Technol. 4, 817–822. Available online at: http://www.ripublication.com/ijafst.htm
Bhattacharyya, R., Ghosh, B. N., Mishra, P. K., Mandal, B., Rao, C. S., Sarkar, D., et al. (2015). Soil degradation in India: challenges and potential solutions. Sustainability 7, 3528–3570. doi: 10.3390/su7043528
Bhuyan, R. (2019). A review note on shifting cultivation in Northeast India amidst changing perceptions. Dhaulagiri J. Sociol. Anthropol. 13, 90–95. doi: 10.3126/dsaj.v13i0.24252
Bian, M., Zhou, M., Sun, D., and Li, C. (2013). Molecular approaches unravel the mechanism of acid soil tolerance in plants. Crop J. 1, 91–104. doi: 10.1016/j.cj.2013.08.002
PubMed Abstract | CrossRef Full Text | Google Scholar
Blecourt, M. D., Grongroft, A., Baumann, S., and Eschenbach, A. (2019). Losses in soil organic carbon stocks and soil fertility due to deforestation for low-input agriculture in semi-arid southern Africa. J. Arid Environ. 165, 88–96. doi: 10.1016/j.jaridenv.2019.02.006
Bouma, J., Dekker, L. W., and Haans, J. C. F. M. (1980). Measurement of depth of water table in heavy clay soil. Soil Sci. 130, 264–270 doi: 10.1097/00010694-198011000-00006
Bouyoucos, G. (1962). Hydrometer method for making particle size analysis of soils. J. Agron. 54, 464–465. doi: 10.2134/agronj1962.00021962005400050028x
Brackin, R., Schmidt, S., Walter, D., Bhuiyan, S., Buckley, S., and Anderson, J. (2017). Soil biological health—what is it and how can we improve it? Proc. Austr. Soc. Sugarcane Technol. 39, 141–154. Available online at: https://www.wettropicsplan.org.au/var/nrm/storage/original/application/9db142b1bdc67da1900dd29e0df13ecb.pdf
Brar, B. S., Dheri, G. S., Lal, R., Singh, K., and Walia, S. S. (2015). Cropping system impacts on carbon fractions and accretion in typic Ustochrept soil of Punjab. J. Crop Improv. 29, 291–300. doi: 10.1080/15427528.2015.1016251
Bremner, J. M. (1960). Determination of nitrogen in soil by Kjeldahl method. J. Agri. Sci. 55, 11–33. doi: 10.1017/S0021859600021572
Brevik, E. C. (2009). “Soil health and productivity,” in Soils, Plant Growth and Crop Production. Encyclopedia of Life Support Systems (EOLSS), Developed under the Auspices of the UNESCO , ed W. Verheye (Oxford: EOLSS Publishers). Available online at: http://www.eolss.net (accessed January 4, 2021).
Brevik, E. C., and Burgess, L. C. (2013). Soils and Human Health . Boca Raton, FL: CRC Press.
Brevik, E. C., and Sauer, T. J. (2015). The past, present, and future of soils and human health studies. SOIL 1, 35–46. doi: 10.5194/soil-1-35-2015
Brevik, E. C., Steffan, J. J., Burgess, L. C., and Cerdà, A. (2017). “Links between soil security and the influence of soil on human health,” in Global Soil Security. Progress in Soil Science Series , eds D. Field, C. Morgan, and A. McBratney (Rotterdam: Springer), 261–274. doi: 10.1007/978-3-319-43394-3_24
Brivik, E. C. (2018). A Brief History of Soil Health Concept . Available online at: http://profile.soil.org/posts/field-and-hostorical-notes/a-brife-historyof-soil-health-concept (accessed November 30, 2020).
Cann, M., Dumanski, J., and Brklacich, M. (1992). The Impacts of Soil Degradation on Crop Yields in the Canadian Prairies: An Annotated Bibliography . Available online at: https://www1.agric.gov.ab.ca/$department/deptdocs.nsf/all/sag11262/$file/Impact_of_Soil_Degradation_on_Crop_Yields.pdf? (accessed January 30, 2021).
Cardoso, E. J. B. N., Vasconcellos, R. L. F., Bini, D., Miyauchi, M. Y. H., Santos, C. A., Alves, P. R. L., et al. (2013). Soil health: looking for suitable indicators. What should be considered to assess the effects of use and management on soil health? Sci. Agricola 70, 274–289. doi: 10.1590/S0103-90162013000400009
Casanova, M., Tapia, E., Seguel, O., and Salazar, O. (2016). Direct measurement and prediction of bulk density on alluvial soils of central Chile. Chilean J. Agric. Res . 76:105–113 doi: 10.4067/S0718-58392016000100015
Casida, L. E. J., Klein, D. A., and Santaro, T. (1964). Soil dehydrogenase activity. Soil Sci. 98, 371–376. doi: 10.1097/00010694-196412000-00004
Chapman, H. D. (1965). Cation-exchange capacity. In: C.A. Black (ed.). Methods of soil analysis - Chemical and microbiological properties. Agronomy 9, 891–901. doi: 10.2134/agronmonogr9.2.c6
Chaudhary, T. N., and Kar, S. (1998). “Aggregate and clod size distribution,” in Theory and Practices in Agrophysics Measurements , eds R. P. Gupta and B. P. Ghildyal (Mumbai: Allied publishers Ltd.), 61–71.
Chauhan, B. S., Mahajan, G., Sardana, V., Timsina, J., and Jat, M. L. (2012). Productivity and sustainability of the rice-wheat cropping system in indo-Gangetic plains of the Indian subcontinent: problems, opportunities' and strategies. Adv. Agron. 117, 315–369. doi: 10.1016/B978-0-12-394278-4.00006-4
Chhabra, R. (1996). Soil Salinity and Water Quality . Brookfield, TV: CRC Press, A. A. Balkema publishers), xv+282.
Choudhary, K., Mohanty, M., Sinha, N. K., Rawat, A., Hati, K. M., Saha, R., et al. (2015). Rooting behaviour of chickpea ( Cicer arietinum ) as affected by soil compaction levels in Vertisol of central India. Ind. J. Agri. Sci. 85, 1085–1091.
Choudhary, M., Sharma, P. C., Jat, H. S., Nehra, V., McDonald, A. J., and Garg, N. (2016). Crop residue degradation by fungi isolated from conservation agriculture fields under rice-wheat system of North-west India. Int. J. Recycl. Org. Waste Agri. 5, 349–360. doi: 10.1007/s40093-016-0145-3
Costanza, R., D'Arge, R., de Groot, R., Farberk, S., Grasso, M., Hannon, B., et al. (1997). The value of world's natural ecosystem services and natural capital. Nature 387, 253–260. doi: 10.1038/387253a0
Das, D. K., and Chong, W. N. (1998). “Particle size distribution analysis of soil and textural classification,” in Theory and Practices in Agrophysics Measurements , eds R. P. Gupta, and B. P. (Mumbai: Ghildyal Allied publishers Ltd.), 31–44.
Das, S., Bhattacharyya, R., Das, T. K., Sharma, A. R., Dwivedi, B. S., Meena, M. C., et al. (2021). Soil quality indices in a conservation agriculture based rice-mustard cropping system in North-western Indo-Gangetic plains. Soil Tillage Res. 208:104914. doi: 10.1016/j.still.2020.104914
Davidson, E. A., Savage, K., Verchot, L. V., and Navarro, R. (2002). Minimizing artifacts and biases in chamber-based measurements of soil respiration. Agric. For. Meteorol . 113, 21–37 doi: 10.1016/S0168-1923(02)00100-4
Dhaliwal, G. S., Jindal, V., and Dhawan, A. K. (2010). Insect-pest problems and crop losses: changing trends. Ind. J. Ecol. 74, 1–7.
Dhruvanarayana, V. V., and Babu, R. (1983). Estimation of soil erosion in India. J. Irrigation Drain. Engg. ASCE 109, 419–434. doi: 10.1061/(ASCE)0733-9437(1983)109:4(419)
Doran, J. W., and Zeiss, M. R. (2000). Soil health and sustainability: managing the biotic component of soil quality. Appl. Soil Ecol. 15, 3–11 doi: 10.1016/S0929-1393(00)00067-6
Dore, T., Makowaski, D., Malezieux, E., Jolain, N. M., Tchamitchian, M., and Tittonell, P. (2011). Facing up paradigm of ecological intensification in agronomy: revisiting methods, concepts and knowledge. Eur. J. Agron. 34, 197–210. doi: 10.1016/j.eja.2011.02.006
Eswaran, H., Lal, R., and Reich, P. F. (2001). “Land degradation: an overview,” in Responses to Land Degradation. Proc. 2nd. International Conference on Land Degradation and Desertification, KhonKaen, Thailand . eds E. M. Bridges, I. D. Hannam, L. R. Oldeman, F. W. T. Pening de Vries, S. J. Scherr, and S. Sompatpanit (New Delhi: Oxford Press). Available online at: https://www.nrcs.usda.gov/wps/portal/nrcs/detail/soils/use/?cid=nrcs142p2_054028 (accessed December 24, 2020).
Fageria, N. K. (2007). Green manuring in crop production. J. Plant Nutr. 30, 691–719. doi: 10.1080/01904160701289529
FAO (2008). An International Technical Workshop. Investing in Sustainable Crop Intensification. The Case for Improving Soil Health, Vol. 6. Rome: Integrated Crop Management, FAO.
Field, D. J. (2017). “Soil security: dimensions,” in Global Soil Security. Progress in Soil Science , eds D. J. Field, C. L. S. Morgan, and A. B. McBratney (Cham: Springer). doi: 10.1007/978-3-319-43394-3
Frye, W. W., Ebelhar, S. A., Murdock, L. W., and Blevins. (1982). Soil erosion effects on properties and productivity of two Kentucky soils. Soil Sci. Soc. America J. 46, 1051–1055. doi: 10.2136/sssaj1982.03615995004600050033x
Ganeshamurthy, A. N., Kalaivanan, D., and Rajendiran, S. (2020). “Carbon sequestration potential of perennial horticultural crops in India tropics,” in Carbon Management in Tropical and Subtropical Terrestrial Systems , eds P. K. Ghosh, S. K. Mahanta, D. Mandal, B. Mandal, and S. Ramakrishnan (Singapore: Springer), 20. doi: 10.1007/978-981-13-9628-1_20
Gangaiah, B., and Prasad Babu, M. B. B. (2016). Brown manuring as a tool of weed management and contributor to nitrogen nutrition of direct wet seeded rice. Oryza 53, 415–421.
Gardi, C., Visioli, G., Conti, F. D., Scotti, M., Menta, C., and Bodini, A. (2016). High natural value farmland: assessment of soil organic carbon in Europe. In: optimizing the delivery of multiple ecosystem goods and services in agricultural systems. Front. Environ. Sci. 2016:47. doi: 10.3389/fenvs.2016.00047
Gerard, C. J., Burleson, C. A., Cowley, W. R., Bloodworth, M. E., and Kunza, G. W. (1964). Hardpan Formation in Course and Medium Textured Soils in the Lower Rio Grande Valley of Texas. Texas A and M University, Texas agricultural experiment station, R. E. Patterson, Director, College station, Texas, 1–12 . Available online at: http://oakstrust.library.tamu.edu/bitstream/handle/1969.1/90489/bull1007.pdf?sequence=13 (assessed December 12, 2020).
Ghosh, M., and Devi, A. (2019). Assessment of crop growth, soil properties and crop yield in an upland acidic soil with inorganic fertilizer blended with organic amendments in summer rice cropping seasons. Int. J. Recycl. Org. Waste Agri. 8(Suppl.1), S1–S9. doi: 10.1007/s40093-019-0252-z
Ghosh, P. K., Manna, M. C., Dayal, D., and Wanjari, R. H. (2006). Carbon sequestration potential and sustainable yield index for groundnut and fallow-based cropping system. J. Agri. Sci. 144, 249–259. doi: 10.1017/S0021859606006046
Gibbs, H. K., and Salmon, J. M. (2015). Mapping of world degraded lands. Appl. Geogr. 57, 12–21. doi: 10.1016/j.apgeog.2014.11.024
Gillman, G. P., and Sumpter, E. A. (1986). Modification to the compulsive exchange method for measuring exchange characteristics of soils. Austr. J. Soil Res. 24, 61–66. doi: 10.1071/SR9860061
Goulding, K. W. T. (2016). Soil acidification and their importance of liming agricultural soils with particular reference to the United Kingdom. Soil Use Manag. 32, 390–399. doi: 10.1111/sum.12270
Grace, P. R., Post, W. M., and Hennessy, K. (2006). The potential impact of climate change on Australia's soil organic carbon resources. Carbon Balance Manag. 1:14. doi: 10.1186/1750-0680-1-14
Grose, C. J. (1999). Land Capability Handbook, - Guidelines for Classification of AGRICULTURAL LAND in Tesmania, Second Edition, Department of Primary Industrial, Water and Environment, printed by: Foot and Playsted, Launceston, Tasmansia, Australia , 70 + iv.
Gupta, R. P. (1998a). “Macro pore space analysis,” in Theory and Practices in Agrophysics Measurements , eds R. P. Gupta and B. P. Ghildyal (Mumbai: Allied Publishers Ltd.), 140–146.
Gupta, R. P. (1998b). “Particle size analysis by pipette method,” in Theory and Practices in Agrophysics Measurements , eds R. P. Gupta and B. P. Ghildyal (Mumbai: Allied Publishers Ltd.), 45–49.
Gupta, R. P. (1998c). “Penetration resistance and cone index measurements,” in Theory and Practices in Agrophysics Measurements , eds R. P. Gupta and B. P. Ghildyal (Mumbai: Allied Publishers Ltd.), 110–116.
Gupta, V. K. (2013). “Soil analysis for available micronutrients,” in Methods of Analysis of Soils, Plants, Waters Fertilizers and Organic Manures , ed H. L. S. Tandon (New Delhi: Fertilizer Development and Consultation Organization), 204 + xii.
Haberern, J. (1992). A soil health index. J. Soil Water Conserv. 47:6.
Hafez, E. M., El Hassan, W. H. A., Gaafar, I. A., and Seleiman, M. F. (2015). Effect of gypsum application and irrigation intervals on clay saline-sodic soil characterization, rice water use efficiency, growth and yield. J. Agri. Sci. 208–219. doi: 10.5539/jas.v7n12p208
Halim, N. S. A., Abdullah, R., Karsani, S. A., Osman, N., Panhwar, Q. A., and Ishak, C. F. (2018). Influence of soil amendments on the growth and yield of rice in acidic soil. Agronomy 8:165. doi: 10.3390/agronomy8090165
Halvorson, J. J., Smith, J. L., and Papendick, R. I. (1996). Integration of multiple soil parameters to evaluate soil quality: a field example. Biol. Fertil. Soils 21, 207–214. doi: 10.1007/BF00335937
Haney, R. L., Haney, E. B., Smith, D. R., Harmel, D, and White, M. J. (2018). The Soil Health Toll- a theory and initial broad scale application. Appl. Soil Ecol. 125, 162–168. doi: 10.1016/j.apsoil.2017.07.035
Henao, J., and Baanante, C. (2006). Agricultural Production and Soil Nutrient Mining in Africa, Implications for Resource Conservation and Policy Development, Technical Bulletin . Muscle Shoals, AB: An International Center for Soil Fertility and Agricultural Development.
Herrick, J. E., and Jones, T. L. (2002). A dynamic cone penetrometer for measuring soil penetration resistance. Soil Sci. Soc. Am. J. 66, 1320–1324. doi: 10.2136/sssaj2002.1320
Hesse, P. R. (1971). A Text Book of Soil Chemical Analysis . London: John Murray, 528.
Heydari, N. (2019). “Water productivity improvement under saline condition: case study of the saline areas of lower Kharkheh river basin, Iran,” in Multifunctionality and Impact of Organic and Conventional Agriculture , eds J. Moudry, J. Bernas and K. F. Mendes (IntechOpen Publication), 1–20. doi: 10.5772/intechopen.86891
Hodgson, E. (2012). “Human environment: definition, scope and role of toxicology,” in Progress in Molecular Biology and Translational Science , ed E. Hodgson (Cambridge, MA: Academic Press), 1–10.
Howard, S. A. (1943). An Agricultural Testament (PDF) . Oxford, UK: Oxford University Press.
Idowu, J., Ghimire, R., Flynn, R., and Ganguli, A. (2019). Soil Health: Importance, Assessment and Management. Cooperative Extension Service, College of Agriculture, Consumer and Environmental Sciences, Circular 694B aces.nmsu.edu/pubs . Available online at: http://aces.nmsu.edu/pubs/_circulars/CR694B/welcome/html (accessed December 9, 2020).
Indira Devi, P., Thomas, J., and Raju, R. K. (2017). Pesticide consumption in India: a spatiotemporal analysis. Agri. Econ. Res. Rev. 30, 163–172. doi: 10.5958/0974-0279.2017.00015.5
Islam S.M. D. U. Bhuiyan M. A. H. Mohinuzzaman M. Ali Md,. H And Moon S. R. (2017). A soil health card (SHC) for soil quality monitoring of agricultural lands in south-eastern costal region of Bangladesh. Environ. Syst. Res. 6:15. doi: 10.1186/s40068-017-0092-7
Jat, H. S., Datta, A., Sharma, P. C., Kumar, V., Yadav, A. K., Choudhary, M., et al. (2018). Assessing soil properties and nutrient availability under conservation agriculture practices in a reclaimed sodic soil in cereal-based systems of North-West India. Archiv. Agron. Soil Sci. 64, 531–545. doi: 10.1080/03650340.2017.1359415
Jat, M. L., Majumdar, K., McDonald, A., Sikka, A. K., and Porada, R. S. (2015). Book of Extended Summaries. National Dialogue on Efficient Nutrient Management for Improving Soil Health, September 28-29, 2018 . New Delhi: TAAS, ICAR, CIMMYT, IPNI, CSISA, FAI, 56.
Jehangir, I. A., Khan, M. H., and Bhat, Z. A. (2013). Strategies for increasing crop production and productivity in problem soils. Int. J. Forestry Soil Environ. 3, 73–78. Available online at: https://www.ijfse.com/uploadedfiles/IJFSEArchive/IJFSE2013/3(2)/05.pdf
Jian, J., Du, X., and Stewart, R. D. (2020). A database for global soil health assessment. Sci. Data 7:16. doi: 10.1038/s41597-020-0356-3
Johnson, A. I. (1963). A Field Method for Measurement of Infiltration. Geological survey water supply paper 1544-F . Washington, DC: United State Government Printing Office, F-26.
Jones, D. L., Cross, P., Withers, P. J. A., DeLuca, T. H., Robinson, D. A., Quillium, R. S., et al. (2013). Nutrient stripping: the global disparity between food security and soil nutrient stocks. J. Appl. Ecol. 50, 851–862. doi: 10.1111/1365-2664.12089
Karlen, D. L., and Rice, C. W. (2015). Soil degradation: will humankind ever learn? Sustainability 7, 12490–12501. doi: 10.3390/su70912490
Kassam, A., Friedrich, T., and Derpsch, R. (2019). Global spread of conservation agriculture. Int. J. Environ. Stud. 76, 29–51. doi: 10.1080/00207233.2018.1494927
Katyal, J. C., Datta, S. P., and Golui, D. (2016). Global review on state of soil health. In: Katyal, JC, Chaudhari, SK, Dwivedi, BS, Biswas, DR, Rattan, RK and Majumdar, K (Editors) (2016) Soil Health: Concept, Status and Monitoring. Bullet. Ind. Soc. Soil Sci. 30, 1–13.
Kaur, P., and Kaur, L. (2019). Effects of dangerous chemicals present in the environment on the health of rural peoples in the south western region of Punjab. J. Pharmacogn. Phytochemistr. SP4, 159–162. Available online at: https://www.phytojournal.com/archives/2019/vol8issue4S/PartE/SP-8-4-43-808.pdf
Kemper, W. D. (1965). Method of soil analysis: part 1 physical and mineral properties, including statistics of measurement and sampling 9, 511–519. doi: 10.2134/agronmonogr9.1
Khan, A., Khan, S., Khan, M. A., Qamar, Z., and Waqas, M. (2015). The uptake and bioaccumulation of heavy metals by food plants, their effects on plants nutrients and associated health risk: a review. Environ. Sci. Pollut. Res. 22, 13772–13799. doi: 10.1007/s11356-015-4881-0
Khanna, R., and Gupta, S. (2018). Agrochemicals as a potential cause of groundwater pollution: a review. Int. J. Chem. Stud. 6, 985–990. Available online at: https://www.chemijournal.com/archives/2018/vol6issue3/PartO/6-3-164-332.pdf
Kibblewhite, M. G., Ritz, K., and Swift, M. J. (2008). Soil health in agricultural system. Philos. Trans. Royal Soc. B 363, 685–701. doi: 10.1098/rstb.2007.2178
Kiran, S. C., Nagarajaiah, C., Murthy, M. M., and Ranjith, P. C. (2020). Effect of municipal solid waste open dumping on soil, water, crop, human health and its prospective. Int. J. Environ. Climate Change 10, 36–45. doi: 10.9734/ijecc/2020/v10i830216
Koch K. Chappell A. Eyres M. And Scott E. (2015). Monitor soil degradation or triage of soil security: an Australian Challenge. Sustainability 7, 4870–4892. doi: 10.3390/su7054870
Kulasekaran, R., Biswas, A. K., and Patra, A. K. (2015). Zeolitic farming. Ind. J. Agron. 60, 185–191. doi: 10.4103/0019-5154.152525
Kumar, D., Shivay, Y. S., Shiva, D., Kumar, C., and Prasad, R. (2013). Phizospheric flora and influence of agronomic practices on them: a review. Proc. Natl. Acad. Sci. India Sec. B Biol. Sci. 83, 1–14. doi: 10.1007/s40011-012-0059-4
Kumar, M., Jha, A. K., Hazarika, S., Verma, B. C., Choudhury, B. U., Ramesh, T., et al. (2016). Micronutrients (B, Zn, Mo) for improving crop production on acidic soils of northeast India. Natl. Acad. Sci. Lett. 39, 85–89. doi: 10.1007/s40009-015-0409-x
Kumar, R., Chatterjeea, D., Kumawat, N., Pandey, A., Roy, A., and Kumar, M. (2014). Productivity, quality and soil health as influenced by lime in rice bean cultivars in foothills of north-eastern India. Crop J. 2, 338–344. doi: 10.1016/j.cj.2014.06.001
Kumar, S., Meena, R. S., Lal, R., Yadav, G. S., Mitran, T., Meena, B. L., et al. (2018). “Role of legumes in soil carbon sequestration,” in Legumes for Soil Health and Sustainable Management , eds R. Meena, A. Das, G. Yadav, R. Lal (Singapore: Springer), 109–138. doi: 10.1007/978-981-13-0253-4_4
Kumar, S., Smith, S. R., Fowler, G., Velis, C., Kumar, S. J., Arya, S., Rena, K.umar R., and Cheeseman, C. (2017). Challenges and opportunities associated with waste management in India. Royal Soc. Open Sci. 4:160764. doi: 10.1098/rsos.160764
Kumari, K., and Phogat, V. K. (2008). Rock phosphate: its availability and solubility and solubilization in the soil. Agri. Rev. 29, 108–116. Available online at: https://arccjournals.com/journal/agricultural-reviews/ARCC2494
Lal, R. (2001). Soil degradation by erosion. Land Degrad. Dev. 12, 519–539. doi: 10.1002/ldr.472
Lal, R. (2005). World Crop residue production and implications of its use as a biofuel. Environ. Int. 31, 575–584. doi: 10.1016/j.envint.2004.09.005
Lal, R. (2016). Soil health and carbon management. Food Energy Security 5, 212–222. doi: 10.1002/fes3.96
Lal, R., and Moldenhauer, W. C. (1987). Effects of soil erosion on crop productivity. Crit. Rev. Plant Sci. 5, 303–367. doi: 10.1080/07352688709382244
Le Bissonnais, Y. (1996). Aggregate stability and measurement of soil crustability and erodibility: I. Theory and methodology. Eur. J. Soil Sci. 47, 425–437. doi: 10.1111/j.1365-2389.1996.tb01843.x
Le, Q. B., Nkonya, E., and Mirzabaev, A. (2014). Biomass Productivity-based mapping of global land degradation hotspots; ZEF-Discussion Papers on Development Policy No. 193 . Bonn: University of Bonn.
Lehman, R. M., Cambardella, C. A., Stott, D. E., Martinez, V. A., Manter, D. K., Buyer, J. S., et al. (2015). Understanding and enhancing soil biological health: the solution for reversing soil degradation. Sustainability 7, 988–1027. doi: 10.3390/su7010988
Lindsay, W. L., and Norvell, W. A. (1978). Development of DTPA soil test for zinc, iron, manganese and copper. Soil Sci. Soc. America J. 42, 421–428. doi: 10.2136/sssaj1978.03615995004200030009x
Magdoff, F. (2001). Concept, components and strategies of soil health in agroecosystems. J. Nematol. 33, 169–172.
Maitra, S., and Zaman, A. (2017). Brown manuring, an effective technique for yield sustainability and weed management of cereal crops: a review. Int. J. Bioresour. Sci. 4, 1–5. doi: 10.5958/2454-9541.2017.00001.9
Maity, S. K., and Mukherjee, P. K. (2009). Effect of brown manuring on grain yield and partial factor productivity of nutrients in dry direct seeded summer rice ( Oryza sativa L.) under Terai agro-ecological region of West Bengal. J. Crop Weed 5, 31–35. Available online at: https://www.cropandweed.com/archives/2009/vol5issue2/7.pdf
Mandal, B., Majumdar, B., Bandyopadhyay, P. K., Hazra, G. C., Gangopadhyoy, A., Samantaray, R. N., et al. (2007). The potential of cropping systems and soil amendments for carbon sequestration in soils under long-term experiments in subtropical India. Glob. Change Biol. 13, 357–368. doi: 10.1111/j.1365-2486.2006.01309.x
Mandal, U. K., Singh, G., Victor, U. S., and Sharma, K. L. (2003). Green manuring: its effects on soil properties and crop growth under rice-wheat cropping system. Eur. J. Agron. 19, 225–237. doi: 10.1016/S1161-0301(02)00037-0
Mantel, S., and Van Engelen, V. W. P. (1997). The impact of land degradation on food productivity- Case studies of Uruguvay, Argentina and Kenya. Volume 1: Main report. Report 97/01 . Wagenningen, DC: International Soil Reference and Information Centre, 44+iv.
Manyevere, A., Bangira, C., Gotosa, J., Munjonji, L., and Chikwari, E. (2015). Characteristics and management options of crusting soils in a smallholder farming area of the Zambezi metamorphic belt in northern Zimbabwe. South African J. Plant Soil 32, 157–164. doi: 10.1080/02571862.2015.1018355
McGonigle, T. P., Millers, S. M. H., Evans, D. G., Fairchild, G. L., and Swan, J. A. (1990). A new method which gives an objective measure of colonization of roots by vesicular-arbuscular mycorrhizal fungi. New Phytol. 115, 495–501. doi: 10.1111/j.1469-8137.1990.tb00476.x
McKenzie, B. M., Tisdall, J. M., and Vance, W. H. (2011). “Soil physical quality,” in Encyclopedia of Agrophysics, Encyclopedia of Earth Sciences Series , eds J. Glinski, J. Horabik, and J. Lipiec (Dordrecht: Springer), 770–777.
Meena, R. S., Kumar, S., Datta, R., Lal, R., Vijaykumar, V., Betnicky, M., et al. (2020). Impacts of agrochemicals on soil microbiota and management: a review. Land 9:34. doi: 10.3390/land9020034
Middleton, N., and Thomas, D. (1997). World Atlas of Desertification. 2nd Edn. United Nation Environment Programme. London: Arnold publisher, 182+vii . Available online at: http://hdl.handle.net/2050011822/30300 (assessed December 27, 2020).
Mirzabaev, A., Wu, J., Evans, J., García-Oliva, F., Hussein, I. A. G., Iqbal, M. H., et al. (2019). “Desertification,” in Climate Change and Land: An IPCC Special Report on Climate Change, Desertification, Land Degradation, Sustainable Land Management, Food Security, and Greenhouse Gas Fluxes in Terrestrial Ecosystems , eds P. R. Shukla, J. Skea, E. Calvo Buendia, V. Masson-Delmotte, H.-O. Pörtner, D. C. Roberts, et al. (IPCC).
Mishra, B. N., Prasad, R., Gangaiah, B., and Shivakumar, B. G. (2006a). Organic manure for increased productivity and sustained supply of micronutrients zinc and copper in rice-wheat cropping system. J. Sustain. Agri. 28, 55–66. doi: 10.1300/J064v28n01_06
Mishra, U., and Dhar, D. W. (2004). Biodiversity and biological degradation of soil. Resonance 9, 26–33. doi: 10.1007/BF02902526
Mishra, V. K., Sara, R., and Singh, R. K. (2006b). Physical characterization of soils under different land use systems using non-limiting water range as a soil physical index. J. Ind. Soc. Soil Sci. 54, 146–150.
Mishra, V. K., Srivastava, S., Bhardwaj, A. K., Sharma, D. K., Singh, Y. P., and Nayak, A. K. (2015). Resource conservation strategies for rice-wheat cropping systems on partially reclaimed sodic soils of the indo-Gangetic region and their effects on soil carbon. Nat. Resour. For. 39, 110–122. doi: 10.1111/1477-8947.12071
Morin, J. (1993). “Soil crusting and sealing,” in Soil and Tillage in Africa: Need and Challenges, FAO Soil Bulletin 69, Editorial Group . Rome: FAO information Division. Available online at: http://www.fao.org/3/T1696E/t1696e06.htm (accessed December 3, 2020).
Mortvedt, J. J. (1995). Heavy metal contaminants in inorganic and organic fertilizers. Fertil. Res. 43, 55–61. doi: 10.1007/BF00747683
Murtaza, B., Murtaza, G., Imran, M., Amjad, M., Naeem, A., Shah, G. M., et al. (2017). Yield and nitrogen use efficiency in rice-wheat cropping system in gypsum amended saline-sodic soil. J. Soil Sci. Plant Nutr. 17, 686–701. doi: 10.4067/S0718-95162017000300011
Mythili, G., and Goedecke, J. (2016). “Economics of land degradation in India,” in Economics of Land Degradation and Improvement – A Global Assessment for Sustainable Development , eds E. Nkonya, A. Mirzabaev, and J. Von Braun (Washington, DC: Springer Open), 431–469. doi: 10.1007/978-3-319-19168-3_15
NAAS (2018). Soil Health: New Policy Initiatives for Farmers Welfare. Policy Brief No. 3 . New Delhi: National Academy of Agricultural Sciences, 19.
Nawaz, A., Farooq, M., Lal, R., Rehman, A., Hussain, T., and Nadeem, A. (2016). Influence of Sesbania brown manuring and rice residue mulch on soil health, weeds and system productivity of conservation rice-wheat systems. Land Degrad. Dev. 28, 1078–1090. doi: 10.1002/ldr.2578
Nayak, S., Prasanna, R., Pabby, A., Dominic, T. K., and Singh, P. K. (2004). Effect of urea and BGA-Azolla bio-fertilizers on nitrogen fixation and chlorophyll accumulation in soil cores from rice fields. Biol. Fertil. Soils 40, 67–72. doi: 10.1007/s00374-004-0738-2
Nayak, S. K., Dash, B., and Baliyarsingh, B. (2018). “Microbial remediation of persistent agro-chemicals by soil bacteria: an overview,” in Microbial Biotechnology , eds J. Patra, G. Das, H. S. Shin (Singapore: Springer), 13. doi: 10.1007/978-981-10-7140-9_13
Nciizah, A. D., and Wakindiki, I. I. C. (2015). Soil sealing and crusting effects on infiltration rate: a critical review of shortfalls in prediction models and solutions. Archiv. Agron. Soil Sci. 61, 1211–1230. doi: 10.1080/03650340.2014.998203
Nelson, D. W., and Sommers, L. E. (1980). Total nitrogen analysis of soil and plant tissues. J. Assoc. Off. Analyt. Chemists 63, 770–778. doi: 10.1093/jaoac/63.4.770
Nia, S. H., Zarea, M. J., Rejali, F., and Verma, A. (2012). Yield and yield components of wheat as affected by salinity and inoculation with Azospirillum strains. J. Saudi Soc. Agri. Sci. 11, 113–121. doi: 10.1016/j.jssas.2012.02.001
Nielsen, K. B., Kjoller, R., Bruun, H. H., Schnoor, T. K., and Rosendahl, S. (2016). Colonization of new land by arbuscular mycorrhizal fungi. Fungal Ecol. 20, 22–29. doi: 10.1016/j.funeco.2015.10.004
Nielsen, M. N., and Winding, A. (2002). Microorganisms as Indicator of Soil Health . Denmark: National Environmental Research Institute. Technical Report No. 388.
Norment, C. J. (1987). A comparison of three methods for measuring arthropod abundance in Tundra habitats and its implications in avian ecology. Northwest Sci. 61, 191–198.
Nunan, N., Morgan, M. A., and Herlihy, M. (1998). Ultraviolet absorbance (280 nm) of compounds released from soil during chloroform fumigation as an estimate of the microbial biomass. Soil Biol. Biochem. 30, 1599–1603. doi: 10.1016/S0038-0717(97)00226-5
Obalum, S. E., Chibuike, G. U., Peth, S., and Ouyang, Y. (2017). Soil organic matter as sole indicator of soil degradation. Environ. Monitor. Syst . 189:176. doi: 10.1007/s10661-017-5881-y
Odum, E. P. (1969). The strategy of ecosystem development. Science 164, 262–270. doi: 10.1126/science.164.3877.262
Oerke, E. C. (2006). Centenary review- crop losses to pests. J. Agri. Sci. 144, 31–43. doi: 10.1017/S0021859605005708
Okrent, D. (1999). On intergenerational equity and its clash with intragenerational equity and on the need for policies to guide the regulation of disposal of wastes and other activities posing very long time risks. Risk Anal. 19, 877–901. doi: 10.1111/j.1539-6924.1999.tb00449.x
Olsen, S. R., Cole, C. V., Watanabe, F. S., and Dean, L. (1954). “Estimation of available phosphorus in soil by extraction with sodium carbonate,” in USDA Conc (Washington, DC), 933.
Olsen, S. R., and Sommers, L. E. (1982). “Phosphorus,” in Methods of Soil Analysis, Part 2: Chemical and Microbiological Properties, 2nd Edn , ed A. L. Page (Madison, WI: American Society of Agronomy), 406–407.
Olsson, L., Barbosa, H., Bhadwal, S., Cowie, A., Delusca, K., Flores-Renteria, D., et al. (2019). “Land degradation,” in Climate Change and Land: An IPCC Special Report on Climate Change, Desertification, Land Degradation, Sustainable Land Management, Food Security, and Greenhouse Gas Fluxes in Terrestrial Ecosystems , eds P. R. Shukla, J. Skea, E. Calvo Buendia, V. Masson-Delmotte, H.-O. Pörtner, D. C. Roberts, et al. (IPCC).
Pagliai, M., and Painuli, D. K. (1998). “Soil porosity and structure quantification by image analysis and soil micromorphology,” in Theory and Practices in Agrophysics Measurements , eds R. P. Gupta and B. P. Ghildyal (Mumbai: Allied Publishers Ltd), 128–139.
Pagliai, M., and Stoops, G. (2010). “Physical and biological crusts and seals,” in Interpretation of Micromorphological Features of Soils and Regoliths , eds G. Stoops, V. Maecelino and F. Mees (Amsterdam: Elsevier), 419–440. doi: 10.1016/B978-0-444-53156-8.00019-2
Panagos, P., Standardi, G., Borrelli, P., Lugato, E., Montanarella, L., and Bosello, F. (2018). Cost of agricultural productivity loss due to soil erosion in the European Union: from direct cost evaluation approaches to the use of macroeconomic models. Land Degrad. Dev. 29, 471–484. doi: 10.1002/ldr.2879
Patel, T. R., and McFadden, B. A. (1976). A simple spectrophotometric method for measurement of nematode populations. Analyt. Biochem. 70, 447–453. doi: 10.1016/0003-2697(76)90469-3
Pattanayak, S. K., Mishra, U. K., Sarkar, A. K., and Majumdar, K. (2011). Integrated nutrient management for groundnut and redgram on acid soils in Odisha. Better Crops South Asia 2011, 8–10. Available online at: http://www.ipni.net/publication/bca.nsf/0/E74D54E10E51925A852579A4007A2931/$FILE/Page%208%20BCSA%202011.pdf
Pawlas, A. K., Jaruga, A. U., and Smreczak, B. (2019). Soil quality index for agricultural areas under different levels of anthropopressure. Int. Agrophys. 33, 455–462. doi: 10.31545/intagr/113349
Pierce, F. J., and Lal, R. (1994). “Monitoring the impact of soil erosion on crop productivity,” in Soil Erosion: Research Methods, 2nd Edn , ed R. Lal (Ankeny, IA: Soil and Water Conservation Society), 235–263. doi: 10.1201/9780203739358-10
Pieters, A. J. (1927). Green Manuring: Principles and Practices . New York, NY: John Wiley and Sons, Inc.
Pimentel, D., and Burgess, M. (2013). Soil erosion threatens food production. Agriculture 3, 443–463. doi: 10.3390/agriculture3030443
Pooniya, V., Shivay, Y. S., Rana, A., Nain, L., and Prasanna, R. (2012). Enhancing soil nutrient dynamics and productivity of Basmati rice through residue incorporation and zinc fertilization. Eur. J. Agron. 41, 28–37. doi: 10.1016/j.eja.2012.03.004
Power, A. G. (2013). “Ecology of agriculture,” in Encyclopaedia of Biodiversity, 2nd Edn , ed S. A. Levin (Cambridge, CA: Academic Press), 9–15. doi: 10.1016/B978-0-12-384719-5.00006-X
Prasad, R., Hobbs, P., and Shivay, Y. S. (2018). New frontiers in phosphate fertilizers. Ind. J. Fertilisers 14, 20–26.
Prasad, R., Shivay, Y. S., Kumar, D., and Sharma, S. N. (2006). Learning by Doing Exercises in Soil Fertility (A Practical Manual for Soil Fertility) . New Delhi: Division of Agronomy, Indian Agricultural Research Institute, 68.
Qaswar, M., Huang, J., Ahmed, W., Li, D., Liu, S., Ali, S., et al. (2019). Long term green manure rotations improve soil biochemical properties, yield sustainability and nutrient balances in acidic paddy soil under rice-based cropping system. Agronomy 9:780. doi: 10.3390/agronomy9120780
Qureshi, J. N., and Njihia, C. M. (1984). “Soil related constraints to crop production in East Africa,” in Proceedings of the 6th Annual General Meeting of the Soil Science Society of East Africa (Nairobi: Kenya Soil Survey).
Ramesh, K., and Chandrasekaran, B. (2004). Soil organic carbon build up and dynamics in rice-rice cropping system. J. Agron. Crop Sci. 190, 21–27. doi: 10.1046/j.0931-2250.2003.00069.x
Rao, A. S., and Reddy, K. S. (2013). “Analysis of soils for pH, EC and available major nutrients,” in Methods of Analysis of Soils, Plants, Waters Fertilizers and Organic Manures , ed H. L. S. Tandon (New Delhi: Fertilizer Development and Consultation Organization), 204 + xii.
Rao, Y. N., and Jo, I. S. (1998). “Pore space analysis by using mercury intrusion porosimetry,” in Theory and Practices in Agrophysics Measurements , eds R. P. Gupta and B. P. Ghildyal (Mumbai: Allied Publishers Ltd.), 117–126.
Rattan, R. K., Kumar, M., Narwal, R. P., and Singh, A. P. (2009). “Soil health and nutritional Security-Micronutrients,” in Proceedings of Platinum Jubilee Symposium of Indian Society of Soil Science , 249–265.
Ray, S. S., and Gupta, R. P. (2001). Effect of green manuring and tillage practices on physical properties of puddled loam soil under transplanted rice-wheat cropping system. J. Ind. Soc. Soil Sci. 49, 670–678. Available online at: https://www.indianjournals.com/ijor.aspx?target=ijor:jisss&volume=49&issue=4&article=012
Reddy, A. A. (2017). Impact Study of Soil Health Card Scheme . Hyderabad: National institute of Agricultural Extension management (MANAGE), 210+ xxiii.
Reddy, C. V., Tiwari, A., Tedia, K., Verma, A., and Saxena, R. P. (2017). Long term fertilizer experiment on soil properties and yield of rice on chromustert. Int. J. Farm Sci. 7, 87–91. doi: 10.20546/ijcmas.2017.611.012
Ribeiro-Barros, A. I., Silva, M. J., Moura, I., Ramalho, J. C., Maguas-Hanson, C., and Ribeiro, N. S. (2018). “The potential of tree and shrub legumes in agroforestry systems,” in Nitrogen in Agriculture Updates , ed Amanullah (IntechOpen), 223–240. doi: 10.5772/intechopen.69995
Richards, L. A., and Weaver, L. R. (1943). Pressure-plate apparatus for measuring moisture sorption and transmission by soils. Soil Sci. 56, 395–404. doi: 10.1097/00010694-194312000-00001
Rickson, R. J., Deeks, L. K., Graves, A., Harris, J. A. H., Kibblewhite, M. G., and Sakrabani, R. (2015). Input constraints to food production: The impact of soil degradation. Food Security 7, 351–367. doi: 10.1007/s12571-015-0437-x
Roberts, T. L., and Johnston, A. E. (2015). Phosphorus use efficiency and management in agriculture. Resour. Conserv. Recycl. 105, 275–281. doi: 10.1016/j.resconrec.2015.09.013
Rodale, J. I. (1945). Pay Dirt: Farming and Gardening with Composts . New York, NY: Devin Adair Company.
Romig, D. E., Garlynd, M. J., Harris, R. F., and McSweeney, K. (1995). How farmers assess soil health and quality. J. Soil Water Cons . 50, 229–236.
Roy Chowdhury, S., Nayak, A. K., Brahmanand, P. S., Mohanty, R. K., Chakraborty, S., Kumar, A., and Ambast, S. K. (2018). Delineation of Waterlogged Areas using Spatial Techniques for Suitable Crop Management in Eastern India. Bulletin No.79 . Bhubaneswar: ICAR Indian Institute of Water Management, 44.
Sadh, P. K., Duhan, S., and Duhan, J. S. (2018). Agro-industrial waste and their utilization using solid state fermentation: a review. Bioresour. Bioproces. 5:1. doi: 10.1186/s40643-017-0187-z
Saha, S. K. (2003). “Water and wind induced soil erosion assessment and monitoring using remote sensing and GIS,” in Satellite Remote Sensing and GIS Applications in Agricultural Meteorology, Proceeding of Workshop , eds M. V. K. Sivakumar, P. S. Roy, K. Harmsen and S. K. Saha, 315–330.
Saha, S. S., Saha, B. N., Hazra, G. C., Pati, S., Pal, B., Kundu, D., et al. (2018). Assessing the suitability of sewage-sludge produced in Kolkata, India for their agricultural use. Proc. Ind. Natl. Sci. Acad. 84, 781–792. doi: 10.16943/ptinsa/2018/49410
Sahoo, U. K., Singh, S. L., Gogoi, A., Kenye, A., and Sahoo, S. S. (2019). Active and passive soil organic carbon pools as affected by different land use types in Mizoram, Northeast India. PLoS ONE 14:e0219969. doi: 10.1371/journal.pone.0219969
Samineni, S., Siddique, K. H. M., Gaur, P. M., and Colmer, T. D. (2011). Salt sensitivity of the vegetative and reproductive stages in chickpea ( Cicer arietinum L.): podding is a particularly sensitive stage. Environ. Exp. Bot. 71, 260–268. doi: 10.1016/j.envexpbot.2010.12.014
Scofield, A. M. (1986). Organic farming – the origin of the name. Biol. Agri. Horticult. 4, 1–5. doi: 10.1080/01448765.1986.9754481
Sekhar, M., Tomer, S. K., Thiyaku, S., Giriraj, P., Murthy, S., and Mehta, V. K. (2018). Groundwater level dynamics in Bengaluru city, India. Sustainability 10:26. doi: 10.3390/su10010026
Selvi, A., Rajasekar, A., Theerthagiri, J., Ananthaselvam, A., Sathishkumar, K., Madhavan, J., et al. (2019). Integrated remediation processes toward heavy metal removal/recovery from various environments-a review. Front. Environ. Sci . 7:66. doi: 10.3389/fenvs.2019.00066
Shaheenuzzamn, M.d. (2014). Screening of chickpea genotypes against salinity stress. Bangladesh J. Agri. Res. 39, 605–619. doi: 10.3329/bjar.v39i4.22539
Shahid, S. A., Zaman, M., and Heng, L. (2018). “Soil salinity: historical perspectives and a world overview of the problem,” in Guidelines for Salinity Assessment, Mitigation and Adaptation Using Nuclear and Related Techniques (Chem: Springer), 43–53. doi: 10.1007/978-3-319-96190-3_2
Shamshuddin, J., Panhwar, Q. A., Shazana, M. A. R. S., Elisa, A. A., Fauziah, C. I., and Naher, U. A. (2016). Improving the productivity of acid sulfate soils for rice cultivation using limestone, basalt, organic fertilizer and/or their combinations. Sains Malaysiana 45, 383–392. Available online at: http://www.ukm.my/jsm/pdf_files/SM-PDF-45-3-2016/08%20J.%20Shamshudin.pdf
Sharma, D. K., Singh, A., Sharma, P. C., Dagar, J. C., and Chaudhari, S. K. (2016). Sustainable management of sodic soils for crop production: opportunities and challenges. J. Soil Salinity Water Qual. 8, 109–130.
Sharma, G., Sharma, L. K., and Sharma, K. C. (2019). Assessment of land use change and its effect on soil carbon stock using multitemporal satellite data in semiarid region of Rajasthan, India. Ecol. Proces. 8:42. doi: 10.1186/s13717-019-0193-5
Sharma, S. N., and Prasad, R. (2003). Yield and P uptake by rice and wheat grown in a sequence as influenced by phosphate fertilization with diammonium phosphate and Mussoorie rock phosphate with or without crop residues and phosphate solubilizing bacteria. J. Agri. Sci. 141, 359–369. doi: 10.1017/S0021859603003678
Shetty, R., and Prakesh, N. B. (2020). Effect of different biochars on acid soil and growth parameters of rice plants under aluminium toxicity. Sci. Rep. 10:11249. doi: 10.1038/s41598-020-69262-x
Shivay, Y. S., Krogstad, T., and Singh, B. R. (2010). Mineralization of copper, manganese and zinc from rock mineral flour and city waste compost for efficient use in organic farming. Plant Soil 326, 425–435. doi: 10.1007/s11104-009-0023-0
Sims, G. K. (1990). “Biological degradation of soil,” in Advances in Soil Science , eds R. Lal, and B. A. Stewart (New York, NY: Springer), 289–330. doi: 10.1007/978-1-4612-3322-0_8
Singh, A. K., Arora, S., Singh, Y. P., Verma, C. L., Bhardwaj, A. K., and Sharma, N. (2018). Water use in rice crop through different methods of irrigation in a sodic soil. Paddy Water Environ. 16, 587–593. doi: 10.1007/s10333-018-0650-2
Singh, B. K., Campbell, C. D., Sorenson, S. J., and Zhou, J. (2009a). Soil genomics. Nat. Rev. Microbiol. 7:756. doi: 10.1038/nrmicro2119-c1
Singh, D., Chhonkar, P. K., and Pandey, R. N. (1999). Soil, Plant, Water Analysis – A Methods Manual . New Delhi: Division of Soil Science and Agricultural Chemistry, IARI.
Singh, G., Babu, R., Narain, P., Bhushan, L. S., and Abrol, I. P. (1992). Soil erosion rates in India. J. Soil Water Conserv. 47, 97–99.
Singh, R. P., Singh, P. K., and Singh, A. K. (2009b). Effect of green manuring on physic-chemical properties of soil and productivity of rice. Oryza 46, 120–123.
Singh, V. K., Dwivedi, B. S., Tiwari, K. N., Majumdar, K., Rani, M., Singh, S. K., et al. (2014). Optimizing nutrient management strategies for rice–wheat system in the Indo-Gangetic Plains of India and adjacent region for higher productivity, nutrient use efficiency and profits. Field Crop Res. 164, 30–44. doi: 10.1016/j.fcr.2014.05.007
Singh, Y. P., Singh, R., Sharma, D. K., Mishra, V. K., and Arora, S. (2016). Optimizing gypsum levels for amelioration of sodic soils to enhance grain yield and quality of rice ( Oryza sativa L.). J. Ind. Soc. Soil Sci. 64, 33–40. doi: 10.5958/0974-0228.2016.00005.0
Song, G., Li, L., Pan, G., and Zhang, Q. (2005). Topsoil organic carbon storage of china and its loss by cultivation. Biogeochemistry 74, 47–65. doi: 10.1007/s10533-004-2222-3
Song, G., Li, S., and Hui, R. (2017). Effect of biological soil crusts on seed germination and growth of an exotic and two native plant species in an arid ecosystem. PLoS ONE 12:e0185839. doi: 10.1371/journal.pone.0185839
Sonneveld, B. G. J. S., Keyzer, M. A., and Ndiaye, D. (2016). Quantifying the impact of land degradation on crop production: the case of Senegal. Solid Earth 7, 93–103. doi: 10.5194/se-7-93-2016
Stafford, E. (1931). Skeleton planting. J. Forestry 29, 41–47.
Sterk, G., and Stoorvogel, J. J. (2020). Desertification: scientific versus political realities. Land 9:156. doi: 10.3390/land9050156
Stewart, W. D. P., Fitzgerald, G. P., and Burris, R. H. (1967). In-situ studies on N2 fixation using the acetylene reduction technique. Proc. Natl. Acad. Sci. U. S. A. 58, 2071–2078. doi: 10.1073/pnas.58.5.2071
Subbiah, B. V., and Asija, G. L. (1956). A rapid procedure for assessment of available nitrogen in rice soils. Curr. Sci. 25, 259–260.
Sur, H. S., and Gupta, R. P. (1998). “Infiltration rate measurements,” in Theory and Practices in Agrophysics Measurements , eds R. P. Gupta and B. P. Ghildyal (Mumbai: Allied Publishers Ltd.), 191–194.
Swaminathan, M. S. (1987). “The promise of agroforestry for ecological and nutritional security,” in Agroforestry: A Decade of Development , eds H. A. Steppler and P. K. R. Nair (Nairobi: International Council for Research in Agroforestry), 25–41.
Swarnalakshmi, K., Prasanna, R., Kumar, A., Pattnaik, S., Chakravarty, K., Shivay, Y. S., et al. (2013). Evaluating the influence of novel cyanobactrial biofilmedbiofertilizers on soil fertility and plant nutrition of wheat. Eur. J. Soil Biol. 55, 107–116. doi: 10.1016/j.ejsobi.2012.12.008
Tabatabai, M. A., and Bremner, J. M. (1969). Use of p-Nitrophenyl phosphate for assay of soil phosphatase activity. Soil Biol. Biochem. 1, 301–307. doi: 10.1016/0038-0717(69)90012-1
Tabatabai, M. A., and Bremner, J. M. (1972). Assay of urease activity in soils. Soil Biol. Biochem. 4, 479–487. doi: 10.1016/0038-0717(72)90064-8
Tahat, M. M., Alananbeh, K. M., Othman, Y. A., and Lescovar, D. I. (2020). Soil health and sustainable agriculture. Sustainability 12:4859. doi: 10.3390/su12124859
Tandon, H. L. S. (2013). Methods of Analysis of Soils, Plants, Waters, Fertilizers and Organic Manures. New Delhi: Fertilizer Development and Consultation Organization, 204 + xii.
Timsina, J., Singh, V. K., and Majumdar, K. (2013). Potassium management in rice-maize systems in south Asia. J. Plant Nutr. Soil Sci. 176, 317–330. doi: 10.1002/jpln.201200253
Tingwu, L., Juan, X., Guangyong, L., Jianhua, M., Jianping, W., Zhizhong, L., et al. (2003). Effect of drip irrigation with saline water on water use efficiency and quality of watermelons. Water Resour. Manag. 17, 395–408. doi: 10.1023/B:WARM.0000004917.16604.2c
Tottonell, P. (2014). Ecological intensification of agriculture- sustainable by nature. Curr. Opin. Environ. Sustainabil. 8, 53–61. doi: 10.1016/j.cosust.2014.08.006
USDA (1936). Agricultural Adjustment Administration, Soil Health and National Wealth . Washington, DC: USDA.
USDA (1961). Land capability classification, Agriculture Handbook No. 210.Soil conservation service . Washington, DC: U.S. Department of Agriculture, U.S. Government Printing Office, 21 + iii.
Valadares, R. V., Avila-Silva, L. D., Teixeira, R. D. S., De Sousa, R. N., and Vergutz, L. (2016). “Green manures and crop residues as source of nutrients in tropical environment,” in Organic Fertilizers – From Basic Concepts to Applied Outcomes , eds M. L. Larramendy and S. Soloneski (IntechOpen publication). Available online at: https://www.intechopen.com/books/organic-fertilizers-from-basicconcepts-to-applied-outcomes/green-manures-and-crop-residues-assource-of-nutrients-in-tropical-environment (accessed November 20, 2020).
Valentin, C., and Brasson, L. M. (1992). Morphology, genesis and classification of surface crusts in loamy and sandy loam soil. Geoderma 55, 225–245. doi: 10.1016/0016-7061(92)90085-L
Valentin, C., and Brasson, L. M. (1997). “Soil crusting,” in Methods for Assessment of Soil Degradation, Advances in Soil Science , eds R. Lal, W. H. Blum, C. Valentine and B. A. Stewart (Boca Raton, FL: CRC Press), 89–107.
Van Bruggen, A. H. C., and Semenov, A. M. (2000). In search of biological indicators for soil health and disease suppression. Appl. Soil Ecol. 15, 13–24. doi: 10.1016/S0929-1393(00)00068-8
Walia, M., and Goyal, S. (2010). Effect of heavy metal contaminated sewage sludge on soil microbiological properties and growth of India mustard. Archiev. Agron. Soil Sci. 56, 563–574. doi: 10.1080/03650340903165923
Walkley, A. J., and Black, I. A. (1934). An examination of the Degtjareff method for determination of soil organic matter and a proposed modification of the chromic acid titration method. Soil Sci. 37, 29–38. doi: 10.1097/00010694-193401000-00003
Wallace, H. A. (1910). Relation Between Livestock Farming and the Fertility of the Land. Retrospective Theses and Dissertations, 98 . Available online at: http://lib.dr.iastate.edu/rtd/98 (accessed November 20, 2020).
Wander, M. M., Cihacek, L. J., Coyne, M., Drijber, R. A., Grossman, J. M., Gutknecht, J. L. M., et al. (2019). Developments in agricultural soil quality and health: reflections by the research committee on soil organic matter management. Front. Environ. Sci. 7:109. doi: 10.3389/fenvs.2019.00109
Weyers, S. L., Schomberg, H. H., Hendrix, P. F., Spokas, K. A., and Endale, D. M. (2008). Construction of an electrical device for sampling earthworm populations in the field. Appl. Eng. Agri. 24, 391–397. doi: 10.13031/2013.24492
Wiebe, K. (2003). Linking land quality, agricultural productivity and food security. Resource economics division, Economic Research Service, Agricultural Economic Report No. 823. Washington, DC: U.S. Department of Agriculture, 60+iii . Available online at: http://www.ers.usda.gov (accessed January 30, 2021).
Wienhold, B. J., Andrews, S. S., Kuykendall, H., and Karlen, D. L. (2008). Recent advances in soil quality assessment in the United States. J. Ind. Soc. Soil Sci. 56, 1–10. Available online at: https://digitalcommons.unl.edu/usdaarsfacpub/1204
Wiesmeier, M., Poeplau, C., Sierra, C. A., Maier, H., Fruhauf, C., Hubner, R., et al. (2016). Projected loss of soil organic carbon in temperate agricultural soils in the 21st century: effects of climate change and carbon input trends. Sci. Rep. 6:32525. doi: 10.1038/srep32525
Wijitkosum, S. (2020). Reducing vulnerability to desertification by using the spatial measures in a degraded area in Thailand. Land 9:49. doi: 10.3390/land9020049
Williams, A. J., Pagliai, M., and Stoops, G. (2018). “Physical and biological surface crusts and seals,” in Interpretation of Micro-Morphological Features of Soil and Regoliths, 2nd Edn , eds G. Stoops, V. Macrcelino and F. Mees (Amsterdam: Elsevier Publication), 539–574. doi: 10.1016/B978-0-444-63522-8.00019-X
Williams, C. H., and Steinberg, A. (1959). Soil sulphur fractions as chemical indices of available sulphur in some Australian soils. Austr. J. Agri. Res. 10, 340–352. doi: 10.1071/AR9590340
Wischmeier, W. H., and Smith, D. D. (1978). Predicting Rainfall Erosion Losses: Guide to Conservation Planning. USDA, Agriculture Handbook 537 . Washington, DC: U.S. Government Printing Office.
Wishmeier, W. H., and Smith, D. D. (1960). “A universal soil-loss equation to guide conservation farm planning,” in Transactions of Seventh International Congress on Soil Science, Vol. 1 (Madison, WI), 418–425.
Woodruff, N. P., and Siddoway, F. H. (1965). A wind erosion equation, soil science society of America Proceedings 29, 602–608. doi: 10.2136/sssaj1965.03615995002900050035x
Wrench, G. T. (1939). Health and the soil. Br. Med. J. 1, 276–277. doi: 10.1136/bmj.1.4075.276
Yadav, G. S., Das, A., Lal, R., Babu, S., Datta, M., Meena, R. S., et al. (2019). Impact of no tillage and mulching on soil carbon sequestration under rice ( Oryza sativa L.) rapeseed ( Brassica campestris , L. var. rapeseed) cropping system in hilly agro-ecosystem of the Eastern Himalayas, India. Agri. Ecosyst. Environ. 275, 81–92. doi: 10.1016/j.agee.2019.02.001
Yaduvanshi, N. P. S. (2003). Substitution of inorganic fertilizers by organic manures and the effect on soil fertility in a rice-wheat rotation on reclaimed sodic soil in India. J. Agri. Sci. 140, 161–168. doi: 10.1017/S0021859603002934
Yaduvanshi, N. P. S., Setter, T. L., Sharma, S. K., Singh, N. K., and Kulshreshtha, N. (2010). “Waterlogging effect on wheat yield, redox potential, manganese and iron in different soils of India,” in Soil Solutions for a Changing World, 19th World Congress on Soil Science, 1-6 August, 2010 (Brisbane, QLD), 45–48.
Yan, A., Wang, Y., Tan, S. N., Mohd Yusof, M. L., Ghosh, F., and Chen, Z. (2020). Phytoremediation: a promising approach for revegetation of heavy metal-polluted land. Front. Plant Sci. 11:359. doi: 10.3389/fpls.2020.00359
Yang, L., Bai, J., Liu, J., Zeng, N., and Cao, W. (2018). Green manuring effect on changes of soil nitrogen fractions, maize growth, and nutrient uptake. Agronomy 8:261. doi: 10.3390/agronomy8110261
Yoder, R. E. (1936). A direct method of aggregate analysis of soils and a study of the physical nature of erosion losses. Agron. J . 28, 337–351. doi: 10.2134/agronj1936.00021962002800050001x
Zahir, Z. A., Arshad, M., and Frankenberger, W. T. (2004). Plant growth promoting rhizobactaria: application and perspectives in agriculture. Adv. Agron. 81, 97–167. doi: 10.1016/S0065-2113(03)81003-9
Zhang, W. (2018). Global pesticide use: profile, trend, cost/benefit and more. Proc. Acad. Ecol. Environ. Sci. 8, 1–27.
Zingore, S., Mutegi, J., Agesa, B., Tamene, L., and Kihara, J. (2015). Soil degradation in sub-Saharan Africa and crop production options for rehabilitation. Better Crops 99, 24–26. Available online at: https://hdl.handle.net/10568/68702
Keywords: integrated farming system, novel agronomic approaches, soil degradation, soil health, conservation tillage, soil microbial diversity
Citation: Shahane AA and Shivay YS (2021) Soil Health and Its Improvement Through Novel Agronomic and Innovative Approaches. Front. Agron. 3:680456. doi: 10.3389/fagro.2021.680456
Received: 14 March 2021; Accepted: 27 July 2021; Published: 06 September 2021.
Reviewed by:
Copyright © 2021 Shahane and Shivay. This is an open-access article distributed under the terms of the Creative Commons Attribution License (CC BY) . The use, distribution or reproduction in other forums is permitted, provided the original author(s) and the copyright owner(s) are credited and that the original publication in this journal is cited, in accordance with accepted academic practice. No use, distribution or reproduction is permitted which does not comply with these terms.
*Correspondence: Yashbir Singh Shivay, ysshivay@hotmail.com
Disclaimer: All claims expressed in this article are solely those of the authors and do not necessarily represent those of their affiliated organizations, or those of the publisher, the editors and the reviewers. Any product that may be evaluated in this article or claim that may be made by its manufacturer is not guaranteed or endorsed by the publisher.
Thank you for visiting nature.com. You are using a browser version with limited support for CSS. To obtain the best experience, we recommend you use a more up to date browser (or turn off compatibility mode in Internet Explorer). In the meantime, to ensure continued support, we are displaying the site without styles and JavaScript.
- View all journals
- Explore content
- About the journal
- Publish with us
- Sign up for alerts
- Review Article
- Published: 23 August 2022
Soil microbiomes and one health
- Samiran Banerjee 1 &
- Marcel G. A. van der Heijden ORCID: orcid.org/0000-0001-7040-1924 2 , 3
Nature Reviews Microbiology volume 21 , pages 6–20 ( 2023 ) Cite this article
32k Accesses
223 Citations
581 Altmetric
Metrics details
- Microbial ecology
- Soil microbiology
The concept of one health highlights that human health is not isolated but connected to the health of animals, plants and environments. In this Review, we demonstrate that soils are a cornerstone of one health and serve as a source and reservoir of pathogens, beneficial microorganisms and the overall microbial diversity in a wide range of organisms and ecosystems. We list more than 40 soil microbiome functions that either directly or indirectly contribute to soil, plant, animal and human health. We identify microorganisms that are shared between different one health compartments and show that soil, plant and human microbiomes are perhaps more interconnected than previously thought. Our Review further evaluates soil microbial contributions to one health in the light of dysbiosis and global change and demonstrates that microbial diversity is generally positively associated with one health. Finally, we present future challenges in one health research and formulate recommendations for practice and evaluation.
This is a preview of subscription content, access via your institution
Access options
Access Nature and 54 other Nature Portfolio journals
Get Nature+, our best-value online-access subscription
24,99 € / 30 days
cancel any time
Subscribe to this journal
Receive 12 print issues and online access
195,33 € per year
only 16,28 € per issue
Buy this article
- Purchase on Springer Link
- Instant access to full article PDF
Prices may be subject to local taxes which are calculated during checkout
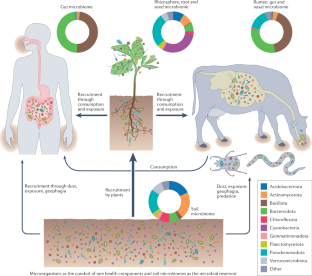
Similar content being viewed by others
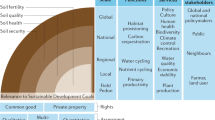
The concept and future prospects of soil health
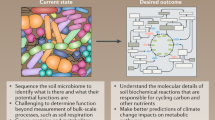
Soil microbiomes and climate change
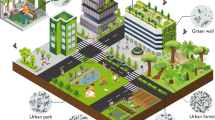
Harnessing soil biodiversity to promote human health in cities
Data availability.
The data for microbiome composition in Fig. 1 are available in Dryad: https://datadryad.org/stash/share/CPLkD5krQ1-MgaaiI3T0eIyGCTolnsN6tgK0sJ5GlQg .
Berg, G. et al. Microbiome definition re-visited: old concepts and new challenges. Microbiome 8 , 1–22 (2020). This article proposes a definition of the microbiome by distinguishing the terms microbiome and microbiota, and provides a discussion on the heterogeneity and dynamics of microbiomes in time and space.
Google Scholar
Berendsen, R. L., Pieterse, C. M. J. & Bakker, P. A. H. M. The rhizosphere microbiome and plant health. Trends Plant. Sci. 17 , 478–486 (2012).
Article CAS Google Scholar
Carthey, A. J. R., Gillings, M. R. & Blumstein, D. T. The extended genotype: microbially mediated olfactory communication. Trends Ecol. Evol. 33 , 885–894 (2018).
Article Google Scholar
Singh, B. K., Liu, H. & Trivedi, P. Eco-holobiont: a new concept to identify drivers of host-associated microorganisms. Environ. Microbiol. 22 , 564–567 (2020).
Adair, K. L., Wilson, M., Bost, A. & Douglas, A. E. Microbial community assembly in wild populations of the fruit fly Drosophila melanogaster . ISME J. 12 , 959–972 (2018).
Trinh, P., Zaneveld, J. R., Safranek, S. & Rabinowitz, P. M. One health relationships between human, animal, and environmental microbiomes: a mini-review. Front. Public Health 6 , 1–9 (2018).
Mackenzie, J., McKinnon, M. & Jeggo, M. in Confronting Emerging Zoonoses: The One Health Paradigm (eds Yamada, A. et al.) 1–254 (2014).
Destoumieux-Garzón, D. et al. The one health concept: 10 years old and a long road ahead. Front. Vet. Sci. 5 , 1–13 (2018).
Rüegg, S. R. et al. A systems approach to evaluate one health initiatives. Front. Vet. Sci. 5 , 1–18 (2018).
Bar-On, Y. M., Phillips, R. & Milo, R. The biomass distribution on Earth. Proc. Natl Acad. Sci. USA 115 , 6506–6511 (2018). This article provides an assessment of the overall biomass composition of the biosphere and shows that terrestrial biomass is about two orders of magnitude higher than marine biomass.
van der Heijden, M. G. A., Bardgett, R. D. & Van Straalen, N. M. The unseen majority: soil microbes as drivers of plant diversity and productivity in terrestrial ecosystems. Ecol. Lett. 11 , 296–310 (2008). This article summarizes various roles of soil microorganisms in terrestrial ecosystems and highlights that soil microorganisms must be considered as important drivers of plant diversity and productivity.
Bardgett, R. D. & van der Putten, W. H. Belowground biodiversity and ecosystem functioning. Nature 515 , 505–511 (2014). This review emphasizes the diversity of microorganisms and animals that live in soils and how microorganisms contribute to ecosystem functioning.
Fierer, N. Embracing the unknown: disentangling the complexities of the soil microbiome. Nat. Rev. Microbiol. 15 , 579–590 (2017). This review highlights the complexity of soil microorganisms and the roles they play in various biogeochemical cycles.
Dias, P. C. Sources and sinks in population biology. Trends Ecol. Evol. 11 , 326–330 (1996).
Edwards, J. et al. Structure, variation, and assembly of the root-associated microbiomes of rice. Proc. Natl Acad. Sci. USA 112 , E911–E920 (2015). This article provides detailed evidence on the assembly and recruitment of the root-associated microbiomes.
Walsh, C. M., Becker-Uncapher, I., Carlson, M. & Fierer, N. Variable influences of soil and seed-associated bacterial communities on the assembly of seedling microbiomes. ISME J. 15 , 2748–2762 (2021).
Rochefort, A. et al. Transmission of seed and soil microbiota to seedling. mSystems 6 , e0044621 (2021).
Bergna, A. et al. Tomato seeds preferably transmit plant beneficial endophytes. Phytobiomes J. 2 , 183–193 (2018).
Abdelfattah, A., Wisniewski, M., Schena, L. & Tack, A. J. M. Experimental evidence of microbial inheritance in plants and transmission routes from seed to phyllosphere and root. Environ. Microbiol. 23 , 2199–2214 (2021).
Bulgarelli, D., Schlaeppi, K., Spaepen, S., van Themaat, E. V. L. & Schulze-Lefert, P. Structure and functions of the bacterial microbiota of plants. Annu. Rev. Plant Biol. 64 , 807–838 (2013). This review highlights the composition of plant-associated microbiota and summarizes the factors that drive their structure and functions.
Fitzpatrick, C. R. et al. Assembly and ecological function of the root microbiome across angiosperm plant species. Proc. Natl Acad. Sci. USA https://doi.org/10.1073/pnas.1717617115 (2018).
Arumugam, M. et al. Enterotypes of the human gut microbiome. Nature 473 , 174–180 (2011). This article performs a metagenomic analysis of samples from four countries and reveals the factors that drive the enterotypes of the human gut microbiome.
Amat, S., Holman, D. B., Timsit, E., Schwinghamer, T. & Alexander, T. W. Evaluation of the nasopharyngeal microbiota in beef cattle transported to a feedlot, with a focus on lactic acid-producing bacteria. Front. Microbiol. 10 , 1988 (2019).
Trivedi, P., Leach, J. E., Tringe, S. G., Sa, T. & Singh, B. K. Plant–microbiome interactions: from community assembly to plant health. Nat. Rev. Microbiol. 18 , 607–621 (2020). This review summarizes the genetic, biochemical and metabolic interactions between the host plant and its associated microbiomes.
Mahaney, W. C. & Krishnamani, R. Understanding geophagy in animals: standard procedures for sampling soils. J. Chem. Ecol. 29 , 1503–1523 (2003).
Liddicoat, C. et al. Naturally-diverse airborne environmental microbial exposures modulate the gut microbiome and may provide anxiolytic benefits in mice. Sci. Total Environ. 701 , 134684 (2020).
Ottman, N. et al. Soil exposure modifies the gut microbiota and supports immune tolerance in a mouse model. J. Allergy Clin. Immunol. 143 , 1198–1206.e12 (2019).
Attwood, G. T. et al. Applications of the soil, plant and rumen microbiomes in pastoral agriculture. Front. Nutr. 6 , 1–17 (2019).
Mcgrath, D., Poole, D. B. R., Fleming, G. A. & Sinnott, J. Soil ingestion by grazing sheep. Ir. J. Agric. Res. 21 , 135–145 (1982).
Healy, W. B. Ingestion of soil by dairy cows. N. Z. J. Agric. Res. 11 , 487–499 (2012).
Ross, A. A., Müller, K. M., Weese, J. S. & Neufeld, J. D. Comprehensive skin microbiome analysis reveals the uniqueness of human skin and evidence for phylosymbiosis within the class Mammalia. Proc. Natl Acad. Sci. USA 115 , E5786–E5795 (2018).
Bindari, Y. R., Moore, R. J., Van, T. T. H., Brown, S. W. W. & Gerber, P. F. Microbial taxa in dust and excreta associated with the productive performance of commercial meat chicken flocks. Anim. Microbiome https://doi.org/10.1186/s42523-021-00127-y (2021).
Sun, H., Peng, K., Xue, M. & Liu, J. Metagenomics analysis revealed the distinctive ruminal microbiome and resistive profiles in dairy buffaloes. Anim. Microbiome 3 , 1–13 (2021).
Sing, D. & Sing, C. F. Impact of direct soil exposures from airborne dust and geophagy on human health. Int. J. Environ. Res. Public Health https://doi.org/10.3390/ijerph7031205 (2010).
Nyanza, E. C., Joseph, M., Premji, S. S., Thomas, D. S. K. & Mannion, C. Geophagy practices and the content of chemical elements in the soil eaten by pregnant women in artisanal and small scale gold mining communities in Tanzania. BMC Pregnancy Childbirth 14 , 1–10 (2014).
Pearson, A. L. et al. Associations detected between measures of neighborhood environmental conditions and human microbiome diversity. Sci. Total Environ. 745 , 141029 (2020).
Liddicoat, C. et al. Ambient soil cation exchange capacity inversely associates with infectious and parasitic disease risk in regional Australia. Sci. Total Environ. 626 , 117–125 (2018).
Stanaway, I. B. et al. Human oral buccal microbiomes are associated with farmworker status and azinphos-methyl agricultural pesticide exposure. Appl. Environ. Microbiol. 83 , e02149-16 (2017).
Shukla, S. K. et al. The nasal microbiota of dairy farmers is more complex than oral microbiota, reflects occupational exposure, and provides competition for staphylococci. PLoS ONE 12 , 1–18 (2017).
Doran, J. W. & Zeiss, M. R. Soil health and sustainability: managing the biotic component of soil quality. Appl. Soil Ecol. 15 , 3–11 (2000).
McBratney, A. B., Field, D. J. & Koch, A. The dimensions of soil security. Geoderma 213 , 203–213 (2014).
Lehmann, J., Bossio, D. A., Kögel-Knabner, I. & Rillig, M. C. The concept and future prospects of soil health. Nat. Rev. Earth Environ. 1 , 544–553 (2020).
Bünemann, E. K. et al. Soil quality — a critical review. Soil Biol. Biochem. 120 , 105–125 (2018). This review provides a critical appraisal of soil quality and summarizes a wide variety of indicators.
Fierer, N., Wood, S. A. & Bueno de Mesquita, C. P. How microbes can, and cannot, be used to assess soil health. Soil Biol. Biochem. 153 , 108111 (2021).
Garland, G. et al. Crop cover is more important than rotational diversity for soil multifunctionality and cereal yields in European cropping systems. Nat. Food 2 , 28–37 (2021).
Tamburini, G. et al. Agricultural diversification promotes multiple ecosystem services without compromising yield. Sci. Adv. 6 , eaba1715 (2020).
Edlinger, A., Garland, G. & Banerjee, S. Agricultural management and pesticide use reduce the functioning of benecial plant symbionts. Nat. Ecol. Evol . https://doi.org/10.1038/s41559-022-01799-8 (2022). This study demonstrates that pesticides reduce the natural nutrient uptake capacity of one of the oldest and most widespread symbionts of plants.
Sallach, J. B., Thirkell, T. J., Field, K. J. & Carter, L. J. The emerging threat of human-use antifungals in sustainable and circular agriculture schemes. Plants People Planet 3 , 685–693 (2021).
Banerjee, S. et al. Agricultural intensification reduces microbial network complexity and the abundance of keystone taxa in roots. ISME J. 13 , 1722–1736 (2019).
Schmidt, M. W. I. et al. Persistence of soil organic matter as an ecosystem property. Nature 478 , 49–56 (2011). This review highlights that the stability of SOM is determined by microhabitat properties.
Liang, C. & Balser, T. C. Microbial production of recalcitrant organic matter in global soils: implications for productivity and climate policy. Nat. Rev. Microbiol. 9 , 75 (2011).
Schimel, J. P. & Schaeffer, S. M. Microbial control over carbon cycling in soil. Front. Microbiol. 3 , 1–11 (2012). This review discusses the role of microorganisms in soil carbon cycling and highlights the ecology of microorganisms in terms of broad and narrow processes in soil.
Kallenbach, C. M., Frey, S. D. & Grandy, A. S. Direct evidence for microbial-derived soil organic matter formation and its ecophysiological controls. Nat. Commun. 7 , 1–10 (2016). This study provides evidence that soil microbial biomass can be a chemically diverse yet stable pool of soil carbon.
Banerjee, S. et al. Microbial interkingdom associations across soil depths reveal network connectivity and keystone taxa linked to soil fine-fraction carbon content. Agric. Ecosyst. Environ. 320 , 107559 (2021).
Bender, S. F., Wagg, C. & van der Heijden, M. G. A. An underground revolution: biodiversity and soil ecological engineering for agricultural sustainability. Trends Ecol. Evol. 31 , 440–452 (2016).
Alori, E. T., Glick, B. R. & Babalola, O. O. Microbial phosphorus solubilization and its potential for use in sustainable agriculture. Front. Microbiol. 8 , 1–8 (2017).
Saha, M. et al. Microbial siderophores and their potential applications: a review. Environ. Sci. Pollut. Res. 23 , 3984–3999 (2015).
Brevik, E. et al. Soil and human health: current status and future needs. Air Soil Water Res. 13 , 1–23 (2020).
Lugtenberg, B. & Kamilova, F. Plant-growth-promoting rhizobacteria. Annu. Rev. Microbiol. 63 , 541–556 (2009). This review discusses the rhizosphere as a habitat and summarizes various beneficial roles of the rhizosphere microbiota.
van der Heijden, M. G. A., Martin, F. M., Selosse, M. A. & Sanders, I. R. Mycorrhizal ecology and evolution: the past, the present, and the future. N. Phytol. 205 , 1406–1423 (2015).
Richardson, A. E., Barea, J. M., McNeill, A. M. & Prigent-Combaret, C. Acquisition of phosphorus and nitrogen in the rhizosphere and plant growth promotion by microorganisms. Plant Soil 321 , 305–339 (2009).
Berg, G., Grube, M., Schloter, M. & Smalla, K. The plant microbiome and its importance for plant and human health. Front. Microbiol. 5 , 1–2 (2014).
Parniske, M. Arbuscular mycorrhiza: the mother of plant root endosymbioses. Nat. Rev. Microbiol. 6 , 763–75 (2008).
Smith, S. & Read, D. Mycorrhizal Symbiosis (Elsevier, 2008).
Hoeksema, J. D. et al. A meta-analysis of context-dependency in plant response to inoculation with mycorrhizal fungi. Ecol. Lett. 13 , 394–407 (2010).
Lekberg, Y. & Koide, R. T. Is plant performance limited by abundance of arbuscular mycorrhizal fungi? A meta-analysis of studies published between 1988 and 2003. N. Phytol. 168 , 189–204 (2005).
Salomon, M. J. et al. Global evaluation of commercial arbuscular mycorrhizal inoculants under greenhouse and field conditions. Appl. Soil Ecol. 169 , 104225 (2022).
Gill, S. S. et al. Piriformospora indica : potential and significance in plant stress tolerance. Front. Microbiol. 7 , 332 (2016).
Geisen, S. et al. Soil protists: a fertile frontier in soil biology research. FEMS Microbiol. Rev. 42 , 293–323 (2018).
Wall, D. H., Nielsen, U. N. & Six, J. Soil biodiversity and human health. Nature 528 , 69–76 (2015).
Backer, R. et al. Plant growth-promoting rhizobacteria: context, mechanisms of action, and roadmap to commercialization of biostimulants for sustainable agriculture. Front. Plant Sci. 871 , 1–17 (2018).
Philippot, L., Raaijmakers, J. M., Lemanceau, P. & Van Der Putten, W. H. Going back to the roots: the microbial ecology of the rhizosphere. Nat. Rev. Microbiol. 11 , 789–799 (2013). This review highlights the importance of understanding the rhizosphere and its microbiota for sustainable agriculture and climate change mitigation.
Schlaeppi, K. & Bulgarelli, D. The plant microbiome at work. Mol. Plant Microbe Interact. 28 , 212–217 (2015).
Compant, S., Samad, A., Faist, H. & Sessitsch, A. A review on the plant microbiome: ecology, functions, and emerging trends in microbial application. J. Adv. Res. 19 , 29–37 (2019).
Fitzpatrick, C. R. et al. The plant microbiome: from ecology to reductionism and beyond. Annu. Rev. Microbiol. 74 , 81–100 (2020).
Deshani Igalavithana, A. et al. Assessment of soil health in urban agriculture: soil enzymes and microbial properties. Sustainability 9 , 310 (2017).
van der Heijden, M. G. A., Bruin, S., De, Luckerhoff, L., van Logtestijn, R. S. & Schlaeppi, K. A widespread plant–fungal–bacterial symbiosis promotes plant biodiversity, plant nutrition and seedling recruitment. ISME J. 10 , 1–11 (2016).
Zhang, J. et al. NRT1.1B is associated with root microbiota composition and nitrogen use in field-grown rice. Nat. Biotechnol. 37 , 676–684 (2019).
Van Elsas, J. D. et al. Microbial diversity determines the invasion of soil by a bacterial pathogen. Proc. Natl Acad. Sci. USA 109 , 1159–1164 (2012). This study shows an inverse relationship between soil microbial diversity and survival of the invading species E. coli O157:H7. The study reveals a lack of competitive ability of invading pathogen in species-rich environments.
Wagg, C., Schlaeppi, K., Banerjee, S., Kuramae, E. E. & van der Heijden, M. G. A. Fungal–bacterial diversity and microbiome complexity predict ecosystem functioning. Nat. Commun. 10 , 1–10 (2019).
Fitzpatrick, C. R., Mustafa, Z. & Viliunas, J. Soil microbes alter plant fitness under competition and drought. J. Evol. Biol. 32 , 438–450 (2019).
Expósito, R. G., de Bruijn, I., Postma, J. & Raaijmakers, J. M. Current insights into the role of rhizosphere bacteria in disease suppressive soils. Front. Microbiol. 8 , 1–12 (2017).
Schlatter, D., Kinkel, L., Thomashow, L., Weller, D. & Paulitz, T. Disease suppressive soils: new insights from the soil microbiome. Phytopathology 107 , 1284–1297 (2017).
McSpadden Gardener, B. B. & Weller, D. M. Changes in populations of rhizosphere bacteria associated with take-all disease of wheat. Appl. Environ. Microbiol. 67 , 4414–4425 (2001).
Mendes, R. et al. Deciphering the rhizosphere microbiome for disease-suppressive bacteria. Science 332 , 1097–1100 (2011).
Kikuchi, Y., Hosokawa, T. & Fukatsu, T. An ancient but promiscuous host–symbiont association between Burkholderia gut symbionts and their heteropteran hosts. ISME J. 5 , 446–460 (2010).
Hannula, S. E., Zhu, F., Heinen, R. & Bezemer, T. M. Foliar-feeding insects acquire microbiomes from the soil rather than the host plant. Nat. Commun. 10 , 1–9 (2019). This study shows soil as the reservoir of microorganisms for leaf-feeding caterpillars and reveals the connection between the soil and insect microbiomes.
Kikuchi, Y. et al. Symbiont-mediated insecticide resistance. Proc. Natl Acad. Sci. USA 109 , 8618–8622 (2012).
Bruijning, M., Henry, L. P., Forsberg, S. K. G., Metcalf, C. J. E. & Ayroles, J. F. Natural selection for imprecise vertical transmission in host–microbiota systems. Nat. Ecol. Evol. 6 , 77–87 (2021).
Wilschut, R. A. & Geisen, S. Nematodes as drivers of plant performance in natural systems. Trends Plant Sci. 26 , 237–247 (2021).
Reese, A. T. & Dunn, R. R. Drivers of microbiome biodiversity: a review of general rules, feces, and ignorance. mBio 9 , e01294-18 (2018).
Peixoto, R. S., Harkins, D. M. & Nelson, K. E. Advances in microbiome research for animal health. Annu. Rev. Anim. Biosci. 9 , 289–311 (2021).
UIA. Soil-borne diseases in animals. The Encyclopedia of World Problems http://encyclopedia.uia.org/en/problem/141320 (2021).
Lerner, P. Nocardiosis. Clin. Infect. Dis. 22 , 891–905 (1996).
Costa, J. L. N. et al. Outbreak of malignant oedema in sheep caused by Clostridium sordellii , predisposed by routine vaccination. Vet. Rec. 160 , 594–595 (2007).
Young, S. L., Sherman, P. W., Lucks, J. B. & Pelto, G. H. Why on earth?: evaluating hypotheses about the physiological functions of human geophagy. Q. Rev. Biol. 86 , 97–120 (2011).
Blum, W. E. H., Zechmeister-Boltenstern, S. & Keiblinger, K. M. Does soil contribute to the human gut microbiome? Microorganisms 7 , 287 (2019).
Hanski, I. et al. Environmental biodiversity, human microbiota, and allergy are interrelated. Proc. Natl Acad. Sci. USA 109 , 8334–8339 (2012). This study reveals the connection between allergy in humans and microbial diversity in environments.
Wills-Karp, M., Santeliz, J. & Karp, C. L. The germless theory of allergic disease: revisiting the hygiene hypothesis. Nat. Rev. Immunol. 1 , 69–75 (2001).
Yazdanbakhsh, M., Kremsner, P. G. & Van Ree, R. Parasites and the hygiene hypothesis. Sci 296 , 490–494 (2002).
Hopping, K. A., Chignell, S. M. & Lambin, E. F. The demise of caterpillar fungus in the Himalayan region due to climate change and overharvesting. Proc. Natl Acad. Sci. USA 115 , 11489–11494 (2018).
Hirt, H. Healthy soils for healthy plants for healthy humans. EMBO Rep. 21 , 1–5 (2020).
Brevik, E. C. & Burgess, L. C. The 2012 fungal meningitis outbreak in the United States: connections between soils and human health. Soil Horiz. 54 , 1–4 (2013).
Steffan, J. J., Brevik, E. C., Burgess, L. C. & Cerdà, A. The effect of soil on human health: an overview. Eur. J. Soil Sci. 69 , 159–171 (2018).
Loukas, A. et al. Hookworm infection. Nat. Rev. Dis. Primers 2 , 1–18 (2016).
Rangel, J. M., Sparling, P. H., Crowe, C., Griffin, P. M. & Swerdlow, D. L. Epidemiology of O157:H7 outbreaks, United States, 1982–2002. Emerg. Infect. Dis. 11 , 603–609 (2005).
Franz, E. et al. Manure-amended soil characteristics affecting the survival of E. coli O157:H7 in 36 Dutch soils. Environ. Microbiol. 10 , 313–327 (2008).
Berg, G., Eberl, L. & Hartmann, A. The rhizosphere as a reservoir for opportunistic human pathogenic bacteria. Environ. Microbiol. 7 , 1673–1685 (2005).
Guzman-Otazo, J. I. et al. Diarrheal bacterial pathogens and multi-resistant enterobacteria in the Choqueyapu River in La Paz, Bolivia. PLoS ONE https://doi.org/10.1371/journal.pone.0210735 (2019).
Barnes, G., Saunders, D. G. O. & Williamson, T. Banishing barberry: the history of Berberis vulgaris prevalence and wheat stem rust incidence across Britain. Plant Pathol. 69 , 1193–1202 (2020).
Lauber, C. L., Hamady, M., Knight, R. & Fierer, N. Pyrosequencing-based assessment of soil pH as a predictor of soil bacterial community structure at the continental scale. Appl. Environ. Microbiol. 75 , 5111–5120 (2009).
Chu, H. et al. Soil bacterial diversity in the Arctic is not fundamentally different from that found in other biomes. Environ. Microbiol. 12 , 2998–3006 (2010).
Wei, Y. et al. Does pH matter for ecosystem multifunctionality? An empirical test in a semi-arid grassland on the Loess Plateau. Funct. Ecol. https://doi.org/10.1111/1365-2435.14057 (2022).
Svenningsen, N. B. et al. Suppression of the activity of arbuscular mycorrhizal fungi by the soil microbiota. ISME J. 12 , 1296–1307 (2018).
Sul, W. J. et al. Tropical agricultural land management influences on soil microbial communities through its effect on soil organic carbon. Soil Biol. Biochem. 65 , 33–38 (2013).
Geyer, K. M., Kyker-Snowman, E., Grandy, A. S. & Frey, S. D. Microbial carbon use efficiency: accounting for population, community, and ecosystem-scale controls over the fate of metabolized organic matter. Biogeochemistry 127 , 173–188 (2016).
Rillig, M. C. et al. The role of multiple global change factors in driving soil functions and microbial biodiversity. Science 366 , 886–890 (2019).
Cavicchioli, R. et al. Scientists’ warning to humanity: microorganisms and climate change. Nat. Rev. Microbiol. 17 , 569–586 (2019). This article is a consensus statement of scientists across the world and highlights how microorganisms affect climate change but are also affected by anthropogenic activities.
Querejeta, J. I. et al. Lower relative abundance of ectomycorrhizal fungi under a warmer and drier climate is linked to enhanced soil organic matter decomposition. N. Phytol. 232 , 1399–1413 (2021).
Singh, B. K., Bardgett, R. D., Smith, P. & Reay, D. S. Microorganisms and climate change: terrestrial feedbacks and mitigation options. Nat. Rev. Microbiol. 8 , 779–790 (2010). This review discusses microbial controls of terretrial greenhouse gas emissions and highlights the importance of soil microorganisms for climate change mitigation.
Delgado-Baquerizo, M. et al. The proportion of soil-borne pathogens increases with warming at the global scale. Nat. Clim. Chang. 10 , 550–554 (2020). This study shows how elevated temperatures can increase the abundance of soil-borne plant pathogens, highlighting that climate change can lead to further prevalence of plant diseases.
Romero, F. et al. Humidity and high temperature are important for predicting fungal disease outbreaks worldwide. N. Phytol. https://doi.org/10.1111/NPH.17340 (2021).
Trivedi, P., Mattupalli, C., Eversole, K. & Leach, J. E. Enabling sustainable agriculture through understanding and enhancement of microbiomes. N. Phytol. 230 , 2129–2147 (2021).
Zhou, Z., Wang, C. & Luo, Y. Meta-analysis of the impacts of global change factors on soil microbial diversity and functionality. Nat. Commun. 11 , 1–10 (2020).
CAS Google Scholar
Jousset, A. et al. Where less may be more: how the rare biosphere pulls ecosystems strings. ISME J. 11 , 853–862 (2017).
Chen, Q. L. et al. Rare microbial taxa as the major drivers of ecosystem multifunctionality in long-term fertilized soils. Soil Biol. Biochem. 141 , 107686 (2020).
Persson, L. et al. Outside the safe operating space of the planetary boundary for novel entities. Environ. Sci. Technol. 56 , 1510–1521 (2022).
Larsson, D. G. J. & Flach, C. F. Antibiotic resistance in the environment. Nat. Rev. Microbiol. 20 , 257–269 (2021).
Kahn, L. H. Antimicrobial resistance: a one health perspective. Trans. R. Soc. Trop. Med. Hyg. 111 , 255–260 (2017).
Hofer, U. The cost of antimicrobial resistance. Nat. Rev. Microbiol. 17 , 3 (2018).
McEwen, S. & Collignon, P. Antimicrobial resistance: a one health perspective. Microbiol. Spectr . 6 , 1–26 (2018).
Zhang, Y. J. et al. Transfer of antibiotic resistance from manure-amended soils to vegetable microbiomes. Environ. Int. 130 , 104912 (2019).
Bahram, M. et al. Structure and function of the global topsoil microbiome. Nature https://doi.org/10.1038/s41586-018-0386-6 (2018). This study provides a global estimate of the structure and function of soil bacteria and fungi.
Matson, P. A. A., Parton, W. J. J., Power, A. G. G. & Swift, M. J. J. Agricultural intensification and ecosystem properties. Science 277 , 504–509 (1997).
Babin, D., Leoni, C., Neal, A. L., Sessitsch, A. & Smalla, K. Editorial to the thematic topic “towards a more sustainable agriculture through managing soil microbiomes”. FEMS Microbiol. Ecol. 97 , fiab094 (2021).
Tilman, D., Cassman, K. G., Matson, P. A., Naylor, R. & Polasky, S. Agricultural sustainability and intensive production practices. Nature 418 , 671–677 (2002). This excellent review shows how the use of chemical fertilizers and pesticides increased with agricultural production over seven decades.
Oehl, F. et al. Impact of land use intensity on the species diversity of arbuscular mycorrhizal fungi in agroecosystems of Central Europe. Appl. Environ. Microbiol. 69 , 2816–2824 (2003).
Tsiafouli, M. A. et al. Intensive agriculture reduces soil biodiversity across Europe. Glob. Chang. Biol. 21 , 973–985 (2015).
Verbruggen, E. et al. Positive effects of organic farming on below-ground mutualists: of mycorrhizal fungal comparison in agricultural communities soils. N. Phytol. 186 , 968–979 (2010).
Gossner, M. M. et al. Land-use intensification causes multitrophic homogenization of grassland communities. Nature 540 , 266–269 (2016).
Gámez-Virués, S. et al. Landscape simplification filters species traits and drives biotic homogenization. Nat. Commun. 6 , 1–8 (2015).
Wittwer, R. A. et al. Organic and conservation agriculture promote ecosystem multifunctionality. Sci. Adv. 7 , eabg6995 (2021).
Fenner, K., Canonica, S., Wackett, L. P. & Elsner, M. Evaluating pesticide degradation in the environment: blind spots and emerging opportunities. Science 341 , 752–758 (2013).
Silva, V. et al. Pesticide residues in European agricultural soils — a hidden reality unfolded. Sci. Total Environ. 653 , 1532–1545 (2019).
Riedo, J. et al. Widespread occurrence of pesticides in organically managed agricultural soils — the ghost of a conventional agricultural past? Environ. Sci. Technol. 55 , 2919–2928 (2021).
Delgado-Baquerizo, M. et al. Multiple elements of soil biodiversity drive ecosystem functions across biomes. Nat. Ecol. Evol. 4 , 210–220 (2020).
Chen, T. et al. A plant genetic network for preventing dysbiosis in the phyllosphere. Nature 580 , 653–657 (2020).
Turnbaugh, P. J. et al. An obesity-associated gut microbiome with increased capacity for energy harvest. Nature 444 , 1027–1031 (2006). This article shows the link between gut microbiota and obesity in humans.
Breidenbach, A. et al. Microbial functional changes mark irreversible course of Tibetan grassland degradation. Nat. Commun. 13 , 1–10 (2022).
Hu, W. et al. Aridity-driven shift in biodiversity–soil multifunctionality relationships. Nat. Commun. 12 , 1–15 (2021).
Delgado-Baquerizo, M. et al. Global homogenization of the structure and function in the soil microbiome of urban greenspaces. Sci. Adv. 7 , eabg5809 (2021).
Van Boeckel, T. P. et al. Global trends in antimicrobial use in food animals. Proc. Natl Acad. Sci. USA 112 , 5649–5654 (2015).
Starr, E. P. et al. Stable-isotope-informed, genome-resolved metagenomics uncovers potential cross-kingdom interactions in rhizosphere soil. mSphere 6 , e00085-21 (2021).
Trubl, G. et al. Soil viruses are underexplored players in ecosystem carbon processing. mSystems 3 , e00076-18 (2018).
Williamson, K. E., Fuhrmann, J. J., Wommack, K. E. & Radosevich, M. Viruses in soil ecosystems: an unknown quantity within an unexplored territory. Annu. Rev. Virol. 4 , 201–219 (2017).
Flandroy, L. et al. The impact of human activities and lifestyles on the interlinked microbiota and health of humans and of ecosystems. Sci. Total Environ. 627 , 1018–1038 (2018).
Tang, T. et al. Antibiotics increased host insecticide susceptibility via collapsed bacterial symbionts reducing detoxification metabolism in the brown planthopper, Nilaparvata lugens . J. Pest. Sci. 94 , 757–767 (2021).
Ryan, M. J. et al. Development of microbiome biobanks — challenges and opportunities. Trends Microbiol. 29 , 89–92 (2021).
FAO, ITPS, GSBI, SCBD & EC. State of Knowledge of Soil Biodiversity - Status, challenges and potentialities (FAO, 2020).
Jones, C. M. et al. Recently identified microbial guild mediates soil N 2 O sink capacity. Nat. Clim. Chang. 4 , 801–805 (2014).
Naylor, D. et al. Soil microbiomes under climate change and implications for carbon cycling. Annu. Rev. Environ. Resour. 45 , 29–59 (2020).
Banerjee, S. & Siciliano, S. D. Spatially tripartite interactions of denitrifiers in arctic ecosystems: activities, functional groups and soil resources. Environ. Microbiol. 14 , 2601–2613 (2012).
Six, J., Elliott, E. T., Paustian, K. & Doran, J. W. Aggregation and soil organic matter accumulation in cultivated and native grassland soils. Soil Sci. Soc. Am. J. 62 , 1367–1377 (1998).
Anthony, M. A., Crowther, T. W., Maynard, D. S., van den Hoogen, J. & Averill, C. Distinct assembly processes and microbial communities constrain soil organic carbon formation. One Earth 2 , 349–360 (2020).
Wei, Z. et al. Trophic network architecture of root-associated bacterial communities determines pathogen invasion and plant health. Nat. Commun. 6 , 1–9 (2015).
Harman, G. E., Howell, C. R., Viterbo, A., Chet, I. & Lorito, M. Trichoderma species — opportunistic, avirulent plant symbionts. Nat. Rev. Microbiol. 2 , 43–56 (2004).
Saleem, M., Arshad, M., Hussain, S. & Bhatti, A. S. Perspective of plant growth promoting rhizobacteria (PGPR) containing ACC deaminase in stress agriculture. J. Ind. Microbiol. Biotechnol. 34 , 635–648 (2007).
Morris, W. E., Uzal, F. A., Fattorini, F. R. & Terzolo, H. Malignant oedema associated with blood-sampling in sheep. Aust. Vet. J. 80 , 280–281 (2002).
Zhu, T. et al. Bacterivore nematodes stimulate soil gross N transformation rates depending on their species. Biol. Fertil. Soils 54 , 107–118 (2018).
Edwards, A. A., Mathura, C. B. & Edwards, C. H. Effects of maternal geophagia on infant and juvenile rats. J. Natl Med. Assoc. 75 , 895–902 (1983).
Gibbs, E. P. J. The evolution of One Health: a decade of progress and challenges for the future. Vet. Rec. 174 , 85–91 (2014).
Dukes, T. W. That other branch of medicine: an historiography of veterinary medicine from a Canadian perspective. Can. Bull. Med. Hist. 17 , 229–243 (2000).
World Health Organization. Constitution. WHO https://www.who.int/about/governance/constitution (2021).
Zegeye, E. K. et al. Selection, succession, and stabilization of soil microbial consortia. mSystems 4 , e00055-19 (2019).
Hooper, D. U. et al. Effects of biodiversity on ecosystem functioning: a consensus of current knowledge. Ecol. Monogr. 75 , 3–35 (2005).
Banerjee, S., Schlaeppi, K. & van der Heijden, M. G. A. Keystone taxa as drivers of microbiome structure and functioning. Nat. Rev. Microbiol. 16 , 567–576 (2018). This commentary article highlights how keystone taxa can determine microbiome complexity and functioning. It also discusses the factors that determine their activities.
Nuñez, M. A., Horton, T. R. & Simberloff, D. Lack of belowground mutualisms hinders Pinaceae invasions. Ecology 90 , 2352–2359 (2009).
Scheffer, M., Carpenter, S., Foley, J. A., Folke, C. & Walker, B. Catastrophic shifts in ecosystems. Nature 413 , 591–596 (2001).
Clemmensen, K. E. et al. A tipping point in carbon storage when forest expands into tundra is related to mycorrhizal recycling of nitrogen. Ecol. Lett. 24 , 1193–1204 (2021). This study shows that the role of mycorrhizal fungi in tundra soil carbon stocks is linked to a tipping point.
Gilbert, J. A. et al. Current understanding of the human microbiome. Nat. Med. 24 , 392–400 (2018). This review provides an assessment of the human microbiome and challenges in our understanding.
Download references
Author information
Authors and affiliations.
Department of Microbiological Sciences, North Dakota State University, Fargo, ND, USA
- Samiran Banerjee
Plant–Soil Interactions Group, Agroscope, Zurich, Switzerland
- Marcel G. A. van der Heijden
Department of Plant and Microbial Biology, University of Zurich, Zurich, Switzerland
You can also search for this author in PubMed Google Scholar
Contributions
The authors contributed equally to all aspects of the article.
Corresponding authors
Correspondence to Samiran Banerjee or Marcel G. A. van der Heijden .
Ethics declarations
Competing interests.
The authors declare no competing interests.
Peer review
Peer review information.
Nature Reviews Microbiology thanks Heribert Hirt, Pankaj Trivedi and the other, anonymous, reviewer(s) for their contribution to the peer review of this work.
Additional information
Publisher’s note.
Springer Nature remains neutral with regard to jurisdictional claims in published maps and institutional affiliations.
Related links
FAO One Health: https://www.fao.org/one-health/
One Health Commission: https://www.onehealthcommission.org/
WHO One Health: https://www.who.int/news-room/questions-and-answers/item/one-health
A characteristic microbial community occupying a reasonably well-defined habitat which has distinct physio-chemical properties. Thus, the microbiome is holistically defined as the microorganisms and their structural elements including nucleic acids, proteins, lipids, polysaccharides as well as various metabolites. Microbiomes also encompass microorganisms and their activities, including their spatiotemporal dynamics, which results in the formation of specific ecological niches.
An imbalance of microbiome structure and composition that is caused by host/environmental perturbations. It is usually associated with loss of taxonomic and/or functional diversity.
The number, relative abundance and composition of different microbial taxa present at a particular location. Thus, microbial diversity is a measure of microbial variation at the taxonomic, genetic, phylogenetic, functional and ecosystem levels. An optimal index should incorporate both richness and evenness.
The ability of a microbiome to withstand a perturbation and remain unchanged in terms of community structure and composition.
Factors related to soil properties.
Natural or anthropogenic factors that are affecting environments globally.
A decline in the differences between ecosystems owing to external factors often resulting in reduced diversity and dominance of certain microbial groups.
Critical points that may occur owing to a single or a series of environmental perturbations and may either lead to dysbiosis or an alternative stable or healthy state.
A healthy and stable state of microbiota with high diversity and abundance of commensals.
An important trait of microbiome stability whereby some taxa are functionally replaceable as other groups can continue their functions.
The ability of a microbiome to endure a perturbation and return to a healthy state despite encountering initial changes in structure and composition.
In this hypothesis, biodiversity insures ecosystems against perturbations and decline in functioning, as a diverse community guarantees that some groups will maintain functioning in the event that other groups fail.
A ‘healthy’ state that may occur owing to resilience in which the structure and composition of a microbiome are different from that of the original healthy state and yet the microbiome may continue to perform the same functions.
Rights and permissions
Springer Nature or its licensor holds exclusive rights to this article under a publishing agreement with the author(s) or other rightsholder(s); author self-archiving of the accepted manuscript version of this article is solely governed by the terms of such publishing agreement and applicable law.
Reprints and permissions
About this article
Cite this article.
Banerjee, S., van der Heijden, M.G.A. Soil microbiomes and one health. Nat Rev Microbiol 21 , 6–20 (2023). https://doi.org/10.1038/s41579-022-00779-w
Download citation
Accepted : 18 July 2022
Published : 23 August 2022
Issue Date : January 2023
DOI : https://doi.org/10.1038/s41579-022-00779-w
Share this article
Anyone you share the following link with will be able to read this content:
Sorry, a shareable link is not currently available for this article.
Provided by the Springer Nature SharedIt content-sharing initiative
This article is cited by
Arbuscular mycorrhizal fungi and streptomyces: brothers in arms to shape the structure and function of the hyphosphere microbiome in the early stage of interaction.
- Feiyan Jiang
Microbiome (2024)
Nutrient availability and plant phenological stage influence the substrate microbiome in container-grown Impatiens walleriana ‘Xtreme Red’
- Juan Quijia Pillajo
- Laura J. Chapin
- Michelle L. Jones
BMC Plant Biology (2024)
Plant growth promotion and biocontrol properties of a synthetic community in the control of apple disease
- Rongye Qiao
- Mingzhen Xu
- Zhiquan Mao
Niche availability and competitive loss by facilitation control proliferation of bacterial strains intended for soil microbiome interventions
- Senka Čaušević
- Manupriyam Dubey
- Jan Roelof van der Meer
Nature Communications (2024)
Biotic homogenization, lower soil fungal diversity and fewer rare taxa in arable soils across Europe
Quick links.
- Explore articles by subject
- Guide to authors
- Editorial policies
Sign up for the Nature Briefing newsletter — what matters in science, free to your inbox daily.


IMAGES
COMMENTS
The concepts also differ in the view of soil rights and assessments. Soil health encompasses a broad range of ecosystem functions, services and actors, impacting a wide array of sustainability ...
The burgeoning broad public interest in the soil health concept is largely grounded in historical development. Even though the term 'soil health' has been more regularly used in the scientific and popular literature only since the early 2000s 107,108,109, the analogy of the soil ecosystem to an organism reaches far into the past.Soil is frequently part of creation myths 110 and humans have ...
In this paper, we discuss a new term " Soil Health Gap" that is defined as the difference between soil health in an undisturbed native soil and current soil health in a cropland in a given agroecosystem. Soil Health Gap can be determined based on a general or specific soil property such as soil carbon.
The major indicators used to assess soil health have been categorized into three groups: physical, chemical, and biological (Moebius-Clune et al., 2016).Fig. 4 shows a simplified overview of select soil health indicators, where a measure of the water availability spans in the physical domain as it is influenced by texture, bulk density, and aggregation, nutrient availability in the chemical ...
This paper reviews the recent advances of the scientific soil health system. The current understanding and interpretation of soil health from the perspectives of soil functions, processes, and ...
A recent review paper discussed the differences between the widely used terms "soil quality" and "soil health", concluding that these terms can be considered equivalent and thus can be used interchangeably (Bünemann et al., 2018). In this review, we opted to use the term soil health.
The database, named SoilHealthDB, currently focuses on four main conservation management methods: cover crops, no-tillage, agro-forestry systems, and organic farming. These studies represent 354 ...
for soil health research and management. Soil- health and soil- ecosystem services Soils provide multiple ecosystem services (Fig. 2) and, as such, ...
The crucial role of healthy soil in achieving sustainable food production and environment is increasingly recognized, as is the importance of proper assessment of soil quality. We introduce a new framework, open soil index (OSI), which integrally evaluates soil health of agricultural fields and provides recommendation for farming practices. The OSI is an open-source modular framework in which ...
This study reports on results obtained within TUdi, the European Union's Horizon 2020 Research and Innovation action under grant agreement No. 101000224. Data on soil health issues and discussions with farmers in Czechia were provided within project No. QK22010261 (Use of new soil protection technologies in agricultural practice).
In the past decade soil health has been intensively studied as a science and practiced as a means to help improve the global social, environmental, and economic sustainability. This paper reviews the recent advances of the scientific soil health system. The current understanding and interpretation of soil health from the perspectives of soil functions, processes, and properties is summarized.
It has proved a powerful tool in conveying best practice to lay audiences, and is increasingly found in international fora, strategic programmes (e.g. Soil Health and Food Mission, the Soil Health Institute, the Soil Biology and Soil Health Partnership, UK 25 Year Environment Plan), agreements and long term plans (Jian et al., 2020).
One is that the idea of human health is already implicit in widely accepted definitions of soil health, 277 and the connection between soil health and human health is already documented. 19,204 Commonly used soil health definitions also incorporate the concepts of improving air and water quality, and these are goals that already enjoy ...
Crop Protection Innovation Should Capitalize on the Role of Soil in Pest Regulation. The functioning of soil biological communities is a key feature of soil health (Lehmann et al., 2020).Soil-based agroecosystem services important for crop health, like pest management and nutrient cycling, depend on biological interaction (Delgado-Baquerizo et al., 2020).
The soil properties of each sample were collected using the Comprehensive Assessment of Soil Health (CASH) framework (Table S1), which uses biological (soil organic matter, respiration, ACE ...
A variety of in-tervention measures have been proposed to enhance soil's ecosystem service in protecting human health. For exam-ple, foliar spray with nano- silicon and nano- selenium can effectively reduce Cd uptake by rice (Deng et al., 2021). Ecological restoration was used to reduce the mobility and health risk associated with cadmium and ...
Cropping systems, including crop diversification, crop rotation and intercropping, and related agronomic practices used in agriculture impact soil health and quality from various spatial and temporal aspects (Vukicevich et al., 2016).Cropping systems were initially designed to maximize yield from agro-systems, but modern agriculture has become increasingly concerned about the environmental ...
Soil health scores for regenerative farms ranged from 11 to 30 (mean = 20). Those for the conventional farms ranged from 3 to 14 (mean = 8). In terms of individual farm pairs, the regenerative farms were consistently higher, with up to 5 times more soil organic matter (mean = 2.0), and up to 7 times higher soil health scores (mean = 3.3) (Fig ...
Soil health is an old concept receiving renewed attention. Defined as a soil's capacity to function, soil health is composed of physical, chemical, and biological attributes. The improvement and maintenance of soil health is considered a cornerstone of organic agriculture. Although there are numerous studies that compare organic systems with conventional systems, fewer studies compare ...
The outcomes of soil use and management decisions are reflected in soil health (Doran and Safley, 1997). Assessing soil health involves the selection of indicators, quantification or qualitative scoring, and providing a final index with appropriate weighting and integration (Rinot et al., 2019). Biophysical indicators are particularly relevant ...
PDF | On Jan 1, 2012, Joyalata Laishram and others published Soil quality and soil health: A review. | Find, read and cite all the research you need on ResearchGate
1 Central Agricultural University, Imphal, India; 2 Division of Agronomy, Indian Council of Agricultural Research-Indian Agricultural Research Institute, New Delhi, India; Soil is an important natural resource providing water, nutrient, and mechanical support for plant growth. In agroecosystem, continuous manipulation of soil is going on due to addition of input, removal of nutrients, changing ...
A range of studies have revealed that soil microbial diversity is positively linked to various components of one health, including aspects of soil 79, plant 20 and ecosystem 146 health. The ...