IEEE Account
- Change Username/Password
- Update Address

Purchase Details
- Payment Options
- Order History
- View Purchased Documents
Profile Information
- Communications Preferences
- Profession and Education
- Technical Interests
- US & Canada: +1 800 678 4333
- Worldwide: +1 732 981 0060
- Contact & Support
- About IEEE Xplore
- Accessibility
- Terms of Use
- Nondiscrimination Policy
- Privacy & Opting Out of Cookies
A not-for-profit organization, IEEE is the world's largest technical professional organization dedicated to advancing technology for the benefit of humanity. © Copyright 2024 IEEE - All rights reserved. Use of this web site signifies your agreement to the terms and conditions.
- Search Menu
- Sign in through your institution
- Advance Articles
- Author Guidelines
- Open Access Options
- Self-Archiving Policy
- About International Journal of Low-Carbon Technologies
- Editorial Board
- Advertising and Corporate Services
- Journals Career Network
- Dispatch Dates
- Journals on Oxford Academic
- Books on Oxford Academic

Article Contents
1. introduction, 2. systematic literature review: methods and materials, 3. results of the bibliographic analysis of data, 4. applications of nanotechnology in ev, 5. summary of key research findings, 6. conclusions, declaration of competing interest, acknowledgements, author contributions.
- < Previous
A systematic review of nanotechnology for electric vehicles battery

- Article contents
- Figures & tables
- Supplementary Data
Pulkit Kumar, Harpreet Kaur Channi, Atul Babbar, Raman Kumar, Javed Khan Bhutto, T M Yunus Khan, Abhijit Bhowmik, Abdul Razak, Anteneh Wogasso Wodajo, A systematic review of nanotechnology for electric vehicles battery, International Journal of Low-Carbon Technologies , Volume 19, 2024, Pages 747–765, https://doi.org/10.1093/ijlct/ctae029
- Permissions Icon Permissions
Nanotechnology has increased electric vehicle (EV) battery production, efficiency and use. Nanotechnology is explored in this electric car battery illustration. Nanoscale materials and topologies research has increased battery energy density, charge time and cycle life. Nanotubes, graphene and metal oxides improve energy storage, flow and charging/discharge. Solid-state and lithium-air high-energy batteries are safer, more energy dense and more stable using nanoscale catalysts. Nanotechnology improves battery parts. Nanostructured fluids reduce lithium dendrite, improving batteries. Nanocoating electrodes may reduce damage and extend battery life. Nanotechnology benefits the planet. Nanomaterials allow battery parts to employ ordinary, safe materials instead of rare, harmful ones. Nanotechnology promotes battery recycling, reducing waste. Change does not influence stable, cost-effective or scalable items. Business opportunities for nanotechnology-based EV batteries need more research. High-performance, robust and environmentally friendly batteries might make electric cars more popular and transportation more sustainable with research and development. An outline of EV battery nanotechnology researchexamines the publication patterns, notable articles, collaborators and contributions. This issue was researched extensively, indicating interest. Research focuses on anode materials, energy storage and battery performance. A research landscape assessment demonstrates EV battery nanotechnology’s growth and future. A comprehensive literature review examined nanosensors in EVs. Our study provides a solid foundation for understanding the current state of research, identifying major trends and discovering nanotechnology breakthroughs in EV sensors by carefully reviewing, characterizing and rating important papers.
The future of nanotechnology with electric vehicles (EVs) is uncertain. Researchers and engineers use nano-manipulating materials to boost EVs’ speed, efficiency and longevity [ 1 ]. Nanotechnology makes coatings for EVs, battery technology, energy harvesting, sensors, catalysis and lightweight materials possible [ 2 ]. By enhancing energy storage, charging speed, component weight and durability, nanotechnology makes electric automobiles more efficient and practical, which could help circumvent transportation system limitations. A cleaner, greener and more sustainable transportation future will be ushered in as nanotechnology improves the capabilities of electric automobiles and increases their widespread acceptability. Nanotechnology’s quick growth has generated exciting potential for the EV revolution [ 3 ]. The use of nanotechnology can improve the performance, efficiency and longevity of EVs. Battery technology, lightweight materials, energy harvesting, sensors, catalysis and coatings are all examples of applications where scientists and engineers have taken advantage of nanoparticles’ unique capabilities [ 4 ]. The batteries used in EVs are improving because of nanotechnology [ 5 ].
Graphene and carbon nanotubes are two examples of nanoscale materials that improve energy storage, electrode surface area and electrode conductivity. The lightweight materials made possible by nanotechnology may also impact EV design [ 6 ]. Nanomaterial composites, including carbon nanotubes and nanofibers, have superior strength-to-weight ratios. Lightening the load improves the vehicle’s mileage, range and handling. EVs use nanotechnology for energy collection. Nanogenerators convert the kinetic energy produced by the car’s motion and vibrations into electrical power. Nanostructured solar panels on the vehicle’s surface capture sun energy for EV charging [ 7 ].
Nanotechnology allows for the development sophisticated sensors that keep tabs on and control the operation of EVs. The susceptible and accurate nanosensors can measure temperature, pressure and gas concentrations, increase safety, give better battery management and enhance vehicle performance. Catalysis and fuel cell technologies, which provide alternatives to EV batteries, rely heavily on nanotechnology [ 8 ]. Using nanocatalysts, fuel cells can convert energy more cleanly. Fuel cells are an option for EVs because of their longer ranges and shorter recharging times [ 9 ]. Intelligent coatings and self-repairing materials can be produced via nanotechnology for EVs. Scratches, corrosion and weathering are all thwarted by nanocoating, and the coating’s self-healing properties repair minor damage [ 10 ]. These coatings’ durability, hydrophobicity and UV resistance increase EVs’ aesthetics, longevity and sustainability. As progress in nanotechnology advances, the outlook for EVs improves. Nanotechnology can make transportation systems more effective, practical and environmentally friendly [ 11 ]. Figure 1 shows the few primary ways nanotechnology can be applied to EVs. Cleaner, greener transportation may lie in the hands of EVs that utilize nanoparticles and nanoscale engineering.

Nanotechnology in EVs.
To further explore how EV batteries might be improved, it is vital to provide a more comprehensive explanation of the specific roles played by nanotubes, graphene, metal oxides and nanoscale catalysts. Nanomaterials are crucial in enhancing different performance characteristics of EV batteries [ 12 ]. The remarkable conductivity features of nanotubes and graphene enable efficient electron transit, hence increasing the overall conductivity of the battery. Metal oxides enhance batteries’ energy storage capacity, improving their efficiency in storing and releasing energy during charge and discharge processes [ 13 ]. In addition, catalysts at the nanoscale operate as promoters to enhance the speed of reaction kinetics, optimizing the charge and discharge efficiency. Furthermore, integrating these nanomaterials improves EV batteries’ security and durability by addressing concerns regarding excessive heat generation [ 14 ] and establishing a more substantial structural foundation [ 15 ]. An in-depth investigation into the unique effects of each nanomaterial on energy storage [ 16 ], conductivity, charge/discharge processes, safety and stability issues offers a detailed comprehension of their combined influence on the advancement of EV battery technology [ 17 ].
Nanotechnology has become a major force in improving the performance and economy of batteries for EVs. Materials like nanotubes, graphene, metal oxides and nanoscale catalysts play key roles in this. Nanotubes and graphene are great for storing energy because they are highly conductive and have a lot of surface area [ 15 ]. This makes them better electrode materials for lithium-ion batteries, which are widely used in EVs. These materials make it easier for electrons and ions to move faster, which leads to more energy and faster charging and discharging. On the other hand, metal oxides are great alternatives to standard cathode materials because they have higher capacity and better cycling stability. Their nanoscale size makes charge transfer even more efficient, which lowers the energy lost during cycles [ 18 ]. Graphene is a single layer of carbon atoms grouped in a hexagonal lattice. It is very good at conducting electricity and is also very strong. It makes the conductivity better when added to battery electrodes, which speeds up the charging and discharging processes. Nanotubes, like carbon nanotubes, have a lot of surface area and can carry electricity well. This has helped make electrode materials that are light and work well. Metal oxides, such as manganese oxide and titanium oxide, have better electrochemical qualities [ 19 ]. This meets the need for materials in EV batteries that have a higher energy density and last longer between cycles.
Moreover, tiny catalysts have completely changed the electrochemical processes that happen inside batteries. These helpers, which are usually made of precious metals like platinum and palladium, make the processes that reduce and release oxygen easier in fuel cells and metal–air batteries. This makes them work better overall. Nanostructuring these catalysts also makes them more active, which means they can convert and store energy more efficiently. When it comes to safety, nanomaterials help make improved battery systems possible [ 20 ]. Nanoscale coatings and additives can make lithium-ion batteries safer by making them more stable at high temperatures, lowering the risk of burning, and stopping dendrites from forming. Nanomaterials also make things more stable, which means batteries last longer. This means they don’t need to be replaced as often, and throwing away batteries has less of an effect on the world [ 21 ]. According to the researchers, adding nanotubes, graphene, metal oxides and tiny catalysts to the design and make-up of EV batteries is a big step forward for making them safer, more stable and better at storing energy and conducting electricity. As scientists continue to study and improve these nanotechnology uses, the chances of making electric transportation more efficient and environmentally friendly grow [ 22 ].
1.1. Importance of nanosensors
Sanguesa et al. (2021) reviewed EV battery technological trends, charging techniques and future research challenges and prospects. They discussed the pros and cons of lead-acid, nickel-metal hydride, lithium-ion and lithium-sulfur EV batteries. They also discussed EV charging standards and modes such as AC and DC, slow and rapid, wireless and bidirectional. They discussed nanosensors’ high sensitivity, low power consumption, miniaturization, fabrication, integration and calibration concerns for battery management [ 23 ]. A literature overview on EV technology and its various uses, including smart grid, vehicle to grid, and vehicle to home, was presented. Energy efficiency, emissions reduction, grid stability, battery cost, range anxiety and charging infrastructure were only some of the topics covered. Several global EV programs and projects were highlighted, including Tesla, Nissan Leaf and Dubai Smart City [ 24 ]. A systematic literature review (SLR) on EV consumer acceptance was published by Han et al. [ 25 ]. They synthesized the methodology, ideas and variables from 57 peer-reviewed publications published between 2015 and 2022 on EV purchase, behavior and usage intentions. They found that attitudes, norms, perceived behavioral control, awareness, knowledge, personal values, emotions and social influence affect EV adoption. They also highlighted literature gaps and suggested additional research [ 25 ]. The authors of Basu et al. (2018) provided a synopsis of EVs and EV sensors. The advantages and disadvantages of the various varieties of EVs, including fuel cell EVs (FCEVs), battery EVs (BEVs) and hybrid EVs (HEVs), were discussed. Additionally, they deliberated on the evolving landscape of micro-electro-mechanical system- (MEMS) based miniaturization for sensors and devices utilized in diverse EV applications and the various types of sensors associated with battery and position monitoring [ 26 ].
1.2. Literature review
The low-carbon mobility transition relies on plug-in EVs, including battery and hybrid EVs. Daramy-Williams et al. (2019) found many user experience topics, including driving and travel behaviors, vehicle interactions and subjective factors. EVs have increased the utilization of electric batteries. Many studies have developed and improved battery cell voltage equalization methods by adding noteworthy features [ 27 ]. These cell equalization techniques were the subject of a thorough and methodical review (Das et al., 2020). This SLR focuses on recent efforts that have built energy management storage system (EMSs) for HEVs [ 28 ]. The study conducted by Torreglosa et al. (2020) aimed to provide a quantitative analysis of the chosen works. Notwithstanding advancements in driving range and recharge alternatives, these and additional market impediments persist, rendering the present market share of BEVs inconsequential [ 29 ]. In light of the advancement of hydrogen fuel cell stacks, an emerging powertrain architecture concept for N1 class-type cars was described (Castillo et al., 2020). The fuel cell extended range electric vehicles (FC-EREV)concept combines a battery-electric arrangement with a hydrogen-powered fuel cell stack that acts as a range extender. The lithium-ion battery in EVs can sustain an operational temperature range of 15°C to 35°C employing a battery thermal management system (BTMS) [ 30 ].
Tete et al. (2021) conducted a comprehensive review of experimental and numerical analyses of BTMS utilized in electric and hybrid vehicles. This review encompassed approaches such as air, liquid, phase change material, heat pipe and refrigeration cooling for battery cooling systems. EVs need high-energy batteries. The maximum capacity of lithium-air battery theory using graphene under optimal electron conduction conditions and the experimental maximum obtained by optimizing the structure geometry, examples of structural engineering using carbon fiber and carbon nanotubes in cathode fabrication to perform the reaction properly while providing space for lithium oxide placement, are examined [ 31 ]. Suryatna et al. (2022) described the battery’s mechanism and analyze its constituent parts. As a result of the growth of green logistics and the support of new energy car development policies both domestically and globally, logistics and distribution have embraced EVs as an alternative to traditional fuel vehicles [ 32 ]. Ye et al. (2022) examined the most recent breakthroughs in EV routing models and solution algorithms in logistics and distribution. Passenger vehicles contribute significantly to glasshouse gas (GHG) emissions; thus, precise and current estimates of the comparative emissions of the key types of alternative power trains are essential to support evidence-based policy recommendations [ 33 ]. A systematic review and harmonization of the most recent scientific literature on this subject was presented in Raugei (2022). The findings show that battery BEVs are the most promising option for decarbonizing the passenger vehicle fleet across all global regions studied, with the potential for −70% reductions in GHG emissions compared to conventional gasoline-powered internal combustion engine vehicles. EVs are becoming increasingly prevalent as many nations set net-zero carbon targets for the foreseeable future [ 34 ].
The status, characteristics and application scope of global lithium ion battery (LIB) safety standards and regulations were examined by Lai et al. (2022). The rational test standard upgrade is reviewed in light of recent EV and energy storage power plant fires. Direct drive offers improved systematic economy, more flexible wheel control and better passenger comfort by eliminating the gearbox and transmission [ 35 ]. Cai et al. (2022) reviewed vehicle direct-drive methods and contemporary electrical machine improvements for direct-drive propulsion systems. FCEVs for long-haul applications can reduce road freight CO 2 emissions until long-distance battery-electric mobility matures, depending on the hydrogen fuel source [ 36 ]. Pardhi et al. (2022) examined FCEV powertrain topologies for long-distance HD applications, as well as their operating constraints, cooling requirements, waste heat recovery methods, cutting-edge powertrain control, energy and thermal management strategies, as well as over-the-air route data-based predictive powertrain management with V2X connectivity. Batteries powering EVs provide a promising way to reduce pollution and uncertainty.
In contrast to battery degeneration’s scalability and temporal constraints, machine learning (ML) approaches offer a non-invasive, accurate and low-processing solution [ 37 ]. Sharma and Bora (2023) evaluated these problems objectively and comprehensively. EVs are becoming mainstream as more governments set near-term net-zero carbon ambitions [ 38 ]. Senol et al. (2023) conducted a comprehensive literature analysis in their study, which focused on integrating power networks and Li-ion battery technologies, particularly emphasizing their performance under unfavorable weather circumstances [ 39 ]. Increasing multiscale modeling and design for battery efficiency and safety management was reviewed by Kiran MD et al. (2024). This article shows how machine learning-based data analysis in battery research has advanced, setting the groundwork for cloud and digital battery management to produce trustworthy onboard applications [ 40 ]. Electron and ion transport affect the battery’s energy production under application conditions and how much energy can be used [ 41 ]. The transport mechanisms of ions and electrons for active materials, in addition to positive and negative composite electrodes, were examined by Quilty et al. (2023). Simultaneously, contemporary EVs exhibit a confident capacity to reduce fossil fuel consumption [ 42 ]. Gevorkov et al. (2023) reviewed and analyzed multiport converters’ key characteristics, topologies, pros and cons and applications. Operando characterization is not new, but techniques that can track commercial battery properties under realistic conditions have unlocked a trove of chemical, thermal and mechanical data that could revolutionize lithium-ion device development and use [ 43 ]. The innovative dual-ion battery that is built on aluminum and has a cathode made of three-dimensional graphene possesses high-energy density, low cost and the ability to charge and discharge faster than other batteries, making it an ideal choice for grid storage and personal gadgets by Zhang et al. (2016) [ 44 ]. A novel calcium-ion battery with a high discharge voltage and 95% capacity retention can function consistently at room temperature. This battery has the potential to serve as an alternative to lithium-ion batteries by Wang et al. (2018) [ 45 ]. For sodium-based energy storage applications, this study conducted by Mu et al. (2020) successfully synthesizes high-performance anodes with high-fraction active materials. These anodes achieve good rate capability and cycling stability [ 46 ]. Zhang et al. (2023) examined the difficulties encountered by power systems that heavily rely on inverter-based resources (IBR), specifically emphasizing the inadequate capacity to support voltage and frequency. The authors suggest reorganizing the virtual synchronous generator (VSG’s) control blocks by aligning the control blocks of a VSG with the control perspective of conventional synchronous generators. The reorganization above streamlines and clarifies the control pathway governing the system’s active and reactive power output, enabling easier virtual inertia and attenuation parameters to be adjusted. The article presents a compact signal model for IBR controlled by VSG, including voltage and current loops modules, instantaneous power calculation and an LC (inductors (L) and capacitors (C)) filter [ 47 ]. A current discrepancy that occurs during charge and discharging in high-temperature superconducting (HTS) non-insulation closed-loop coils may result in novel phenomena, such as a rapid decrease or increase in magnetic field at particular positions, and may influence operational current judgment by Lu et al. (2022) [ 48 ]. Wu et al. (2023) centers on the advancements in rare earth-barium-copper oxides superconducting tapes, with a specific emphasis on the nanocomposite EuBa2Cu3O7-δ superconducting films. These films hold significant importance in the context of high-field magnet applications. The scientists utilize a pulsed laser deposition methodology with an exceedingly rapid growth rate of up to 100 nm/s—two orders of magnitude higher than traditional approaches. This enables them to accomplish both rapid growth and a substantial capacity to transport field current [ 49 ]. Shen et al. (2024) have presented an innovative energy management approach that seeks to optimize the functionality of energy storage systems in EVs, specifically focusing on reducing the aging of lithium-ion batteries caused by high-frequency power requirements. Fuzzy logic control and ensemble empirical mode decomposition are incorporated into the proposed method. At the outset, the power demand of EVs is decomposed into intrinsic mode function components. Subsequently, permutation entropy is utilized to reconstruct each component into low- or high-frequency components [ 50 ]. A proposal was made by Zhao et al. (2023) for the preview-based human-like trajectory planning model (PHTPM), which is subsequently evaluated and assessed through comparative and generalizability tests. The findings indicate that the implementation of the driver preview feature empowers PHTPM to precisely emulate the attributes of proficient drivers during left turns while surpassing them during right turns [ 51 ]. A critical scenario search technique for intelligent vehicle testing that is based on the social cognitive optimization algorithm has been suggested, and the findings show that the proposed method has the potential to increase both the search efficiency, and the coverage of important scenarios was figured out by Zhu et al. (2023) [ 52 ]. This research presents a novel model that has the potential to improve our understanding of the behavior of lithium deposition and pave the way for the development of stable and secure lithium metal anodes through the utilization of bimetallic metal organic framework- (MOF) derived materials in the construction of three-dimensional frameworks studied by Wei et al. (2023) [ 53 ]. Based on the findings of the analysis conducted by Yue et al. (2023), it can be concluded that the stability of the road system in the presence of incident effects is directly connected to the severity of the event, the signal control strategy, the penetration rate and the spatial distribution of autonomous vehicles. In conclusion, simulation results are carried out to demonstrate the efficacy of our suggested event management policy in enhancing the rate of recovery and the stability of road networks [ 54 ]. In order to solve the issue in which the response quality is decreased as a result of factors such as parameter mismatch and disturbance, an adaptive disturbance observer-based improved super-twisting sliding mode control (ISTSMC-ADOB) has been developed. With the purpose of achieving adaptive compensation, avoiding the usage of high-gain feedback, and expanding the applicability of the conventional disturbance observer, an adaptive disturbance observer, also known as an ADOB, was intentionally constructed by Lu et al. (2022) [ 55 ]. Yu et al. (2023) has discussed the difficulties that arise when attempting to implement aqueous zinc-ion batteries in practical applications. The paper focuses on the unstable electrode/electrolyte interface that is related to inhomogeneous zinc deposition and side reactions. The solution that has been offered involves the utilization of L-carnitine (L-CN) as an effective addition for the purpose of stabilizing electrodes and extending the lifespan of batteries. When L-CN is present in minute quantities, it exhibits a remarkable synergy between quaternary ammonium cations, COO− anions and hydroxyl groups. This synergy can influence the electrochemical deposition/insertion of Zn 2+ and the activity of water molecules [ 56 ]. Hou et al. (2023) have centered on developing a self-powered, lightweight biomimetic mouse whisker sensor (BMWS) that draws inspiration from the extraordinarily perceptive whisker detection exhibited by mice. The BMWS, unlike its predecessors that attempted to imitate animal whiskers, surmounts drawbacks, including a cumbersome design, dependence on external power and restricted application scenarios. By utilizing the triboelectric effect and a meticulously engineered framework, the BMWS exhibits exceptional capabilities in detecting collisions and maintaining signal stability. Intelligent early warning, direction identification, hole width discrimination and real-time distance sensing are among the many duties in which it excels [ 57 ]. A novel mechanism and technology for directed energy deposition-arc in the production of large parts, referred to as alternating-arc-based additive manufacturing and enabled by a polarity-switching self-adaptive shunt, are presented Yan et al. (2023). The experimental outcomes demonstrate that the proposed system facilitates the alternating passage of current through the welding wire and the substrate, generating electronegative arcs at the anode with the wire and electropositive arcs at the cathode with the substrate. By modulating the arcs, decoupling control between thermal force and mass transfer is accomplished. The electropositive arc is responsible for cathode cleaning and molten pool temperature, whereas the electronegative arc regulates wire melting, particle size and temperature [ 58 ]. A battery–supercapacitor hybrid energy storage system and an accompanying energy management strategy are proposed in the article by Wang et al. (2024) in a response to the growing need for compact motors with high output torque, specifically for use in mobile robotics. This method draws inspiration from automobiles’ high-capacity hybrid energy systems. In conditions of minimal torque, the motor operates on battery power, with any excess power being directed toward charging the supercapacitor. When faced with torque overload conditions, the motor is supplied with high current by swiftly discharging the supercapacitor, which guarantees an instantaneous increase in output power. The objective of the energy management strategy is to regulate the supercapacitor’s charging and discharging processes to prevent interference with the motor’s power supply from the battery and maintain current stability and control during discharge [ 59 ].

Process of SLR.

Flow chart of selection of data for bibliometric analysis.
The resources utilized in this SLR comprise bibliometric data extracted from various sources, such as journal articles, conference papers, scholarly surveys, books, essays and review articles. These sources are analyzed to determine author affiliations, country collaboration maps, citation patterns, keywords plus and the frequency of author keywords. The present study employs a systematic procedure to select research publications about nanomaterials in EVs. The literature search involved comprehensive exploration using databases like Scopus. Key search terms included ‘nanotechnology in EV batteries,’ ‘electrode nanocoating’ and related variations. Potential biases in article selection were mitigated by employing rigorous inclusion criteria, prioritizing peer-reviewed studies and avoiding undue influence from commercial interests. Figure 2 shows the process of SLR.
The Scopus article research selections were restricted in the first step, as shown in Figure 3 and Table 1 . From 1991 to July 2023, this analysis uncovered 2361 publications concerning EVs, nanotechnology, nanomaterials and sustainable development. We have 2322 articles remaining after eliminating concise surveys, notes, erratum editorials and letters. After publication status-based exclusions of research papers, 2309 remained. The final subjects that were excluded from consideration were the following: astronomy and physics, pharmacology, toxicology, pharmaceutics, earth and planetary science, health profession, agriculture and biological science, neuroscience, immunology, microbiology, business and management, accounting, economics, econometrics and finance, arts and humanities and dentistry. One thousand five hundred forty products were remaining.
Bibliometric analysis is a method that quantifies scientific publications by examining citation patterns. Citations, according to a bibliometric study, reveal the impact, influence and connections of academic journals [ 60 ]. The bibliometric analysis theory posits that scientific knowledge is transmitted through scholarly publications, wherein the number and caliber of citations gauge the scientific significance of an article or publication it garners [ 61 ]. Bibliometric researchers utilize citation data to quantify author output, journal influence, collaboration patterns and research trends. According to this theory, more people cite influential articles [ 62 ].
Additionally, intellectual networks between authors, institutions and fields may be reflected in citations [ 63 , 64 ]. By quantifying these citation patterns, bibliometric analysis evaluates research output, influence and collaboration [ 63 ]. Bibliometric research is regulated by mathematical and statistical models, including co-citation analysis, citation counts, h-index and bibliographic coupling. Academics can quantify and contrast scholastic output, discern notable works and authors and monitor the evolution of specific publications or research domains through these techniques. Citation patterns can disclose the structure and dynamics of scientific knowledge, aiding policymakers, institutions and researchers in making informed decisions regarding funding allocation, research evaluation and scholarly communication, according to bibliometric analysis [ 65 ].
By examining the bibliography, the most cited journals are determined. Energy and environmental science, nanoenergy, ACS Applied Materials and Interfaces and the Journal of Materials Chemistry , all of which have contributed to the study of nanomaterials in EVs, are utilized in the proposed research. Numerous scholarly periodicals indicate that nanomaterials are an emerging trend for energy storage and lightweight materials to reduce the weight of EVs, as demonstrated by this review [ 66 ]. Bibliometric analysis is an expert methodology that assists researchers in assessing the advancement of various approaches through the statistical distribution of data about citation analysis, keywords, author affiliation and country of contribution. The top journals are shown in Table 2 .
Detailed analysis of nanomaterials in EV data is presented in this section. In recent decades, the quantity of publications and literary sources is evaluated in Sections 3.1 and 3.2. Additionally, the country production analysis is presented in Section 3.3. The thematic evaluation of the keywords in the publications is presented in Section 3.4. Additionally, the collaboration map of the top 15 nations, most cited countries, country production over time and source dynamics is provided in Section 3.5. Section 3.6 provides a comprehensive analysis of the findings regarding author keywords, most common terms, a tree map of keywords and word clouds. Section 3.7 presents three field plots depicting the relationships between author keywords, author countries and keywords plus.
3.1. Literature analysis of publications
Figure 4 illustrates the annual distribution of publications from 1991 to 2023. However, upon examining the graph, we have identified a substantial fluctuation in the number of publications on nanomaterials in EVs. The number of publications in this field was ‘0’ before 2003 but rose after 2005. However, between 2012 and 2021, there has been a significant increase in the number of publications devoted to investigations. The calculated annual growth rate was approximately 12.79%.
3.2. Institutions
A visual representation of the collaborations between the five most prestigious institutions worldwide and their publications over the last 30 years is presented in Figure 5 . As stated, five major institutions contribute to the Scopus Journal, with Nanyang Technological University ranking first with 945 publications and Beijing University ranking second with 586 publications. Simultaneously, the University of Science and Technology of China, the School of Material Science and Engineering and Tsinghua University ranked third, fourth and fifth in the Scopus database from 1991 to 2023, with net publications of 528, 475 and 409, respectively.
Main information of data taken from Scopus.
. | . |
---|---|
Timespan | 1991:2023 |
Sources (journals, books, etc.) | 587 |
Documents | 1540 |
Annual growth rate % | 12.79 |
Document average age | 7.62 |
Average citations per doc | 63.84 |
References | 80 178 |
Index keywords or keywords plus | 11 422 |
Author’s keywords | 3371 |
Authors | 5108 |
Authors of single-authored docs | 95 |
Single-authored docs | 99 |
Co-authors per doc | 4.9 |
International co-authorships (%) | 24.42 |
Article | 811 |
Book | 30 |
Book chapter | 117 |
Conference paper | 339 |
Conference review | 20 |
. | . |
---|---|
Timespan | 1991:2023 |
Sources (journals, books, etc.) | 587 |
Documents | 1540 |
Annual growth rate % | 12.79 |
Document average age | 7.62 |
Average citations per doc | 63.84 |
References | 80 178 |
Index keywords or keywords plus | 11 422 |
Author’s keywords | 3371 |
Authors | 5108 |
Authors of single-authored docs | 95 |
Single-authored docs | 99 |
Co-authors per doc | 4.9 |
International co-authorships (%) | 24.42 |
Article | 811 |
Book | 30 |
Book chapter | 117 |
Conference paper | 339 |
Conference review | 20 |
Top 15 journals in the research area under the study
. | . | . |
---|---|---|
1 | 71 | |
2 | 64 | |
3 | 57 | |
4 | 32 | |
5 | 28 | |
6 | 27 | |
7 | 27 | |
8 | 26 | |
9 | 25 | |
10 | 24 | |
11 | 24 | |
12 | 22 | |
13 | 21 | |
14 | 16 | |
15 | 15 |
. | . | . |
---|---|---|
1 | 71 | |
2 | 64 | |
3 | 57 | |
4 | 32 | |
5 | 28 | |
6 | 27 | |
7 | 27 | |
8 | 26 | |
9 | 25 | |
10 | 24 | |
11 | 24 | |
12 | 22 | |
13 | 21 | |
14 | 16 | |
15 | 15 |
3.3. Analysis of countries
Diverse nations have contributed to academic journals concerning the application of nanotechnology to EVs. The cumulative number of publications originates from the 10 countries in Figure 6 . Based on the analysis, China holds the highest position regarding the total number of articles. Following that, India and the United States occupied the second and third positions, respectively, with 1584 and 480 publications, while China produced 2370 articles within the specified period.
3.4. Thematic evaluation of keywords
Based on the evaluation of keywords from 1991 to 2023, as shown in Figure 7 , several authors have repeatedly utilized a small number of keywords. The following terms were examined following the analysis: self-powered sensors, batteries, triboelectric nanogenerator, nanotechnology, renewable energy, lithium-ion batteries, lithium-ion batteries, electrode material and electrochemical performance.

Annual distribution of the publications.

Top 5 productive institutions.

Top 15 countries with the maximum number of publications.

Thematic evaluation of keywords.
3.5. Country collaboration
The collaborative efforts of numerous nations are illustrated in Figure 8 , and Figure 9 shows the cloud-based structure of the various country collaborations. Based on the bibliometric survey’s analysis, the following countries have been identified as having the greatest number of collaborations in the field of nanotechnology for EVs: China, India, the United States, South Korea, Germany, Japan, the United Kingdom, the Philippines, Singapore, France, Australia, Canada, Italy, Spain and Malaysia.

Country collaboration map.

Cloud-based structure of the various country collaborations.
3.6. Keywords
Each node in Figure 10a corresponds to a distinct keyword. We opted to include only the top 50 out of 1000 keywords. The prevailing research topics identified were ‘nanotechnology,’ ‘lithium,’ ‘electrodes,’ ‘electric batteries,’ ‘nanostructured materials’ and ‘energy efficiency.’ A cloud structure of keywords is depicted in Figure 10b , which resembles the analysis in Figure 10a ; however, the distinction between the two figures lies in the quantity of keywords. The authors spent the most time gathering the following 50 keywords for the cloud of keywords: ‘nanotechnology,’ ‘lithium-ion batteries,’ ‘lithium,’ ‘electrodes,’ ‘electric batteries’ and lithium compounds. In contrast, Figure 10c illustrates the tree map structure of keywords as they appear in the publications of various authors.

a. Keyword co-occurrence network. b Keywords cloud of top 50 keywords. c. Tree map of keywords.
3.7. Three-field plot
The three field plots between author keywords, author countries and keywords plus were depicted in Figure 11 . The preceding 20-author keywords, utilized by most authors in their publications, are displayed on the left side of the plot. The author countries to which they belong are then indicated in the middle. The top 20 countries were analyzed, with China, the United States, Korea and India ranking the highest. The concluding segment of the narrative presents keywords plus, which includes the top 20 keywords and those used by the author. Concerning the keywords, this plot is structured to examine the research field and the authors’ collaborative network.

Three field plots between author keywords, author countries and keywords plus.
3.8. Bibliometric analysis challenges
Researchers may encounter several problems and restrictions when using R-Studio Biblioshiny for bibliometric analysis. One big problem is that bibliographic records are very different from one another. This is because different sources may use different formats and terminologies, which makes it hard to combine and standardize data. It might also be hard to ensure the bibliometric study’s accuracy if the data’s quality and completeness vary from database to database [ 67 ]. Another problem is that scientific literature is always changing; new papers are always being added, and databases might not be updated very often, which could mean the results are incomplete or outdated. Also, full-text articles may not always be available or easily accessible, which could affect the study’s completion. Researchers may also have trouble developing relevant search queries because the buzzwords and the criteria they use to include or leave out information can greatly affect the results [ 68 ]. Even with these problems, R-Studio Biblioshiny for bibliometric analysis is still a useful tool that helps researchers get around many of these issues and learn important things about the scholarly world.
Additionally, picking the right bibliometric indicators and measures presents different problems. To measure the effect, output and teamwork, researchers must be meticulous in picking the right factors. If you misinterpret metrics or only use a small group of indicators, you might come to the wrong conclusions about the importance of certain study topics or authors [ 69 ]. Also, because some fields are changing and combining different areas of study, it might be hard to correctly group papers into the right research domains. When doing bibliometric analysis, it is also very important to think about ethics, especially when there are disagreements about who wrote a paper or when someone cites themselves in a paper. To ensure the results are accurate, it is important to balance automated data extraction and human verification [ 70 ]. There may also be problems with how bibliometric analyses can be repeated since different researchers may get different results depending on how they read parameters or how the data sources are changed. Even with these problems and restrictions, R-Studio Biblioshiny has an easy-to-use design and many tools that can help with many of them. As software is constantly updated and made better, some problems may be solved. This makes bibliometric analysis a useful and ever-evolving way to understand the scholarly scene. As the scientific literature changes quickly, researchers should stay alert and deal with these problems to ensure their results are strong and correct [ 71 ].
Nanotechnology is currently leading the way in transforming numerous aspects of EV technology. Nanoscale materials, including carbon nanotubes and graphene, are important in battery technology because they improve electrode conductivity, surface area and energy storage. This leads to enhanced energy density, prolonged battery life and accelerated charging, effectively mitigating apprehensions regarding charging durations and range anxiety [ 72 ]. In addition to batteries, nanomaterials can generate lightweight and robust materials for EV construction. This is demonstrated through the integration of carbon nanotubes and nanofibers into composites. This phenomenon fortifies the structure and positively impacts the vehicle’s energy efficiency, range and overall performance [ 73 ]. Energy harvesting in EVs is further facilitated by nanotechnology, as nanostructured solar panels capture solar radiation, and nanogenerators convert mechanical energy into electrical power, thereby contributing to sustainable electricity generation [ 74 ]. Incorporating nanomaterials into supercapacitors and energy storage improves regenerative braking and power delivery, increasing energy storage and charge–discharge rates [ 75 ]. Nanosensors, renowned for their accuracy and sensitivity, provide advantages to EV control and monitoring systems by facilitating the observation of gas, pressure, temperature and concentrations for enhanced performance and safety.
Furthermore, progress in catalysis and fuel cell technologies is facilitated by nanotechnology, which has the potential to supplant conventional EV batteries and enhance the efficiency of energy conversion [ 76 ]. Intelligent coatings and self-healing materials, enabled by nanotechnology, safeguard EV surfaces against corrosion, weathering and abrasion by forming hydrophobic, UV-resistant and durable coatings that increase longevity. Fundamentally, nanotechnology manifests as a paradigm-shifting influence, initiating an era of enhanced EV performance, sustainability and innovation [ 77 ]. Figure 12 shows nanotechnology’s applications, challenges and future perspectives in EVs.

Nanotechnology for EVs.
4.1. Challenges of nanotechnology in EVs
Although nanotechnology can greatly enhance EVs, it presents considerable obstacles that must be thoughtfully assessed and resolved inventively. The significant challenge of scalability arises from the difficulties associated with mass-producing nanotechnology processes, which hinder the consistent and superior integration of nanomaterials into EV components. The complexities inherent in nanotechnology further complicate issues about scalability, thereby demanding progress in manufacturing methodologies to guarantee extensive implementation [ 78 , 79 ]. Nanotechnology materials and processes are more expensive to manufacture; thus, cost-effectiveness is essential. Nanomaterials in EV batteries and lightweight components may raise production costs, affecting EV affordability and consumer acceptability. Another major challenge is EV nanoparticle and nanostructure stability. Nanomaterials must be durable and reliable to maintain EV performance and safety, especially under harsh operational conditions [ 80 ].
These stability difficulties require continual research and development to improve nanomaterial robustness and compatibility with EV dynamics. In EV nanotechnology integration, environmental concerns are essential [ 81 ]. Nanomaterial manufacturing, use and disposal must meet environmental goals to address health and ecological problems throughout the life cycle of nanotechnology in EVs. Research and collaboration between the scientific and manufacturing sectors aim to overcome these limitations and advance nanotechnology’s use in EVs. Scalability, cost-effectiveness, long-term stability and environmental impact can be addressed to realize nanotechnology’s transformative promise in EVs, enabling sustainable, efficient and widely accessible electric mobility [ 82–84 ].
4.2. Commercial potential of nanotechnology in EV batteries
The discourse on the commercial viability of nanotechnology-enabled EV batteries involves a comprehensive examination of the promising breakthroughs and the obstacles and limitations that hinder their widespread use. Nanotechnology has become a robust and influential factor in energy storage, specifically EV batteries [ 85 ]. These technological developments are crucial for achieving higher performance metrics, including increased energy storage capacity, enhanced conductivity, efficient charge and discharge cycles and enhanced safety and stability. When considering the economic potential of nanotechnology in EV batteries, it is crucial to carefully examine the obstacles that could impede its smooth absorption into the market [ 86 ].
An essential component of this topic revolves around comprehending the market’s preparedness for EV batteries that incorporate nanotechnology. Although the potential advantages are significant, the market must be sufficiently prepared to adopt these technical advancements. Market preparation covers various elements, including establishing infrastructure for manufacturing and distribution, creating regulatory frameworks that can handle emerging technology and promoting consumer awareness and acceptance [ 87 ]. Evaluating the present level of preparedness offers valuable information regarding the timeframe and viability of implementing nanotechnology-enhanced EV batteries on a broader scope. Simultaneously, it is crucial to tackle the obstacles posed by incorporating nanotechnology in EV batteries. The issues encompass a wide spectrum, from intricate technological obstacles to financial constraints. Nanomaterials, including nanotubes, graphene and metal oxides, provide remarkable advancements at the molecular scale. However, their ability to be scaled up and their cost-effectiveness in industrial applications must be thoroughly evaluated. The complexities of production processes, potential environmental consequences and the requirement for specialized knowledge provide problems that require careful and thorough consideration [ 88 ].
Moreover, the conversation should include the possible obstacles to the adoption that could hinder the universal approval of nanotechnology-enabled EV batteries. Barriers can appear in different ways, such as economic, technological and societal issues. Economic factors encompass the aggregate expenses of production, prospective price increases for consumers and the economic viability for producers [ 89 ]. Technological impediments encompass challenges such as the requirement for standardized testing protocols, concerns regarding reliability and compatibility issues with current infrastructure. The success of nanotechnology-enabled EV batteries is heavily influenced by societal variables, including how the public perceives and accepts new technologies. The economic feasibility of EV batteries enhanced by nanotechnology also depends on effectively addressing safety issues and environmental consequences [ 90 ]. Although nanomaterials provide advancements in battery safety and stability, it is crucial to conduct a comprehensive assessment of the potential hazards related to the manufacturing and disposal of nanomaterials. Establishing or modifying regulatory frameworks is necessary to guarantee the secure and conscientious incorporation of nanotechnology into the electric car industry [ 91 ].
Strong competition in the EV sector complicates commercializing nanotechnology-enabled batteries. Given the ongoing advancements in traditional battery technologies and other energy storage solutions, it is crucial to consider the placement of nanotech-enabled batteries in this changing landscape [ 92 ]. An essential aspect of evaluating the commercial success of nanotechnology-enabled EV batteries is comprehending the competitive dynamics, developing distinctive selling factors and distinguishing them from existing technologies. Moreover, the influence of government regulations and incentives in defining the commercial path of nanotechnology-enabled EV batteries should not be underestimated [ 93 ]. Implementing supportive regulations, financial incentives and research funding can greatly expedite the implementation and acceptance of these advanced energy storage options. On the other hand, if there are no well-defined norms or obstacles in regulations, it could impede innovation and discourage investment in the emerging market of nanotechnology-enabled EV batteries [ 94 ].
4.3. Nanoscale breakthroughs boost battery performance
One of the most important factors in improving the overall performance of EV batteries is the adoption of nanoscale enhancements, which are advances on a smaller scale. The essential components of the battery, such as electrodes and catalysts, are subjected to transformations due to using materials such as nanotubes, graphene, metal oxides and nanoscale catalysts [ 95 ]. The nanoscale dimensions of these materials contribute to an increase in surface area, an improvement in conductivity and an enhancement in electrochemical characteristics. Due to these enhancements, the battery’s cycle life is extended, its charge and discharge rates are accelerated and its energy density is increased [ 96 ]. Furthermore, the improvements in nanotechnology also address safety concerns by reducing the dangers of overheating and limiting the creation of dendrites. As a result, the cumulative effect of these small-scale advancements dramatically raises the overall efficiency, reliability and sustainability of EV batteries, paving the way for a more optimistic future in electric transportation [ 86 ].
4.4. Future perspectives of nanotechnology
A comprehensive evaluation of the environmental consequences of incorporating nanomaterials and nanotechnology processes into EVs is imperative. This underscores the criticality of assessing health and environmental risks to ascertain nanotechnology’s feasibility and ecological sustainability in this domain. When considering the future, nanotechnology can fundamentally transform numerous facets of EV technology [ 97 ]. Nanomaterials possessing enhanced energy storage properties such as higher energy densities, faster charging capabilities and longer cycle lifetimes hold promise for substantially augmenting EV batteries’ range, efficiency and overall performance. Furthermore, continuous investigations into nanomaterials have the potential to yield sophisticated lightweight materials that integrate composites and nanoscale structures. Such materials would augment EV construction materials’ strength while decreasing their weight. Consequently, this could enhance maneuverability, range and energy efficiency [ 98 , 99 ].
Nanotechnology can create smart, functional surfaces with self-cleaning, anti-fogging and anti-icing qualities that improve EV visibility and efficiency while reducing maintenance. Nanosensors in EVs enable real-time battery health, temperature and performance monitoring, improving charging, safety and maintenance [ 100 ]. As research advances, sustainable nanomaterials that use abundant, non-toxic resources to make the EV sector more environmentally friendly become a priority. As EV nanomaterial recycling reduces resource depletion and waste throughout the lifecycle of EVs, it supports a circular economy. These advances demonstrate the continuous commitment to leverage nanotechnology’s transformative potential in EVs, balancing innovation with environmental responsibility for a sustainable electric mobility future [ 101 , 102 ].
Readers interested in delving more into nanotechnology in batteries will find that internet resources that clarify complicated topics are an excellent place to begin their exploration [ 103 ]. Websites like Nano.gov provide explanations of nanotechnology and its uses that are suitable for beginners. These explanations include the role that nanotechnology plays in battery technology. In addition, educational sites such as Khan Academy offer illuminating video lessons on nanoscience and its applications in the real world, which makes the process of learning more interesting and accessible [ 104 ]. Exploring articles on respected science websites such as Scientific American or National Geographic can provide in-depth yet understandable insights into how nanomaterials contribute to breakthroughs in battery efficiency. This is especially helpful for individuals who prefer a more hands-on approach [ 105 ]. The last point is that books such as Nanotechnology for Dummies by Earl Boysen and Nancy Boysen offer a straightforward guide to comprehending nanotechnology. This makes it an invaluable resource for readers anxious to dispel the mystery surrounding this fascinating field [ 106 ].
This study conducted a systematic literature analysis to analyze bibliometric data from various sources to gain insights into the changing research landscape of nanomaterials in EVs. The study primarily examined author affiliations, nation collaboration maps, citation patterns, keywords and the frequency of author keywords. The research publication selection process is depicted in Figure 2 , highlighting the meticulousness and thoroughness of the literature evaluation. Between 1991 and July 2023, the research yielded 2361 articles about EVs, nanotechnology, nanomaterials and sustainable development. By applying the exclusion criteria, the selection was narrowed down to 1540 articles that were deemed relevant, resulting in a strong dataset for bibliometric analysis. Prominent journals such as Energy and Environmental Science , Nano Energy , ACS Applied Materials and Interfaces and the Journal of Materials Chemistry emphasized the importance of nanoparticles in the context of EVs. The significance of bibliometric analysis in comprehending the impact, influence and interconnections among academic journals was underscored. Citation patterns were employed to assess the scientific value of articles, monitor research trends and evaluate collaboration networks. The study demonstrated the ever-changing nature of how scientific knowledge is spread and highlighted the importance of bibliometric research in guiding politicians, institutions and researchers when making decisions. Figure 3 depicts how the Scopus article research selections were reduced in the first step.
Section 4 included an in-depth examination of nanomaterials in EVs, including a study of publishing trends, country production, thematic evaluation of keywords, collaboration maps and field plots. Figure 4 depicts the yearly dissemination of publications from 1991 to 2023. It shows a notable increase in research efforts beginning in 2005, with an estimated annual growth rate of around 12.79%. The collaboration map depicted in Figure 5 prominently showcased the significant contributions made by prominent universities, with Nanyang Technological University and Beijing University taking the lead in research endeavors. Figure 6 provides an overview of the worldwide contributions to nanotechnology in EVs, with China, India and the United States as the primary countries leading in this field. The analysis of keywords ( Figure 7 ) revealed the consistent presence of terms such as self-powered sensors, batteries, triboelectric nanogenerator, nanotechnology, renewable energy, lithium-ion batteries, electrode material and electrochemical performance. These terms indicate the main themes of the research. Figures 8 and 9 depict the cooperative endeavors of several nations, with particular emphasis on China, India, the United States, South Korea, Germany, Japan, the United Kingdom, the Philippines, Singapore, France, Australia, Canada, Italy, Spain and Malaysia, which are recognized as significant contributors in this sector. Figures 10 and 11 visually depict keyword analysis, revealing the most common study topics and the network of writers who collaborated. A list of the 50 most significant keywords was compiled, which included terms such as ‘nanotechnology,’ ‘lithium,’ ‘electrodes,’ ‘electric batteries,’ ‘nanostructured materials,’ and ‘energy efficiency.’ The treemap structure ( Figure 10c ) displayed the distribution of keywords in publications by different authors, providing a detailed comprehension of the research landscape. To summarize, this SLR thoroughly examines nanomaterials in EVs, utilizing bibliometric methodologies to uncover patterns in research, collaborations and important theme areas. The results emphasize the increasing significance of nanotechnology in the progression of EV technologies. Research gaps may exist in comprehending the precise applications of nanomaterials, tackling issues related to scalability and cost-efficiency, and investigating potential environmental consequences. Potential areas for future research could prioritize interdisciplinary investigations, technological advancements and sustainable methodologies to facilitate the incorporation of nanomaterials into EV technologies. The findings obtained from this work provide valuable contributions to the wider discussion on the convergence of nanotechnology and electric cars, offering guidance for future research efforts in this rapidly changing and developing subject.
In conclusion, the bibliometric analysis conducted on nanotechnology in EVs provides valuable insights into this field’s research landscape and trends. The study highlights the growing interest and research output dedicated to nanotechnology’s applications in electric cars, showcasing its significance and potential impact. By examining publication trends, influential articles, collaborating institutions and key contributors, the analysis helps identify the key areas of research focus and the leading contributors in the field. This information can guide future research directions, collaboration opportunities and resource allocation. The analysis also highlights the challenges and opportunities associated with nanotechnology in EVs. It reveals the need to address scalability, cost-effectiveness, long-term stability and environmental impact to realize the potential of nanotechnology in this domain fully.
Moreover, the bibliometric analysis is a foundation for further exploration and understanding of nanotechnology in EVs. It provides a basis for researchers, policymakers and industry stakeholders to assess the progress made, identify knowledge gaps and develop strategies to accelerate the development and adoption of nanotechnology in EVs. Overall, the bibliometric analysis of nanotechnology in EVs is a valuable tool that enhances our understanding of the research landscape, highlights emerging trends and offers insights into the future perspectives of this exciting field. It paves the way for further research and collaboration, ultimately contributing to advancing and implementing nanotechnology in EVs for a greener and more sustainable transportation future.
The authors declare that there is no conflict of interest in this work.
The authors extend their appreciation to the Deanship of Scientific Research at King Khalid University, Saudi Arabia, for funding this work through the Research Group Program under grant no. RGP 2/88/44.
Pulkit Kumar: Conceptualization, Methodology, Software, Writing – Original Draft, Data Curation, Writing – Review & Editing; Harpreet Kaur Channi: Conceptualization, Methodology, Supervision, Writing – Review & Editing, Formal analysis; Atul Babbar: Investigation, Supervision, Resources, Formal analysis; Raman Kumar: Investigation, Supervision, Conceptualization, Methodology, Formal analysis, Writing – Review & Editing; Javed Khan Bhutto: Formal analysis, Investigation, Supervision T.M. Yunus Khan: Investigation, Visualization; Abhijit Bhowmik: Formal analysis, Investigation, Supervision; Abdul Razak: Formal analysis, Investigation, Supervision; Anteneh Wogasso Wodajo: Formal analysis, Visualization, Supervision
Thomas J , Patil RS , John J . et al. A comprehensive outlook of scope within exterior automotive plastic substrates and its coatings . Coatings (Basel) 2023 ; 13 :1569. https://doi.org/10.3390/coatings13091569 .
Google Scholar
Channi HK , Kumar R . The role of smart sensors in smart city . Smart Sensor Networks: Analytics, Sharing and Control 2022 ; 92 : 27 – 48 . https://doi.org/10.1007/978-3-030-77214-7_2 .
Parameswaran AK . et al. Recent progress of nanotechnology in the research framework of all-solid-state batteries . Nano Energy 2023 ; 105 :107994. https://doi.org/10.1016/j.nanoen.2022.107994 .
Kaur H , Kumar R , Kumar P . et al. 2024 . Efficient and cost-effective renewable energy integration of photovoltaic and hydro in rural India using homer pro: a case study of Chupki, Punjab. In Talpa Sai PHVS, Potnuru S, Avcar M, Ranjan Kar V (eds). Intelligent Manufacturing and Energy Sustainability: Proceedings of ICIMES 2023 . Springer . 281 – 91 .
Google Preview
Saraogi AK , Ibrahim M , Sangeethkumar E . et al. Battery materials for electric vehicle—a comprehensive review . Mater Today: Proc 2023 ; 72 : 2206 – 11 .
Naik N , Suresh P , Yadav S . et al. A review on composite materials for energy harvesting in electric vehicles . Energies 2023 ; 16 : 3348 . https://doi.org/10.3390/en16083348 .
Haghani M , Sprei F , Kazemzadeh K . et al. Trends in electric vehicles research . Transp Res Part D: Transp Environ 2023 ; 123 :103881. https://doi.org/10.1016/j.trd.2023.103881 .
Khan FNU , Rasul MG , Sayem A . et al. Design and optimization of lithium-ion battery as an efficient energy storage device for electric vehicles: a comprehensive review . J Energy Storage 2023 ; 71 :108033. https://doi.org/10.1016/j.est.2023.108033 .
Saha RK , Kumar R , Dev N . et al. Structural modeling and analysis of fuel cell: a graph-theoretic approach . Comput Sci 2023 ; 9 :e1510. https://doi.org/10.7717/peerj-cs.1510 .
Hameed MM , Mansor MB , Azau MAM . et al. Computational design and analysis of LiFePO4 battery thermal management system (BTMS) using thermoelectric cooling/thermoelectric generator (TEC–TEG) in electric vehicles (EVs) . J Energy Storage 2023 ; 72 :108394. https://doi.org/10.1016/j.est.2023.108394 .
Malik S , Muhammad K , Waheed Y . Nanotechnology: a revolution in modern industry . Molecules 2023 ; 28 : 661 . https://doi.org/10.3390/molecules28020661 .
Wali S . et al. Grid-connected lithium-ion battery energy storage system towards sustainable energy: a patent landscape analysis and technology updates . J Energy Storage 2024 ; 77 :109986. https://doi.org/10.1016/j.est.2023.109986 .
Shahzad K , Cheema II . Low-carbon technologies in automotive industry and decarbonizing transport . J Power Sources 2024 ; 591 :233888. https://doi.org/10.1016/j.jpowsour.2023.233888 .
Ahmad A , Prakash O , Sarangi SK . et al. Thermal and CFD analyses of sustainable heat storage-based passive greenhouse dryer operating in no-load condition . Sustain For 2023 ; 15 : 12067 . https://doi.org/10.3390/su151512067 .
Sharma YK , Kumar Y , Sharma S . et al. Nanotechnologies in the renewable energy sector . Renewable Energy Innovations: Biofuels, Solar, and Other Technologies 2024 ; 41 – 82 . https://doi.org/10.1002/9781119785712.ch2 .
Shu X . et al. Sustainability assessment of energy storage technologies based on commercialization viability: MCDM model . Sustain For 2023 ; 15 : 4707 . [Online]. Available: https://www.mdpi.com/2071–1050/15/6/4707 .
Nazir S , Zhang JM , Junaid M . et al. Metal-based nanoparticles: basics, types, fabrications and their electronic applications . Z Phys Chem 2024 ; 0 . https://doi.org/10.1515/zpch-2023-0375 .
Alemu MA , Worku AK , Getie MZ . Recent advances in electrically rechargeable transition metal-based-air batteries for electric mobility . Inorg Chem Commun 2024 ; 159 :111742. https://doi.org/10.1016/j.inoche.2023.111742 .
Cao J , Ji Y , Shao Z . Nanotechnologies in ceramic electrochemical cells . Chem Soc Rev 2024 ; 53 : 450 – 501 . https://doi.org/10.1039/D3CS00303E .
Aryanfar A , Elias F , Goddard WA . Enhancing the thermal dissipation in batteries via inclusion of central heat sink . J Electrochem Energy Convers Storage 2024 ; 21 . https://doi.org/10.1115/1.4062712 .
Mishra S , Mishra AK . 2024 . Supercapacitors: carbon-based nanostructures for supercapacitor application. In Sonkar P, Ganesan V (eds). Nanomaterials for Sustainable Energy Applications . CRC Press . 77 – 100 .
Zhao Y , Geng C , Wang L . et al. Design and modification of metal sulfide-based catalysts for lithium-sulfur batteries . Particuology 2024 ; 86 : 86 – 100 . https://doi.org/10.1016/j.partic.2023.04.010 .
Sanguesa JA , Torres-Sanz V , Garrido P . et al. A review on electric vehicles: technologies and challenges . Smart Cities 2021 ; 4 : 372 – 404 . [Online]. Available: https://doi.org/10.3390/smartcities4010022 . Accessed on (15 November, 2023) . https://www.mdpi.com/2624–6511/4/1/22 .
Ali MU , Zafar A , Nengroo SH . et al. Towards a smarter battery management system for electric vehicle applications: a critical review of lithium-ion battery state of charge estimation . Energies 2019 ; 12 : 446 . https://doi.org/10.3390/en12030446 .
Han D , Hosamo H , Ying C . et al. A comprehensive review and analysis of nanosensors for structural health monitoring in bridge maintenance: innovations, challenges, and future perspectives . Appl Sci 2023 ; 13 : 11149 . [Online]. Available: . https://www.mdpi.com/2076–3417/13/20/11149 . Accessed on (20 November, 2023) .
Basu AK , Tatiya S , Bhattacharya S . Overview of electric vehicles (EVs) and EV sensors . Sensors for Automotive and Aerospace Applications 2019 ; 107 – 22 . https://doi.org/10.1007/978-981-13-3290-6_7 .
Daramy-Williams E , Anable J , Grant-Muller S . A systematic review of the evidence on plug-in electric vehicle user experience . Transp Res Part D: Transp Environ 2019 ; 71 : 22 – 36 . https://doi.org/10.1016/j.trd.2019.01.008 .
Das UK . et al. Advancement of lithium-ion battery cells voltage equalization techniques: a review . Renew Sustain Energy Rev 2020 ; 134 :110227. https://doi.org/10.1016/j.rser.2020.110227 .
Torreglosa JP , Garcia-Triviño P , Vera D . et al. Analyzing the improvements of energy management systems for hybrid electric vehicles using a systematic literature review: how far are these controls from rule-based controls used in commercial vehicles? Appl Sci 2020 ; 10 : 8744 . https://doi.org/10.3390/app10238744 .
Castillo O , Álvarez R , Domingo R . Opportunities and barriers of hydrogen–electric hybrid powertrain vans: a systematic literature review . Processes 2020 ; 8 : 1261 . https://doi.org/10.3390/pr8101261 .
Tete PR , Gupta MM , Joshi SS . Developments in battery thermal management systems for electric vehicles: a technical review . J Energy Storage 2021 ; 35 :102255. https://doi.org/10.1016/j.est.2021.102255 .
Suryatna A , Raya I , Thangavelu L . et al. A review of high-energy density lithium-air battery technology: investigating the effect of oxides and nanocatalysts . J Chem 2022 ; 2022 : 1 – 32 . https://doi.org/10.1155/2022/2762647 .
Ye C , He W , Chen H . Electric vehicle routing models and solution algorithms in logistics distribution: a systematic review . Environ Sci Pollut Res 2022 ; 29 : 57067 – 90 . https://doi.org/10.1007/s11356-022-21559-2 .
Raugei M . Update on the life-cycle GHG emissions of passenger vehicles: literature review and harmonization . Energies 2022 ; 15 : 7163 . https://doi.org/10.3390/en15197163 .
Lai X , Yao J , Jin C . et al. A review of lithium-ion battery failure hazards: test standards, accident analysis, and safety suggestions . Batteries 2022 ; 8 : 248 . https://doi.org/10.3390/batteries8110248 .
Cai S , Kirtley JL , Lee CH . Critical review of direct-drive electrical machine systems for electric and hybrid electric vehicles . IEEE Trans Energy Convers 2022 ; 37 : 2657 – 68 . https://doi.org/10.1109/TEC.2022.3197351 .
Pardhi S , Chakraborty S , Tran D-D . et al. A review of fuel cell powertrains for long-haul heavy-duty vehicles: technology, hydrogen, energy and thermal management solutions . Energies 2022 ; 15 : 9557 . https://doi.org/10.3390/en15249557 .
Sharma P , Bora BJ . A review of modern machine learning techniques in the prediction of remaining useful life of lithium-ion batteries . Batteries 2023 ; 9 : 13 . https://doi.org/10.3390/batteries9010013 .
Senol M , Bayram IS , Naderi Y . et al. Electric vehicles under low temperatures: a review on battery performance, charging needs, and power grid impacts . IEEE Access 2023 ; 11 : 39879 – 912 . https://doi.org/10.1109/ACCESS.2023.3268615 .
Kiran MD , B R LY , Babbar A . et al. Tribological properties of CNT-filled epoxy-carbon fabric composites: optimization and modelling by machine learning . J Mater Res Technol 2024 ; 28 : 2582 – 601 . https://doi.org/10.1016/j.jmrt.2023.12.175 .
He R , Xie W , Wu B . et al. Towards interactional management for power batteries of electric vehicles . RSC Adv 2023 ; 13 : 2036 – 56 . https://doi.org/10.1039/D2RA06004C .
Quilty CD , Wu D , Li W . et al. Electron and ion transport in lithium and lithium-ion battery negative and positive composite electrodes . Chem Rev 2023 ; 123 : 1327 – 63 . https://doi.org/10.1021/acs.chemrev.2c00214 .
Gevorkov L , Domínguez-García JL , Romero LT . et al. Modern MultiPort converter technologies: a systematic review . Appl Sci 2023 ; 13 : 2579 . https://doi.org/10.3390/app13042579 .
Zhang X , Tang Y , Zhang F . et al. A novel aluminum–graphite dual-ion battery . Adv Energy Mater 2016 ; 6 : 1502588 . https://doi.org/10.1002/aenm.201502588 .
Wang M , Jiang C , Zhang S . et al. Reversible calcium alloying enables a practical room-temperature rechargeable calcium-ion battery with a high discharge voltage . Nat Chem 2018 ; 10 : 667 – 72 . https://doi.org/10.1038/s41557-018-0045-4 .
Mu S , Liu Q , Kidkhunthod P . et al. Molecular grafting towards high-fraction active nanodots implanted in N-doped carbon for sodium dual-ion batteries . Natl Sci Rev 2020 ; 8 : nwaa178 . https://doi.org/10.1093/nsr/nwaa178 .
Zhang X , Gong L , Zhao X . et al. Voltage and frequency stabilization control strategy of virtual synchronous generator based on small signal model . Energy Rep 2023 ; 9 : 583 – 90 . https://doi.org/10.1016/j.egyr.2023.03.071 .
Lu L , Wu W , Gao Y . et al. Study on current discrepancy and redistribution of HTS non-insulation closed-loop coils during charging/discharging and subsequent transient process toward steady-state operation . Supercond Sci Technol 2022 ; 35 :095001. https://doi.org/10.1088/1361-6668/ac7dfe .
Wu Y , Wu H , Zhao Y . et al. Metastable structures with composition fluctuation in cuprate superconducting films grown by transient liquid-phase assisted ultra-fast heteroepitaxy . Mater Today Nano 2023 ; 24 :100429. https://doi.org/10.1016/j.mtnano.2023.100429 .
Shen Y , Xie J , He T . et al. CEEMD-fuzzy control energy management of hybrid energy storage systems in electric vehicles . IEEE Trans Energy Convers 2024 ; 39 : 555 – 66 . https://doi.org/10.1109/TEC.2023.3306804 .
Zhao J , Song D , Zhu B . et al. A human-like trajectory planning method on a curve based on the driver preview mechanism . IEEE Trans Intell Transp Syst 2023 ; 24 : 11682 – 98 . https://doi.org/10.1109/TITS.2023.3285430 .
Zhu B , Sun Y , Zhao J . et al. A critical scenario search method for intelligent vehicle testing based on the social cognitive optimization algorithm . IEEE Trans Intell Transp Syst 2023 ; 24 : 7974 – 86 . https://doi.org/10.1109/TITS.2023.3268324 .
Wei T , Zhou Y , Sun C . et al. An intermittent lithium deposition model based on CuMn-bimetallic MOF derivatives for composite lithium anode with ultrahigh areal capacity and current densities . Nano Res 2023 ; 1 – 7 . https://doi.org/10.1007/s12274-023-6187-8 .
Yue W , Li C , Wang S . et al. Cooperative incident management in mixed traffic of CAVs and human-driven vehicles . IEEE Trans Intell Transp Syst 2023 ; 24 : 12462 – 76 . https://doi.org/10.1109/TITS.2023.3289983 .
Lu Y , Tan C , Ge W . et al. Adaptive disturbance observer-based improved super-twisting sliding mode control for electromagnetic direct-drive pump . Smart Mater Struct 2022 ; 32 :017001.
Yu H , Chen D , Ni X . et al. Reversible adsorption with oriented arrangement of a zwitterionic additive stabilizes electrodes for ultralong-life Zn-ion batteries . Energ Environ Sci 2023 ; 16 : 2684 – 95 . https://doi.org/10.1039/D3EE00982C .
Hou X , Xin L , Fu Y . et al. A self-powered biomimetic mouse whisker sensor (BMWS) aiming at terrestrial and space objects perception . Nano Energy 2023 ; 118 :109034. https://doi.org/10.1016/j.nanoen.2023.109034 .
Yan Z , Hu Q , Jiang F . et al. Mechanism and technology evaluation of a novel alternating-arc-based directed energy deposition method through polarity-switching self-adaptive shunt . Addit Manuf 2023 ; 67 :103504. https://doi.org/10.1016/j.addma.2023.103504 .
Wang Z , Li J , Hu C . et al. Hybrid energy storage system and management strategy for motor drive with high torque overload . J Energy Storage 2024 ; 75 :109432. https://doi.org/doi:10.1016/j.est.2023.109432 .
Sidhu AS , Singh S , Kumar R . Bibliometric analysis of entropy weights method for multi-objective optimization in machining operations . Mater Today: Proc 2022 ; 50 : 1248 – 55 . https://doi.org/10.1016/j.matpr.2021.08.132 .
Kumar R , Rani S , Awadh MA . Exploring the application sphere of the internet of things in industry 4.0: a review, bibliometric and content analysis . Sensor 2022 ; 22 : 4276 . https://doi.org/10.3390/s22114276 .
Kumar R , Goel P . Exploring the domain of interpretive structural modelling (ISM) for sustainable future panorama: a bibliometric and content analysis . Arch Comput Methods Eng 2022 ; 29 : 2781 – 810 . https://doi.org/10.1007/s11831-021-09675-7 .
Kaur S , Kumar R , Kaur R . et al. Piezoelectric materials in sensors: bibliometric and visualization analysis . Mater Today: Proc 2022 ; 65 : 3780 – 6 . https://doi.org/10.1016/j.matpr.2022.06.484 .
A. S. Sidhu , S. Singh , and R. Kumar , "Bibliometric analysis of entropy weights method for multi-objective optimization in machining operations," in 2nd International Conference on Functional Materials, Manufacturing and Performances, ICFMMP 2021 , Volume 50, Part 5 , 2021 , Elsevier Ltd , pp. 1248 – 1255 , [Online]. Available: https://www.scopus.com/inward/record.uri?eid=2-s2.0-85124946918&doi=10.1016%2fj.matpr.2021.08.132&partnerID=40&md5=06e988ed2dd8421efbcc139f893d1dcb . Accessed on (20 November, 2023) .
Kumar R , Singh S , Sidhu AS . et al. Bibliometric analysis of specific energy consumption (SEC) in machining operations: a sustainable response . Sustain For 2021 ; 13 :5617. [Online]. Available: https://doi.org/10.3390/su13105617 . https://www.mdpi.com/2071-1050/13/10/5617 .
Rani S , Kumar R . Bibliometric review of actuators: key automation technology in a smart city framework . Mater Today: Proc 2022 ; 60 : 1800 – 7 . https://doi.org/10.1016/j.matpr.2021.12.469 .
Paganin L , Borsato M . A critical review of design for reliability-a bibliometric analysis and identification of research opportunities . Procedia Manuf 2017 ; 11 : 1421 – 8 . https://doi.org/10.1016/j.promfg.2017.07.272 .
Donthu N , Kumar S , Mukherjee D . et al. How to conduct a bibliometric analysis: an overview and guidelines . J Bus Res 2021 ; 133 : 285 – 96 . https://doi.org/doi:10.1016/j.jbusres.2021.04.070 .
Agbodjan YS , Wang J , Cui Y . et al. Bibliometric analysis of zero energy building research, challenges and solutions . Sol Energy 2022 ; 244 : 414 – 33 . https://doi.org/10.1016/j.solener.2022.08.061 .
Xiong H , Wang Y , Guo X . et al. Current status and future challenges of groundwater vulnerability assessment: a bibliometric analysis . J Hydrol 2022 ; 615 :128694. https://doi.org/10.1016/j.jhydrol.2022.128694 .
Akhtar MN , Haleem A , Javaid M . Exploring the advent of Medical 4.0: a bibliometric analysis systematic review and technology adoption insights . Informatics Health 2024 ; 1 : 16 – 28 . https://doi.org/10.1016/j.infoh.2023.10.001 .
Wong K , Dia S . Nanotechnology in batteries . J Energy Resour Technol 2017 ; 139 :014001. https://doi.org/10.1115/1.4034860 .
Yang B , Ji J , Zhang X . et al. Passive cooling of lithium-ion batteries based on flexible phase change materials: molecular structure, interactions and mechanistic aspects . J Mol Liq 2023 ; 391 :123340. https://doi.org/10.1016/j.molliq.2023.123340 .
Kumar M , Farwaha HS , Kumar R . et al. Thermal performance evaluation of solar collector with rice husk graphene-PCM: bioengineering approach . Case Stud Therm Eng 2023 ; 52 :103773. https://doi.org/10.1016/j.csite.2023.103773 .
Chen Y , Fan Z , Liang L . et al. Nanotechnology in modern practical electric vehicles . Highl Sci Eng Technol 2023 ; 46 : 1 – 7 . https://doi.org/10.54097/hset.v46i.7656 .
Mahardhika SP , Putriani O . 2023 . A review of artificial intelligence-enabled electric vehicles in traffic congestion management. In ICSEDTI 2022: Proceedings of the 1st International Conference on Sustainable Engineering Development and Technological Innovation, ICSEDTI 2022, 11–13 October 2022 . Tanjungpinang, Indonesia : European Alliance for Innovation . 255 .
Sun L . Nanotechnology in the field of electric vehicles . Highl Sci Eng Technol 2023 ; 43 : 327 – 32 . https://doi.org/10.54097/hset.v43i.7436 .
Wang Y . Application of advanced nanotechnology in electric vehicles . Highl Sci Eng Technol 2023 ; 46 : 8 – 13 . https://doi.org/10.54097/hset.v46i.7657 .
Shukla S , Khan R , Saxena A . et al. Future of modern society: sustainability in green nanotechnology . Nanoremediation 2023 ; 393 – 410 . https://doi.org/10.1016/B978-0-12-823874-5.00001-2 .
Kurapati SK , Mahendar Reddy N , Sujithra R . et al. Nanomaterials and nanostructures in additive manufacturing: properties, applications, and technological challenges . Nanotechnology-Based Additive Manufacturing: Product Design, Properties and Applications 2023 ; 1 : 53 – 102 . https://doi.org/10.1002/9783527835478.ch3 .
Madheswaran DK , Vengatesan S , Varuvel EG . et al. Nanofluids as a coolant for polymer electrolyte membrane fuel cells: recent trends, challenges, and future perspectives . J Clean Prod 2023 ; 424 :138763. https://doi.org/10.1016/j.jclepro.2023.138763 .
Zero N . Nano-technology to achieve net zero goals . MBMST 2023 2023 ; 392 : 32 .
Bosu S , Rajamohan N . Recent advancements in hydrogen storage-comparative review on methods, operating conditions and challenges . Int J Hydrogen Energy 2024 ; 52 : 352 – 70 . https://doi.org/10.1016/j.ijhydene.2023.01.344 .
Shilkov VI , Anikin YV , Grishchenko YO . 2023 . Promising innovations for green economy and cleaner production in agriculture. In Trukhachev VI (ed). Unlocking Digital Transformation of Agricultural Enterprises: Technology Advances, Digital Ecosystems, and Innovative Firm Governance . Springer . 177 – 87 .
Soukupová G , Jindra M , Lapka T . et al. Novel silicon nanoparticles-based carbonized polypyrrole nanotube composites as anode materials for Li-ion batteries . J Power Sources 2024 ; 593 :233976. https://doi.org/10.1016/j.jpowsour.2023.233976 .
Poorshakoor E , Darab M . Advancements in the development of nanomaterials for lithium-ion batteries: a scientometric review . J Energy Storage 2024 ; 75 :109638. https://doi.org/10.1016/j.est.2023.109638 .
Hussain W , Sulaman M , Sandali Y . et al. An extensive exploration of non-conventional sources for preparing NiS and its potential applications in battery and photodegradation processes . Mater Sci Eng B 2024 ; 299 :116918. https://doi.org/10.1016/j.mseb.2023.116918 .
Gao S-L , Qin Z-X , Wang B-F . et al. Lithium recovery from the spent lithium-ion batteries by commercial acid-resistant nanofiltration membranes: a comparative study . Desalination 2024 ; 572 :117142. https://doi.org/10.1016/j.desal.2023.117142 .
Thomas F , Mahdi L , Lemaire J . et al. Technological advances and market developments of solid-state batteries: a review . Materials 2024 ; 17 : 239 . https://doi.org/10.3390/ma17010239 .
Schiavi PG , Marrani AG , Russina O . et al. Aqueous electrochemical delithiation of cathode materials as a strategy to selectively recover lithium from waste lithium-ion batteries . J Energy Chem 2024 ; 88 : 144 – 53 . https://doi.org/10.1016/j.jechem.2023.09.040 .
Kharabati S , Saedodin S . A systematic review of thermal management techniques for electric vehicle batteries . J Energy Storage 2024 ; 75 :109586. https://doi.org/10.1016/j.est.2023.109586 .
Divya N , Saju M , Roshna A . et al. 2024 . Smart nanomaterials in energy applications. In Tailored Functional Materials for Clean and Sustainable Development . Apple Academic Press . 263 – 80 .
Karjalainen P , Leinonen V , Olin M . et al. Real-world emissions of nanoparticles, particulate mass and black carbon from a plug-in hybrid vehicle compared to conventional gasoline vehicles . Environ Adv 2024 ; 15 :100454. https://doi.org/10.1016/j.envadv.2023.100454 .
Munonde TS , Raphulu MC . Review on titanium dioxide nanostructured electrode materials for high-performance lithium batteries . J Energy Storage 2024 ; 78 :110064. https://doi.org/10.1016/j.est.2023.110064 .
Lim JM , Jang YS , van T. Nguyen H . et al. Advances in high-voltage supercapacitors for energy storage systems: materials and electrolyte tailoring to implementation . Nanoscale Adv 2023 ; 5 : 615 – 26 . https://doi.org/10.1039/D2NA00863G .
Shi R , Shen Z , Yue Q . et al. Advances in functional organic material-based interfacial engineering on metal anodes for rechargeable secondary batteries . Nanoscale 2023 ; 15 : 9256 – 89 . https://doi.org/10.1039/D3NR01306E .
Edoziuno FO , Adediran AA , Nwaeju CC . et al. 2023 . Nanomaterials. In Verma C, Srivastava V, ... Ebenso EE (eds). Smart Anticorrosive Materials . Elsevier . 19 – 30 . https://doi.org/10.1016/B978-0-323-95158-6.00023-0 .
Huang Z , Li Y , Xu Y . Diverse nanotechnology applications for electric vehicles . Highl Sci Eng Technol 2023 ; 43 : 438 – 49 . https://doi.org/10.54097/hset.v43i.7462 .
Aravindan M . et al. Fuelling the future: a review of non-renewable hydrogen production and storage techniques . Renew Sustain Energy Rev 2023 ; 188 :113791.
Raj KS , Baskaran N , Nair PP . et al. Current scenario and future perspective of food waste into Li-ion based batteries—a critical review . J Hazard Mater Adv 2023 ; 10 :100317. https://doi.org/10.1016/j.hazadv.2023.100317 .
Yang Z . Nanomaterials as well their applications and effects in batteries . Highl Sci Eng Technol 2023 ; 32 : 73 – 82 . https://doi.org/10.54097/hset.v32i.4944 .
Mahdavi Dehkharghani F , Ghahremanlou M , Zandi Z . et al. Future energy and therapeutic perspectives of green nano-technology: recent advances and challenges . Nano Micro Biosyst 2023 ; 2 : 11 – 21 .
Shah SSA , Zafar HK , Javed MS . et al. Mxenes for Zn-based energy storage devices: nano-engineering and machine learning . Coord Chem Rev 2024 ; 501 :215565. https://doi.org/10.1016/j.ccr.2023.215565 .
Li Z , Zhang Y , Zhang S . et al. Phase change materials for lithium-ion battery thermal management systems: a review . J Energy Storage 2024 ; 80 :110259. https://doi.org/10.1016/j.est.2023.110259 .
Karthikeyan B , Velvizhi G . A state-of-the-art on the application of nanotechnology for enhanced biohydrogen production . Int J Hydrogen Energy 2024 ; 52 : 536 – 54 . https://doi.org/10.1016/j.ijhydene.2023.04.237 .
Xie H , Ahmad T , Zhang D . et al. Community-based virtual power plants’ technology and circular economy models in the energy sector: a techno-economy study . Renew Sustain Energy Rev 2024 ; 192 :114189. https://doi.org/10.1016/j.rser.2023.114189 .
Month: | Total Views: |
---|---|
March 2024 | 98 |
April 2024 | 426 |
May 2024 | 464 |
June 2024 | 283 |
July 2024 | 276 |
August 2024 | 52 |
Email alerts
Citing articles via, affiliations.
- Online ISSN 1748-1325
- Print ISSN 1748-1317
- Copyright © 2024 Oxford University Press
- About Oxford Academic
- Publish journals with us
- University press partners
- What we publish
- New features
- Open access
- Institutional account management
- Rights and permissions
- Get help with access
- Accessibility
- Advertising
- Media enquiries
- Oxford University Press
- Oxford Languages
- University of Oxford
Oxford University Press is a department of the University of Oxford. It furthers the University's objective of excellence in research, scholarship, and education by publishing worldwide
- Copyright © 2024 Oxford University Press
- Cookie settings
- Cookie policy
- Privacy policy
- Legal notice
This Feature Is Available To Subscribers Only
Sign In or Create an Account
This PDF is available to Subscribers Only
For full access to this pdf, sign in to an existing account, or purchase an annual subscription.
Recent advances in micro- and nano-machining technologies
- Review Article
- Open access
- Published: 31 March 2017
- Volume 12 , pages 18–32, ( 2017 )
Cite this article
You have full access to this open access article
- Shang Gao 1 , 2 &
- Han Huang 1
4197 Accesses
76 Citations
Explore all metrics
Device miniaturization is an emerging advanced technology in the 21st century. The miniaturization of devices in different fields requires production of micro- and nano-scale components. The features of these components range from the sub-micron to a few hundred microns with high tolerance to many engineering materials. These fields mainly include optics, electronics, medicine, bio-technology, communications, and avionics. This paper reviewed the recent advances in micro- and nano-machining technologies, including micro-cutting, micro-electrical-discharge machining, laser micro-machining, and focused ion beam machining. The four machining technologies were also compared in terms of machining efficiency, workpiece materials being machined, minimum feature size, maximum aspect ratio, and surface finish.
Article PDF
Download to read the full article text
Similar content being viewed by others
Micro and Nano Machining—An Industrial Perspective
Mechanical Micro-machining
Avoid common mistakes on your manuscript.
Luo X, Cheng K, Webb D, et al. Design of ultraprecision machine tools with applications to manufacture of miniature and micro components. Journal of Materials Processing Technology, 2005, 167(2–3): 515–528
Article Google Scholar
Qin Y. Micromanufacturing Engineering and Technology. Oxford: William Andrew, 2010
Google Scholar
Alting L, Kimura F, Hansen H N, et al. Micro engineering. CIRP Annals—Manufacturing Technology, 2003, 52(2): 635–657
Crichton M L, Archer-Jones C, Meliga S, et al. Characterising the material properties at the interface between skin and a skin vaccination microprojection device. Acta Biomaterialia, 2016, 36: 186–194
Vaezi M, Seitz H, Yang S. A review on 3D micro-additive manufacturing technologies. International Journal of Advanced Manufacturing Technology, 2013, 67(5–8): 1721–1754
Liu X, Devor R E, Kapoor S G, et al. The mechanics of machining at the microscale: Assessment of the current state of the science. Journal of Manufacturing Science and Engineering, 2004, 126(4): 666–678
Spearing S M. Materials issues in microelectromechanical systems (MEMS). Acta Materialia, 2000, 48(1): 179–196
Rajurkar K P, Levy G, Malshe A, et al. Micro and nano machining by electro-physical and chemical processes. CIRP Annals—Manufacturing Technology, 2006, 55(2): 643–666
Liu K, Lauwers B, Reynaerts D. Process capabilities of micro-EDM and its applications. International Journal of Advanced Manufacturing Technology, 2010, 47(1–4): 11–19
Masuzawa T. State of the art of micromachining. CIRP Annals— Manufacturing Technology, 2000, 49(2): 473–488
Madou M J. Manufacturing Techniques for Microfabrication and Nanotechnology. Boca Raton: CRC Press, 2011
Book Google Scholar
Brinksmeier E, Riemer O, Stern R. Machining of precision parts and microstructures. In: Inasaki I, ed. Initiatives of Precision Engineering at the Beginning of a Millennium. Berlin: Springer, 2002, 3–11
Chapter Google Scholar
Brousseau E B, Dimov S S, Pham D T. Some recent advances in multi-material micro-and nano-manufacturing. International Journal of Advanced Manufacturing Technology, 2010, 47(1–4): 161–180
Qin Y, Brockett A, Ma Y, et al. Micro-manufacturing: Research, technology outcomes and development issues. International Journal of Advanced Manufacturing Technology, 2010, 47(9–12): 821–837
Dimov S S, Matthews C W, Glanfield A, et al. A roadmapping study in multi-material micro manufacture. In: Proceedings of the Second International Conference on Multi-Material Micro Manufacture. Oxford: Elsevier, 2006, xi–xxv
Chae J, Park S S, Freiheit T. Investigation of micro-cutting operations. International Journal of Machine Tools & Manufacture, 2006, 46(3–4): 313–332
Sumitomo T, Huang H, Zhou L, et al. Nanogrinding of multilayered thin film amorphous Si solar panels. International Journal of Machine Tools & Manufacture, 2011, 51(10–11): 797–805
Yin L, Huang H. Brittle materials in nano-abrasive fabrication of optical mirror-surfaces. Precision Engineering, 2008, 32(4): 336–341
Huo D. Micro-Cutting: Fundamentals and Applications. London: John Wiley & Sons, 2013
Lu Z, Yoneyama T. Micro cutting in the micro lathe turning system. International Journal of Machine Tools & Manufacture, 1999, 39(7): 1171–1183
Rahman M, Asad A, Masaki T, et al. A multiprocess machine tool for compound micromachining. International Journal of Machine Tools & Manufacture, 2010, 50(4): 344–356
Weule H, Hüntrup V, Tritschler H. Micro-cutting of steel to meet new requirements in miniaturization. CIRP Annals—Manufacturing Technology, 2001, 50(1): 61–64
Câmara M A, Rubio J C, Abrão A M, et al. State of the art on micromilling of materials, a review. Journal of Materials Science and Technology, 2012, 28(8): 673–685
Egashira K, Mizutani K. Micro-drilling of monocrystalline silicon using a cutting tool. Precision Engineering, 2002, 26(3): 263–268
Dornfeld D, Min S, Takeuchi Y. Recent advances in mechanical micromachining. CIRP Annals—Manufacturing Technology, 2006, 55(2): 745–768
Aurich J C, Engmann J, Schueler G M, et al. Micro grinding tool for manufacture of complex structures in brittle materials. CIRP Annals—Manufacturing Technology, 2009, 58(1): 311–314
Hoffmeister H, Wenda A. Novel grinding tools for machining precision micro parts of hard and brittle materials. In: Proceedings of the 15th Annual Meeting of the ASPE. 2000, 152–155
Park H, Onikura H, Ohnishi O, et al. Development of microdiamond tools through electroless composite plating and investigation into micro-machining characteristics. Precision Engineering, 2010, 34(3): 376–386
Chen W K, Kuriyagawa T, Huang H, et al. Machining of micro aspherical mould inserts. Precision Engineering, 2005, 29(3): 315–323
Kim J, Kim D S. Theoretical analysis of micro-cutting characteristics in ultra-precision machining. Journal of Materials Processing Technology, 1995, 49(3–4): 387–398
Shaw M C. Precision finishing. CIRP Annals—Manufacturing Technology, 1995, 44(1): 343–348
Liu X, Jun M B, Devor R E, et al. Cutting mechanisms and their influence on dynamic forces, vibrations and stability in microendmilling. In: Proceeding of the ASME 2004 International Mechanical Engineering Congress and Exposition. American Society of Mechanical Engineers, 2004, 583–592
Bissacco G, Hansen H N, De Chiffre L. Size effects on surface generation in micro milling of hardened tool steel. CIRP Annals— Manufacturing Technology, 2006, 55(1): 593–596
Kim C, Mayor J R, Ni J. A static model of chip formation in microscale milling. Journal of Manufacturing Science and Engineering, 2004, 126(4): 710–718
Liu X, Devor R E, Kapoor S G. An analytical model for the prediction of minimum chip thickness in micromachining. Journal of Manufacturing Science and Engineering, 2006, 128(2): 474–481
Vogler MP, Devor R E, Kapoor S G. On the modeling and analysis of machining performance in micro-endmilling, Part I: Surface generation. Journal of Manufacturing Science and Engineering, 2004, 126(4): 685–694
Vogler MP, Kapoor S G, Devor R E. On the modeling and analysis of machining performance in micro-endmilling, Part II: Cutting force prediction. Journal of Manufacturing Science and Engineering, 2004, 126(4): 695–705
Son S M, Lim H S, Ahn J H. Effects of the friction coefficient on the minimum cutting thickness in micro cutting. International Journal of Machine Tools & Manufacture, 2005, 45(4–5): 529–535
Dow T A, Miller E L, Garrard K. Tool force and deflection compensation for small milling tools. Precision Engineering, 2004, 28(1): 31–45
Bao WY, Tansel I N. Modeling micro-end-milling operations. Part I: Analytical cutting force model. International Journal of Machine Tools & Manufacture, 2000, 40(15): 2155–2173
Bao WY, Tansel I N. Modeling micro-end-milling operations. Part II: Tool run-out. International Journal of Machine Tools & Manufacture, 2000, 40(15): 2175–2192
Duan X, Peng F, Yan R, et al. Estimation of cutter deflection based on study of cutting force and static flexibility. Journal of Manufacturing Science and Engineering, 2016, 138(4): 041001
Ma W, He G, Zhu L, et al. Tool deflection error compensation in five-axis ball-end milling of sculptured surface. International Journal of Advanced Manufacturing Technology, 2016, 84(5): 1421–1430
Shabouk S, Nakamoto T. Micro machining of single crystal diamond by utilization of tool wear during cutting process of ferrous material. Journal of Micromechatronics, 2002, 2(1): 13–26
Kalpakjian S, Schmid S R. Manufacturing Processes for Engineering Materials. Upper Saddle River: Prentice Hall, 2007
Schaller T, Bohn L, Mayer J, et al. Microstructure grooves with a width of less than 50 mm cut with ground hard metal micro end mills. Precision Engineering, 1999, 23(4): 229–235
Onikura H, Ohnishi O, Take Y, et al. Fabrication of micro carbide tools by ultrasonic vibration grinding. CIRP Annals—Manufacturing Technology, 2000, 49(1): 257–260
Adams D P, Vasile M J, Benavides G, et al. Micromilling of metal alloys with focused ion beam—Fabricated tools. Precision Engineering, 2001, 25(2): 107–113
Egashira K, Hosono S, Takemoto S, et al. Fabrication and cutting performance of cemented tungsten carbide micro-cutting tools. Precision Engineering, 2011, 35(4): 547–553
Wong Y S, Rahman M, Lim H S, et al. Investigation of micro-EDM material removal characteristics using single RC-pulse discharges. Journal of Materials Processing Technology, 2003, 140(1–3): 303–307
Pham D T, Dimov S S, Bigot S, et al. Micro-EDM—Recent developments and research issues. Journal of Materials Processing Technology, 2004, 149(1): 50–57
Liao Y S, Chen S T, Lin C S, et al. Fabrication of high aspect ratio microstructure arrays by micro reverse wire-EDM. Journal of Micromechanics and Microengineering, 2005, 15(8): 1547–1555
Wang J, Han F. Simulation model of debris and bubble movement in consecutive-pulse discharge of electrical discharge machining. International Journal of Machine Tools & Manufacture, 2014, 77: 56–65
Kadirvel A, Hariharan P, Gowri S. A review on various research trends in micro-EDM. International Journal of Mechatronics and Manufacturing Systems, 2012, 5(5/6): 361–384
Pham D T, Ivanov A, Bigot S, et al. An investigation of tube and rod electrode wear in micro EDM drilling. International Journal of Advanced Manufacturing Technology, 2007, 33(1–2): 103–109
Pham D T, Ivanov A, Bigot S, et al. A study of micro-electro discharge machining electrode wear. Proceedings of the Institution of Mechanical Engineers. Part C, Journal of Mechanical Engineering Science, 2007, 221(5): 605–612
Dimov S, Pham D T, Ivanov A, et al. Tool-path generation system for micro-electro discharge machining milling. Proceedings of the Institution of Mechanical Engineers. Part B, Journal of Engineering Manufacture, 2003, 217(11): 1633–1637
Tsai Y, Masuzawa T. An index to evaluate the wear resistance of the electrode in micro-EDM. Journal of Materials Processing Technology, 2004, 149(1–3): 304–309
Uhlmann E, Roehner M. Investigations on reduction of tool electrode wear in micro-EDM using novel electrode materials. CIRP Journal of Manufacturing Science and Technology, 2008, 1 (2): 92–96
Aligiri E, Yeo S H, Tan P C. A new tool wear compensation method based on real-time estimation of material removal volume in micro-EDM. Journal of Materials Processing Technology, 2010, 210(15): 2292–2303
Bissacco G, Hansen H N, Tristo G, et al. Feasibility of wear compensation in micro EDM milling based on discharge counting and discharge population characterization. CIRP Annals—Manufacturing Technology, 2011, 60(1): 231–234
Masuzawa T, Fujino M, Kobayashi K, et al. Wire electro-discharge grinding for micro-machining. CIRP Annals—Manufacturing Technology, 1985, 34(1): 431–434
Rees A, Dimov S S, Ivanov A, et al. Micro-electrode discharge machining: Factors affecting the quality of electrodes produced on the machine through the process of wire electro-discharge machining. Proceedings of the Institution of Mechanical Engineers. Part B, Journal of Engineering Manufacture, 2007, 221(3): 409–418
Uhlmann E, Piltz S, Jerzembeck S. Micro-machining of cylindrical parts by electrical discharge grinding. Journal of Materials Processing Technology, 2005, 160(1): 15–23
Qu J, Shih A J, Scattergood R O. Development of the cylindrical wire electrical discharge machining process, Part 1: Concept, design, and material removal rate. Journal of Manufacturing Science and Engineering, 2002, 124(3): 702–707
Qu J, Shih A J, Scattergood R O. Development of the cylindrical wire electrical discharge machining process, Part 2: Surface integrity and roundness. Journal of Manufacturing Science and Engineering, 2002, 124(3): 708–714
Rees A, Brousseau E, Dimov S S, et al. Wire electro discharge grinding: surface finish optimisation. Multi-Material Micro Manufacture, 2008, 1–4
Meijer J. Laser beam machining (LBM), state of the art and new opportunities. Journal of Materials Processing Technology, 2004, 149(1–3): 2–17
Knowles M R H, Rutterford G, Karnakis D, et al. Micro-machining of metals, ceramics and polymers using nanosecond lasers. International Journal of Advanced Manufacturing Technology, 2007, 33(1–2): 95–102
Rizvi N H, Apte P. Developments in laser micro-machining techniques. Journal of Materials Processing Technology, 2002, 127 (2): 206–210
Pham D T, Dimov S S, Ji C, et al. Laser milling as a ‘rapid’ micromanufacturing process. Proceedings of the Institution of Mechanical Engineers. Part B, Journal of Engineering Manufacture, 2004, 218(1): 1–7
Corcoran A, Sexton L, Seaman B, et al. The laser drilling of multilayer aerospace material systems. Journal of Materials Processing Technology, 2002, 123(1): 100–106
Matsuoka Y, Kizuka Y, Inoue T. The characteristics of laser micro drilling using a Bessel beam. Applied Physics. A, Materials Science & Processing, 2006, 84(4): 423–430
Biswas R, Kuar A S, Sarkar S, et al. A parametric study of pulsed Nd: YAG laser micro-drilling of gamma-titanium aluminide. Optics & Laser Technology, 2010, 42(1): 23–31
Zheng H Y, Huang H. Ultrasonic vibration-assisted femtosecond laser machining of microholes. Journal of Micromechanics and Microengineering, 2007, 17(8): N58–N61
Mao C, Sun X, Huang H, et al. Characteristics and removal mechanism in laser cutting of cBN-WC-10Co composites. Journal of Materials Processing Technology, 2016, 230: 42–49
Petkov P V, Dimov S S, Minev R M, et al. Laser milling: Pulse duration effects on surface integrity. Proceedings of the Institution of Mechanical Engineers. Part B, Journal of Engineering Manufacture, 2008, 222(1): 35–45
Preuss S, Demchuk A, Stuke M. Sub-picosecond UV laser ablation of metals. Applied Physics. A, Materials Science & Processing, 1995, 61(1): 33–37
von der Linde D, Sokolowski-Tinten K. The physical mechanisms of short-pulse laser ablation. Applied Surface Science, 2000, 154–155: 1–10
Kautek W, Krueger J. Femtosecond pulse laser ablation of metallic, semiconducting, ceramic, and biological materials. Laser Materials Processing: Industrial and Microelectronics Applications, 1994, 600–611
Huang H, Zheng H Y, Lim G C. Femtosecond laser machining characteristics of Nitinol. Applied Surface Science, 2004, 228(1–4): 201–206
Dobrev T, Dimov S S, Thomas A J. Laser milling: Modelling crater and surface formation. Proceedings of the Institution of Mechanical Engineers. Part C, Journal of Mechanical Engineering Science, 2006, 220(11): 1685–1696
Pham D T, Dimov S S, Petkov P V. Laser milling of ceramic components. International Journal of Machine Tools & Manufacture, 2007, 47(3–4): 618–626
Kim C S, Ahn S H, Jang D Y. Review: Developments in micro/nanoscale fabrication by focused ion beams. Vacuum, 2012, 86(8): 1014–1035
Tseng A A. Recent developments in micromilling using focused ion beam technology. Journal of Micromechanics and Microengineering, 2004, 14(4): R15–R34
Tseng A A. Recent developments in nanofabrication using focused ion beams. Small, 2005, 1(10): 924–939
Sugiyama M, Sigesato G. A review of focused ion beam technology and its applications in transmission electron microscopy. Journal of Electron Microscopy, 2004, 53(5): 527–536
Ahn S H, Chun D M, Kim C S. Nanoscale hybrid manufacturing process by nano particle deposition system (NPDS) and focused ion beam (FIB). CIRP Annals—Manufacturing Technology, 2011, 60(1): 583–586
Ding X, Lim G C, Cheng C K, et al. Fabrication of a micro-size diamond tool using a focused ion beam. Journal of Micromechanics and Microengineering, 2008, 18(7): 075017
Picard Y N, Adams D P, Vasile M J, et al. Focused ion beamshaped microtools for ultra-precision machining of cylindrical components. Precision Engineering, 2003, 27(1): 59–69
Shim S, Bei H, Miller M K, et al. Effects of focused ion beam milling on the compressive behavior of directionally solidified micropillars and the nanoindentation response of an electropolished surface. Acta Materialia, 2009, 57(2): 503–510
Xu Z, Fang F, Zhang S, et al. Fabrication of micro DOE using micro tools shaped with focused ion beam. Optics Express, 2010, 18(8): 8025–8032
Wu W, Xu Z, Fang F, et al. Decrease of FIB-induced lateral damage for diamond tool used in nano cutting. Nuclear Instruments & Methods in Physics Research. Section B, Beam Interactions with Materials and Atoms, 2014, 330: 91–98
Li W, Minev R, Dimov S, et al. Patterning of amorphous and polycrystalline Ni 78 B 14 Si 8 with a focused-ion-beam. Applied Surface Science, 2007, 253(12): 5404–5410
Li W, Lalev G, Dimov S, et al. A study of fused silica micro/nano patterning by focused-ion-beam. Applied Surface Science, 2007, 253(7): 3608–3614
Wu S E, Liu C P. Direct writing of Si island arrays by focused ion beam milling. Nanotechnology, 2005, 16(11): 2507–2511
Chang T C, Hong K B, Lai Y Y, et al. ZnO-based microcavities sculpted by focus ion beam milling. Nanoscale Research Letters, 2016, 11(1): 319–325
Lu M, Russell H, Huang H. Fracture strength characterization of protective intermetallic coatings on AZ91E Mg alloys using FIBmachined microcantilever bending technique. Journal of Materials Research, 2015, 30(10): 1678–1685
Download references
Acknowledgements
SG was sponsored by the Chinese Scholarship Council (CSC) under postdoctoral fellow program. HH would like to acknowledge the financial sponsorship from Australia Research Council (ARC) under Future Fellowship program.
Author information
Authors and affiliations.
School of Mechanical and Mining Engineering, The University of Queensland, QLD, 4072, Australia
Shang Gao & Han Huang
Key Laboratory for Precision and Non-traditional Machining Technology of Ministry of Education, Dalian University of Technology, Dalian, 116024, China
You can also search for this author in PubMed Google Scholar
Corresponding author
Correspondence to Han Huang .
Additional information
This article is published with open access at link.springer.com and journal.hep.com.cn
Rights and permissions
Open Access This article is distributed under the terms of the Creative Commons Attribution 4.0 International License (http://creativecommons.org/licenses/by/4.0/), which permits unrestricted use, distribution, and reproduction in any medium, provided you give appropriate credit to the original author(s) and the source, provide a link to the Creative Commons license, and indicate if changes were made.
Reprints and permissions
About this article
Gao, S., Huang, H. Recent advances in micro- and nano-machining technologies. Front. Mech. Eng. 12 , 18–32 (2017). https://doi.org/10.1007/s11465-017-0410-9
Download citation
Received : 02 October 2016
Accepted : 16 October 2016
Published : 31 March 2017
Issue Date : March 2017
DOI : https://doi.org/10.1007/s11465-017-0410-9
Share this article
Anyone you share the following link with will be able to read this content:
Sorry, a shareable link is not currently available for this article.
Provided by the Springer Nature SharedIt content-sharing initiative
- micro machining
- electro discharge machining (EDM)
- laser machining
- focused ion beam (FIB)
Advertisement
- Find a journal
- Publish with us
- Track your research
Micro & Nanotechnology - Mechanical Engineering - Purdue University

Micro and Nanotechnology
There’s a big future in small things. Nanotechnology is the new frontier of engineering, imagining new possibilities in manufacturing, fluid mechanics, robotics, combustion, biomedicine, measurements, heat transfer, and more. Purdue hosts the largest academic cleanroom in the world, the Birck Nanotechnology Center , where interdisciplinary teams have access to the absolute cutting-edge of nano-scale characterization (microscopy and measurements) and fabrication (deposition, etching, lithography, etc.) With these tools, mechanical engineers conduct world-class research in:
- Nanoscale manufacturing
- Micro- and nano-fluidics
- Biomolecular detection
- Nanoscale thermal transport
- Computational modeling
- Nanomechanical materials
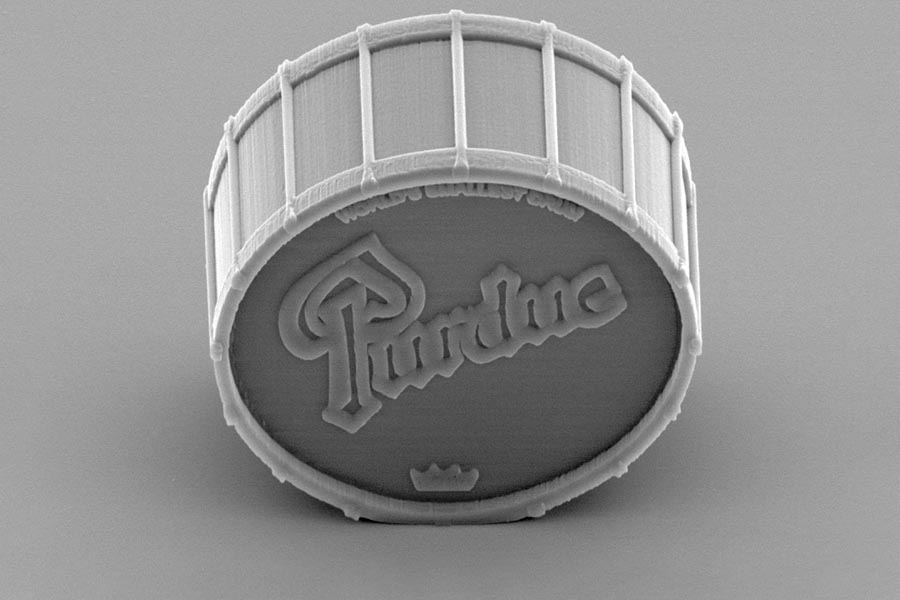
The world's smallest drum
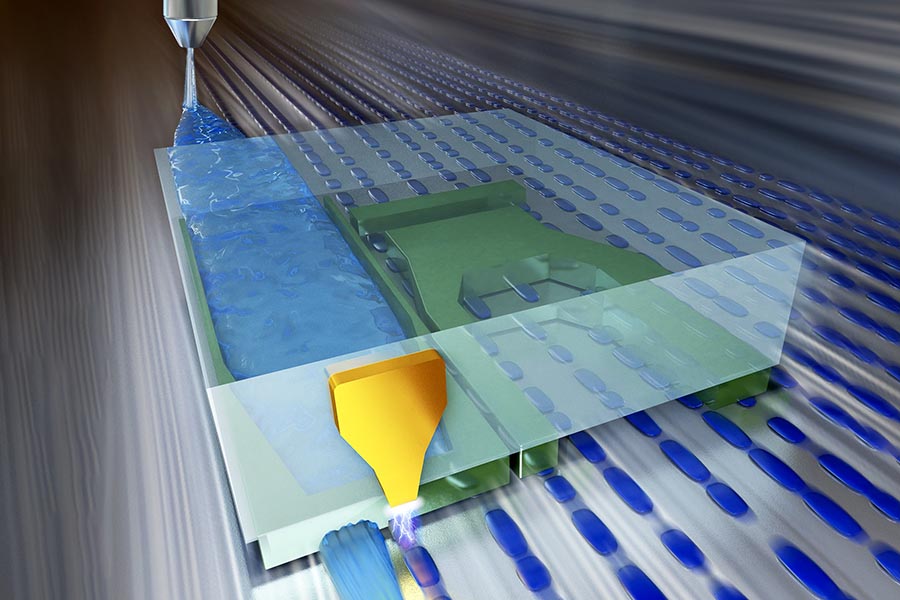
Fastest ever nano-pen "surfs" on a liquid cushion
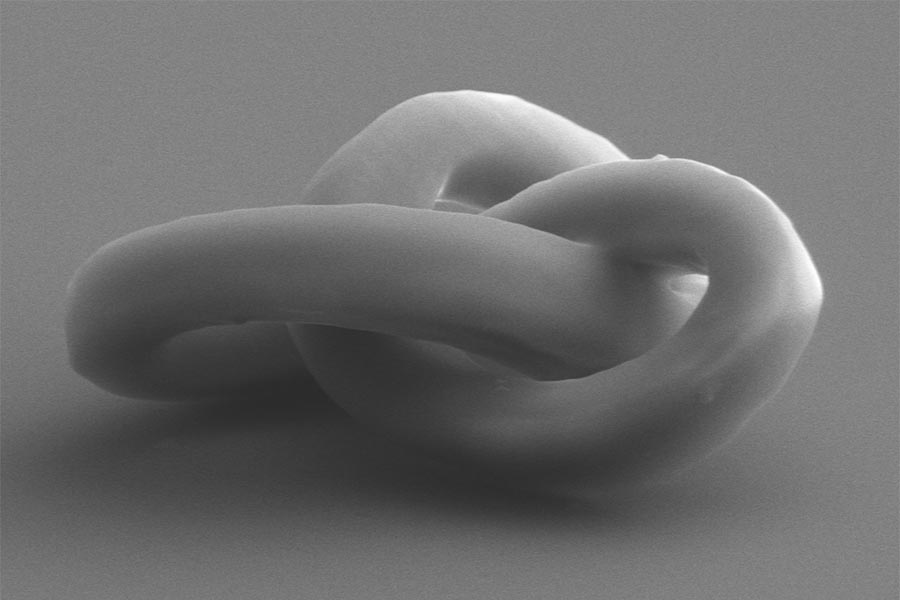
From nano to macro: Nanoscale 3D printing that is fast, smooth, and scalable
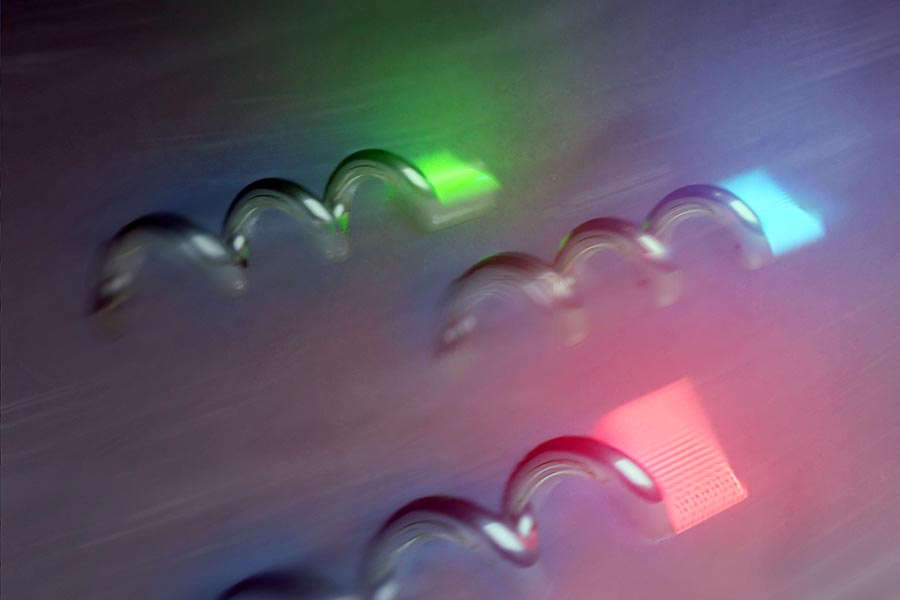
Microrobots show their true colors
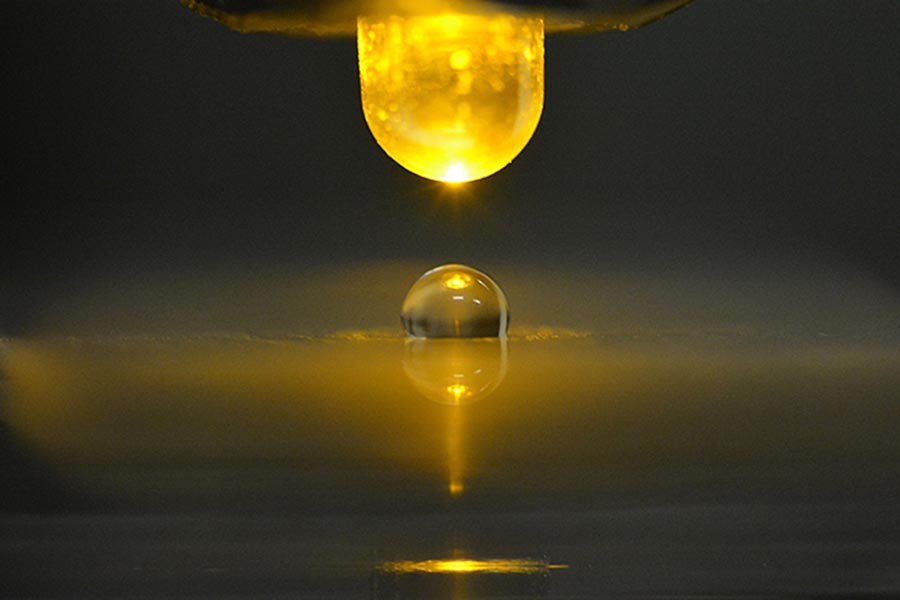
Color-measuring at microscale

Designing cells that follow the leader
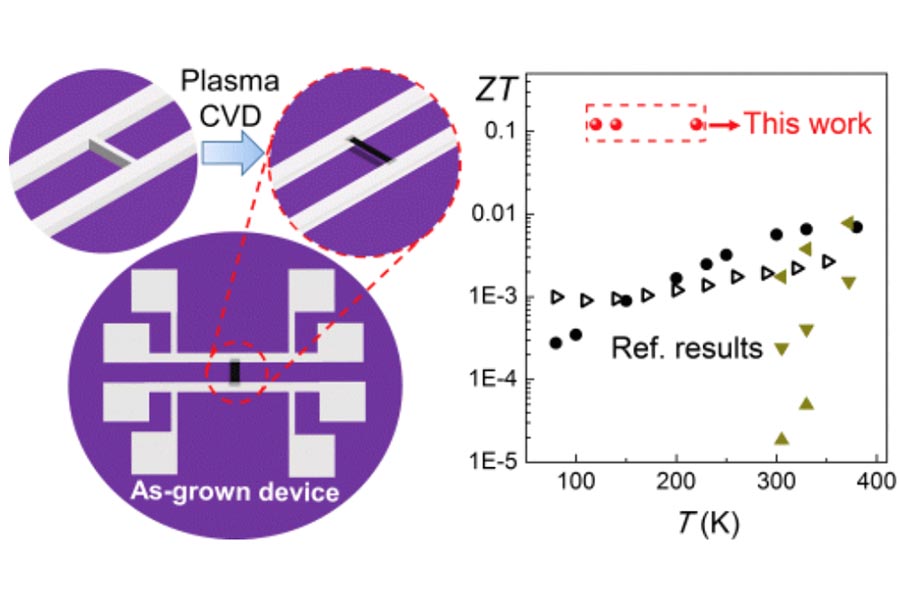
Thermoelectric performance of graphene gets a boost

Nanoscale light manipulation

Nanoneedles deliver medicine with no pain
Faculty in Micro & Nanotechnology
Arezoo ardekani.
- Fluid dynamics
- Biomaterial
- Multiphase flows
- Non-Newtonian fluid dynamics
- Microfluidics
- Complex fluids
- Soft matter
- Modeling of nonlinear systems
- Structural dynamics and localization
- Flow-induced vibrations
- Impacting systems
- Bifurcations and chaos
Shubhra Bansal
- Renewable Energy Materials (physics-based energy yield predictions, sustainable PV and energy storage materials, recycling)
- Electro-Optical-Thermo-Mechanical Reliability (in-situ and in-operando accelerated stress tests)
- Heterogeneous Integration & Advanced Packaging (sub-10 μm pitch interconnects, low-loss interposers)
- Harsh Environment Electronics Integration (high temperature Pb-free solders and nano-thermal interfaces)
Thomas Beechem
- Thermal Physics ... Heat Moving Energy
- Spectroscopy ... "Seeing" energy with light
- Nanophotonics ... Pushing light to see more
- 2D Materials ... Creating functionality by losing dimension
J. Stuart Bolton
- Active and passive noise control
- Sound field visualization
- Structural acoustics and wave propagation in structures
- Noise control material modeling
- Applied signal processing
Mukerrem Cakmak
- Modeling and experimental studies on processing
- Structure property relationships in polymer films and moldings and polymer/metal/ceramic hybrid systems
David Cappelleri
- Multi-scale robotic manipulation and assembly
- Mobile micro/nano robotics
- Micro/nano aerial vehicles
- Micro-Bio robotics
- Mechatronics
- Automation for the life sciences
Chun-Li Chang
George Chiu
- Dynamic systems and control
- Digital and functional printing and fabrication
- Motion and vibration control and perception
- Embedded systems and real-time control
Jong Hyun Choi
- DNA nanotechnology
- Advanced materials
Alex Chortos
- Bio-inspired and mechanically adaptive electronics
- Multimaterial additive fabrication
- Soft actuators (artificial muscles)
- Wearable actuators (haptics)
- Polymer design and polymer physics
- Deformation sensors and transistors
Ivan Christov
- Fluid Mechanics
- Soft Matter
- Granular Flow
- Nonlinear Waves
- Computational Science
Hamid Dalir
- Composites materials design and manufacturing
- Sustainable and recyclable-by-design polymers and composites
- Polymer processing and characterization
- Composites recycling
- Hybrid manufacturing systems
- Multiscale modeling
- Damage mechanics
Christopher Goldenstein
- Laser-absorption spectroscopy, laser-induced fluorescence, & IR imaging sensors for gas temperature, pressure, velocity, and chemical species
- Molecular spectroscopy, photophysics, & energy transfer in gases
- Energetic materials (e.g., explosives & propellants) detection & combustion
- Combustion and propulsion systems (small and large scale)
- Biomedical sensing
Marcial Gonzalez
- Predictive, multi-scale modeling and simulation of microstructure evolution in confined granular systems, with an emphasis in manufacturing processes and the relationship between product fabrication and performance.
- Application areas of interest include:
- (i) particulate products and processes (e.g., flow, mixing, segregation, consolidation, and compaction of powders),
- (ii) continuous manufacturing (e.g., Quality by Design, model predictive control, and reduced order models), and
- (iii) performance of pharmaceutical solid products (e.g., tensile strength, stiffness, swelling and disintegration), biomaterials (e.g., transport and feeding of corn stover) and energetic materials (e.g., deformation and heat generation under quasi-static, near-resonant and impact conditions, and formation and growth of hot spots) materials.
- Sustainable energy and environment
- Combustion and turbulent reacting flows
- Combustion and heat transfer in materials
- Biomedical flows and heat transfer
- Global policy research
Martin Byung-Guk Jun
- Advanced multi-scale manufacturing
- Ultrafast laser machining and processing
- Fiber optic sensors and environmental monitoring
- Spray-based nanoparticle coating and additive manufacturing
- Machining of carbon fiber reinforced polymer (CFRP) composites
Marisol Koslowski
- Computational solid mechanics
- Multiscale modeling of materials
- Finite Elements
- Dislocation dynamics
- Reliability of electronic interconnects
- Shock compression in solids
- Phase transformations
- Energetic materials
Chi Hwan Lee
- Wearable biomedical devices
- 'Crack’-driven transfer printing technology
- Scalable manufacturing technology
- Mechanics and materials for flexible/stretchable electronics
- Acoustic tweezers
- Acoustofluidics
- Acoustic metamaterials
- Ultrasound control
- Underwater communication
- Ultrasound imaging
- Multiphysics wave propagation theory
- Noise control and energy harvesting
- Naturally nanostructured materials
- Energy, water, and wearable technology
- Manufacturing
Ajay Malshe
- Bio-inspired designs
- Surface engineering and multifunctional materials
- Convergent Manufacturing for Industry 5.0: hybrid manufacturing processes, heterogeneous materials, and bio-inspired designs
- Systems integration, productization, and production
- Heavy-duty machines: machining, lubrication, and corrosion
- Heterogeneous and hierarchical integration (mechanical-electrical-optical and nano-micro-meso-macro)
- Precision agricultural and food: cellular agriculture, vertical farming, micro-production, and resilience
- Frugal engineering, social innovations, and social equity
- Manufacturing in space
Amy Marconnet
- Transport Phenomena in Multi-Scale, Heterogeneous Materials & Systems
- Fundamentals of Nanoscale Thermal Transport
- Heat Transfer in Natural and Synthetic Fiber Systems
- Thermofluids Interactions
- Multi-Physics Metrology Design
- Electronics Cooling and Thermal Management
Partha Mukherjee
- Energy storage and conversion (batteries, fuel cells)
- Mesoscale physics and stochastics
- Reactive transport, materials, processing, and microstructure interactions
- Scalable nanomanufacturing: lithography and imaging
- Optical and magnetic data storage
- Nanoscale energy conversion, transfer and storage for alternative energy
Xiulin Ruan
- Simulations of nanoscale thermal transport
- Machine learning, optimization, and high throughput design
- Thermal management in electronics, space, and battery applications
- Transport phenomena in additive manufacturing
- Nanomaterials and devices for sustainable energy
Farshid Sadeghi
- Contact mechanics
- Stresses, fatigue and friction of rolling/sliding
- Micro-mechanics of boundary and mixed lubrication regimes
- Spall initiation and propagation
- Surface science and damage
- Dynamics of ball and rolling element bearings and rotating systems
- Friction induced vibration and squeal in dry contacts
- Friction and wear of dry and lubricated contacts
- Virtual tribology
- Dry and lubricated fretting wear
- MEMS for in-situ monitoring of tribological contacts
- Discrete element modeling
Cagri Savran
- MEMS, nanotechnology
- Protein detection
- Aptamers (Nucleic-acid-based receptor molecules)
- Laser additive manufacturing
- Ultrafast laser matter interaction
- Laser welding
- Laser assisted machining
- Laser shock peening
- Multi-physics, multi-scale modeling
- Micro-nano manufacturing
- Multiphase combustion, particularly related to propellants, explosives, and pyrotechnics
- Nanoscale composite energetic materials
- Advanced energetic materials
- Microscale combustion
Ganesh Subbarayan
- Computational and experimental solid mechanics focused on fatigue, fracture, and multi-physics phase evolution problems
- Computational techniques including Finite Element Analysis (FEA), Isogeometric Analysis (IGA), geometric modeling, CAD and optimal design
- Heterogeneous Integration and Advanced Electronics Packaging with a focus on thermomechanical behavior, reliability, and electrical-thermal-mechanical co-design
Pavlos Vlachos
- Measurement science and instrumentation
- Particle image velocimetry
- Quantification of uncertainty
- Multi-phase flows
- Flow induced vibrations and hydro-kinetic energy
- Biological flows
- Biofluid mechanics
- Biomedical cardiovascular devices
- Heart failure and diastolic dysfunction
Ryan Wagner
- Scanning Probe Microscopy
- Optomechanics
- Mass spectrometry
David Warsinger
- Desalination & Water Treatment
- Water-Food-Energy Nexus
- Thermofluids
- Nanotechnology
- Membrane Science
Carl Wassgren
- Discrete element method (DEM) modeling for particulate systems
- -- model development, e.g., fibrous particles, particle breakage, particle shapes
- -- application to manufacturing, e.g., storage and flow, blending, segregation, drying, coating, wet granulation
- Finite element method (FEM) modeling of powder compaction
- -- e.g., roll compaction, tableting, picking and sticking
- Multi-scale modeling (FEM combined with DEM) of powder dynamics
- -- model development and application to hopper flow, blending, and segregation
- Heat Transfer in Advanced Semiconductor Interconnects & Packaging
- Electronic Cooling & Efficient Thermal Packaging Materials
- Thermal/Mechanical Simulation & Characterization
- Materials, Processing & Architecture Development for Semiconductor Packaging
- MEMS Fabrication for Extreme Heat Flux Microfluidic Cooling
- Advanced Semiconductor Nanoscale 3D Interconnections
- Novel Photonics & Quantum Packaging Technologies
- Reliability Modeling & Characterization
Justin Weibel
- Advanced electronics cooling and packaging technologies
- Phase-change transport phenomena
- Additive manufacturing of thermal management components
- Topological optimization and machine-learning-based design
- Thermal systems analysis for electrification and energy efficiency
- Microscale and nanoscale surface engineering for enhanced thermal transport
Steven Wereley
- Microfluidic MEMS devices
- Development of new microfluidic diagnostic techniques
- Biological flows at the cellular level
- Micro-scale laminar mixing
- Flow transitions and instabilities
- Laser-matter interactions
- Laser-induced plasma and laser-plasma interaction
- Laser applications in manufacturing, materials processing, and other areas
- Heat transfer, particularly nano-scale and ultrafast heat transfer
- Ultrafast laser materials processing and diagnostics
- Nano-optics and laser-based nano-lithography
Huidan (Whitney) Yu
- Image-based computational and experimental fluid dynamics for porous-media and biomedical flows
- Translational research integrating high-performance CFD, image-based and physics-informed machine-learning, and uncertainty quantification to address unmet clinical needs
- GPU-parallelized lattice Boltzmann method for DNS and LES of turbulence
- Micro-bubble coalescence and detachment in microfluidics
- Multiscale superfast 3D optical sensing
- Biophotonic imaging
- Optical metrology
- Machine/computer vision
- 3D video telepresence
- 3D video processing
- Virtual reality
- Human computer interaction
- Deformation, stress, plasticity, fracture
- Multiscale modeling, first-principles, molecular dynamics simulations, and finite element modeling
- In-situ experiments
- Mechanics of redox active materials - Li-ion batteries, Na-ion batteries, all-solid-state batteries
- Mechanics of polymeric materials - organic electrochromics, superelastic organic semiconductors
I want to research in these fundamental areas...
I want to have an impact in....
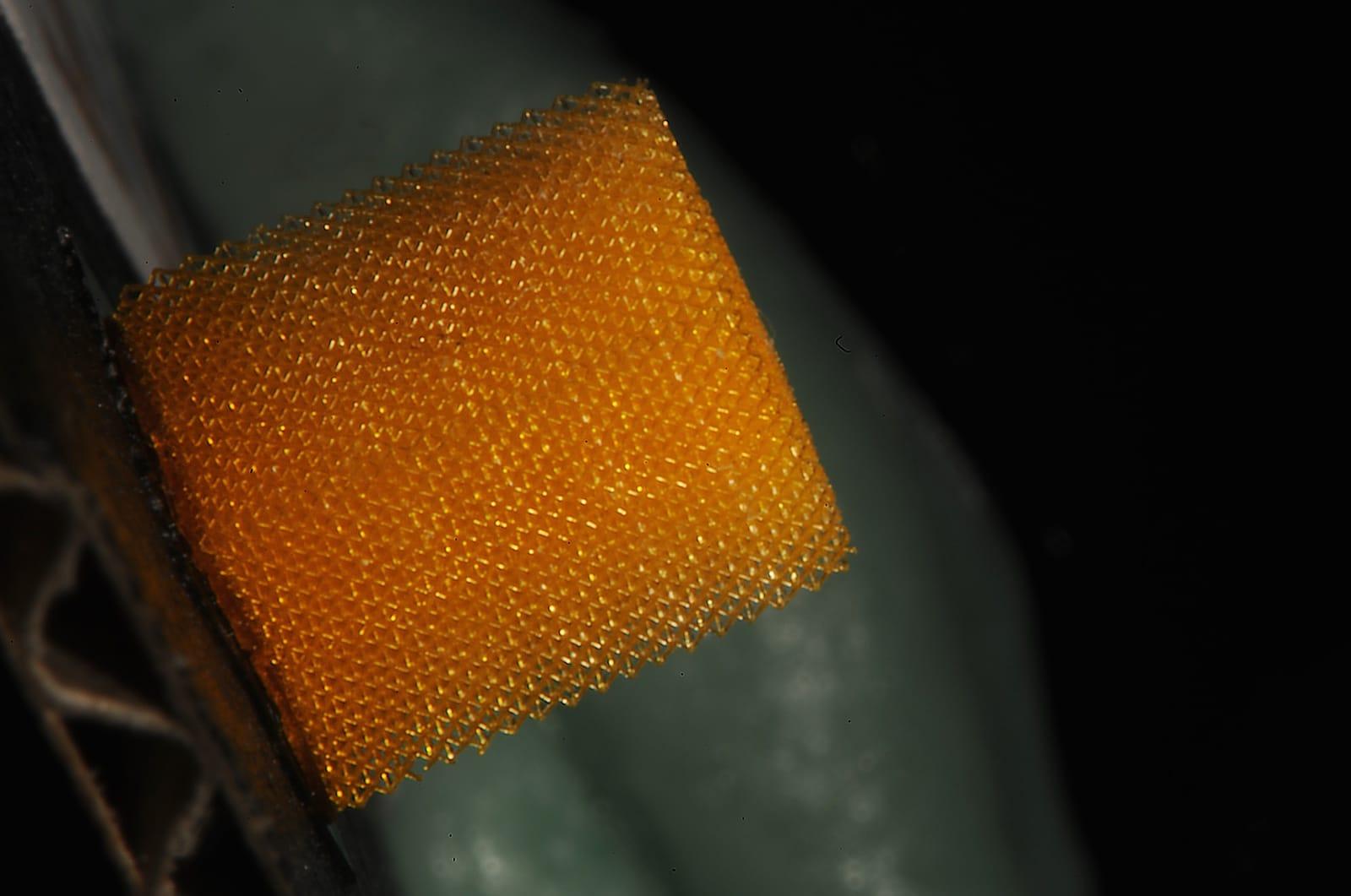
RESEARCH @ MIT MECHE
Micro + nano.
In the micro/nano area, tiny devices offer giant opportunities for technology, consumer products, energy systems, and more.
Scroll to Explore
Explore nano Research
- News + Media
- Featured Labs
Micro and Nanotechnology
The micro/nano area enables airbag sensors, disposable medical sensors, rapid DNA analysis, stronger and lighter materials for transportation, and energy-efficient conversion devices, to name a few.
Research Includes: Micro/nano-enabled energy and power technology, nanobiotechnology, nano/micro design and manufacturing, micro/nanoscale mechanics and materials, micro/nanophotonics, micro/nanoscale transport
Micro And Nanotechnology News + Media
Discovering the Micro/Nano World
2.674: One of the first classes to offer undergraduates a hands-on experience with cutting-edge micro/nano engineering.
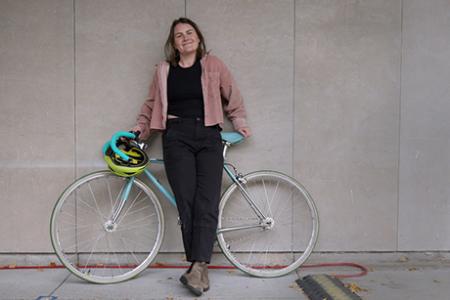
The MIT Bike Lab: A place for community, hands-on learning
MIT's Bike Lab, an all-volunteer student-run bike shop, founded by graduate student Bianca Champenois, provides repair and maintenance services, emphasizes hands-on learning, and promotes sustainable transportation.
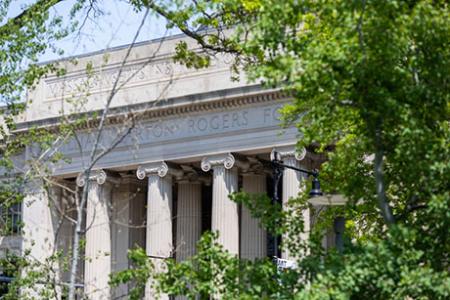
QS World University Rankings rates MIT No. 1 in 11 subjects for 2024
QS World University Rankings has placed MIT in the No. 1 spot in 11 subject areas including Mechanical Engieering for 2024
Micro And Nanotechnology Lab Spotlight
Visit our Micro And Nanotechnology lab sites to learn more about our faculty’s research projects.
- Device Research Laboratory
- Electrochemical Energy Lab
- Mechanosynthesis Group
- Microfluidics and Nanofluidics Research Laboratory
- MicroNanoSystems Lab
- Nanoelectronics Laboratory
- Nanophotonics and 3D Nanomanufacturing Laboratory
- Nanoscale Kinetic Transport and Multiscale Simulation Group
Meet Some of Our Faculty Working On Micro And Nanotechnology Challenges
MechE faculty are passionate, out-of-the-box thinkers who love to get their hands dirty.
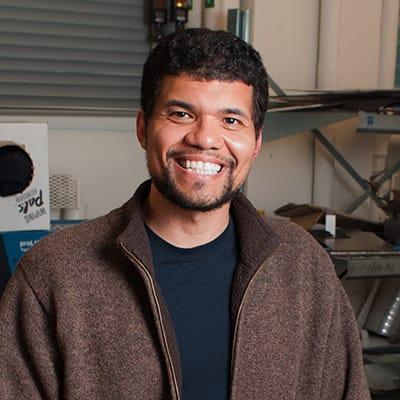
- bioengineering
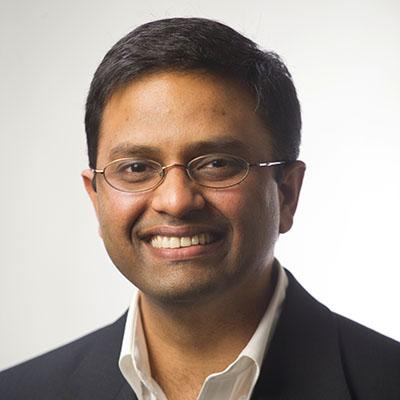
Selected Course Offerings in Micro And Nanotechnology
Learn about the impact of our nano research.
Research areas in MechE are guidelines, not boundaries. Our faculty partner across disciplines to address the grand challenges of today and tomorrow, collaborating with researchers in MechE, MIT, industry, and beyond.
- Impact Health
- Impact Environment
- Impact Innovation
- Impact Security
- Impact Energy

- Current Issues
- Past Issues
- Call for Papers
- Research Topics
Publication Procedure
- Processing Fees
- Editorial Board
The Applications of Nanotechnology in Mechanical Engineering
- File Size 435.78 KB
- File Count 1
- Create Date 29/07/2024
- Last Updated 30/07/2024
Mukesh Devpura,
Post Guest Faculty, CTAE,
Department of Mechanical Engineering,
Abstract— Nanotechnology has become an all-embracing term, which means different things to different people. Nanotechnology is interface technologies that are include many different science and applications area. Nanotechnology falls into this category and offers fundamentally new capabilities to architect a board array of the novel materials, composites and structure on a molecular scale. Here discusses on some of the applications for nanotechnology and shows a few cases of them. That is believed to have the highest probability of success in competitive industry. The nanotechnology that are economically promising for the future include those that have applications in information technology, electronics, building materials, household appliances, textiles, cosmetics, food, environmental technologies, energy technologies and medicine etc.,
Keywords-NanoTechnology,Nanomaterials,Nanocomposite
Share your Published Research Paper Now

What is DOI
Frequently asked questions.
A DOI will help Author(s) easily locate a document from your citation. Think of it like a Social Security number for the article you're citing — it will always refer to that article, and only that one. While a web address (URL) might change, the DOI will never change.
Where can i find DOI:
- In IJSREM journal articles, the DOI will be printed with the article itself, usually on the footer of the page
- If the DOI isn't included in the article, look it up on the website CrossRef.org (use the "Search Metadata" option) to check for an assigned DOI.
- Allows for a quick and precise search.
- Article can always be located.
- Persistent link to its location on the Internet.
- Easier identification of published articles even if the metadata URL is changed.
- Aid in citation tracking, ensuring a researcher has accurate metrics on how and where their research outputs are being used or referenced.
What type of papers does International Journal of Scientific Research in Engineering and Management (IJSREM) publish?
Research Paper, Survey Paper, Informative Article, Case Studies, Review Papers, Comparative Studies
How long does it take for an accepted paper to be actually published?
Approximate will inform within 24 hours via email and sms.
What is the procedure to submit my paper?
Please Submit your Research Paper here Submit Research Paper Online - IJSREM
What is a Paper ID?
Paper ID is an Unique Identification Code provided to your submitted Manuscript. You should always mention the Paper ID during any communication with us.
How long my published paper will stay online?
May I submit more than one article?
We will accept multiple submissions across multiple communities, as long as the author joins each community.
Why IJSREM?
IJSREM is one of the world's leading and fastest-growing research publications with the paramount objective of discovering advances by publishing insightful, double-blind, peer-reviewed scientific journals.
Publication Time Period
The Submitted Article/Paper will accept within 12-24 Hours, and it can publish within 24 Hours
Manuscript Submission - Check Manuscript Plagiarism - Send for Editorial Review - Final Decission to Author - Payment of Publication Fee - Re-screening - Final Publication
Processing Fee's
For Management, Hosting & Office Expenditure IJSREM Journal may charge some amount to publish the paper.
To Publish a Paper ₹: 800/- INR (without DOI)
₹:1000/- INR (with DOI)
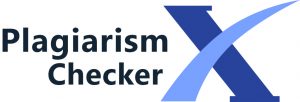
To the extent possible under, Indospace Publications has waived all copyright and related or neighboring rights to Journal. This work is published from India.
Disclaimar Privacy Policy Terms and Conditions
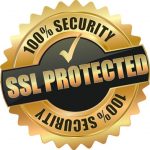
Journal of Applied Mechanical Engineering
ISSN: 2168-9873
Google Scholar citation report
Citations : 1390.
Journal of Applied Mechanical Engineering received 1390 citations as per Google Scholar report
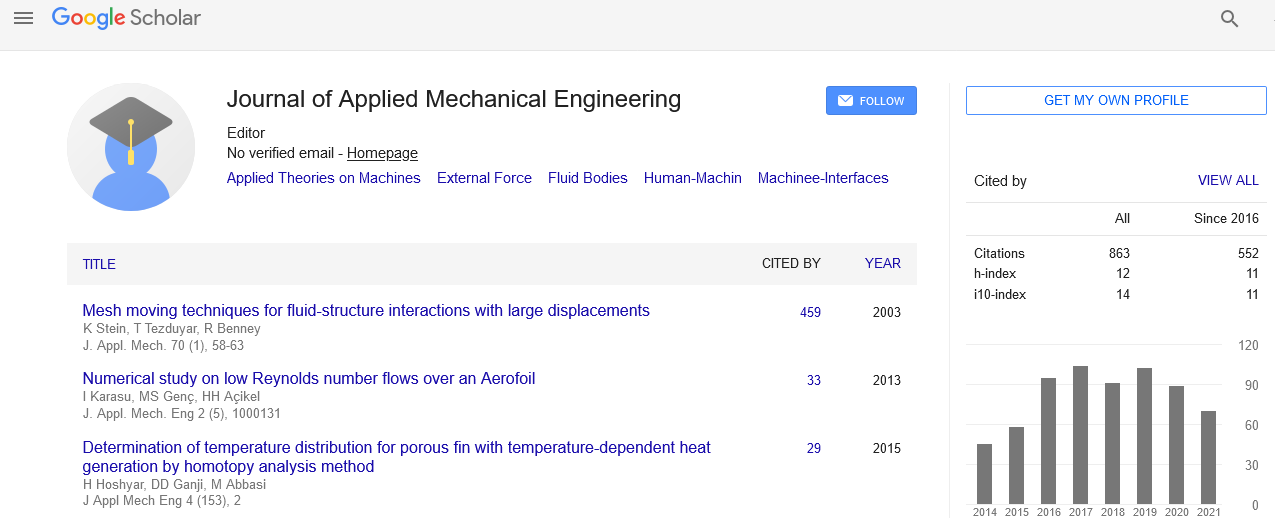
25+ Million Website Visitors
- Genamics JournalSeek
- JournalTOCs
- Hamdard University
- OCLC- WorldCat
- Google Scholar
Useful Links
- Aim and Scope
- Peer Review Process
- Citations Report
- Indexing and Archiving
- Table of Contents
- Submit Paper
- Track Your Paper
- Funded Work
Share This Page
Journal flyer.
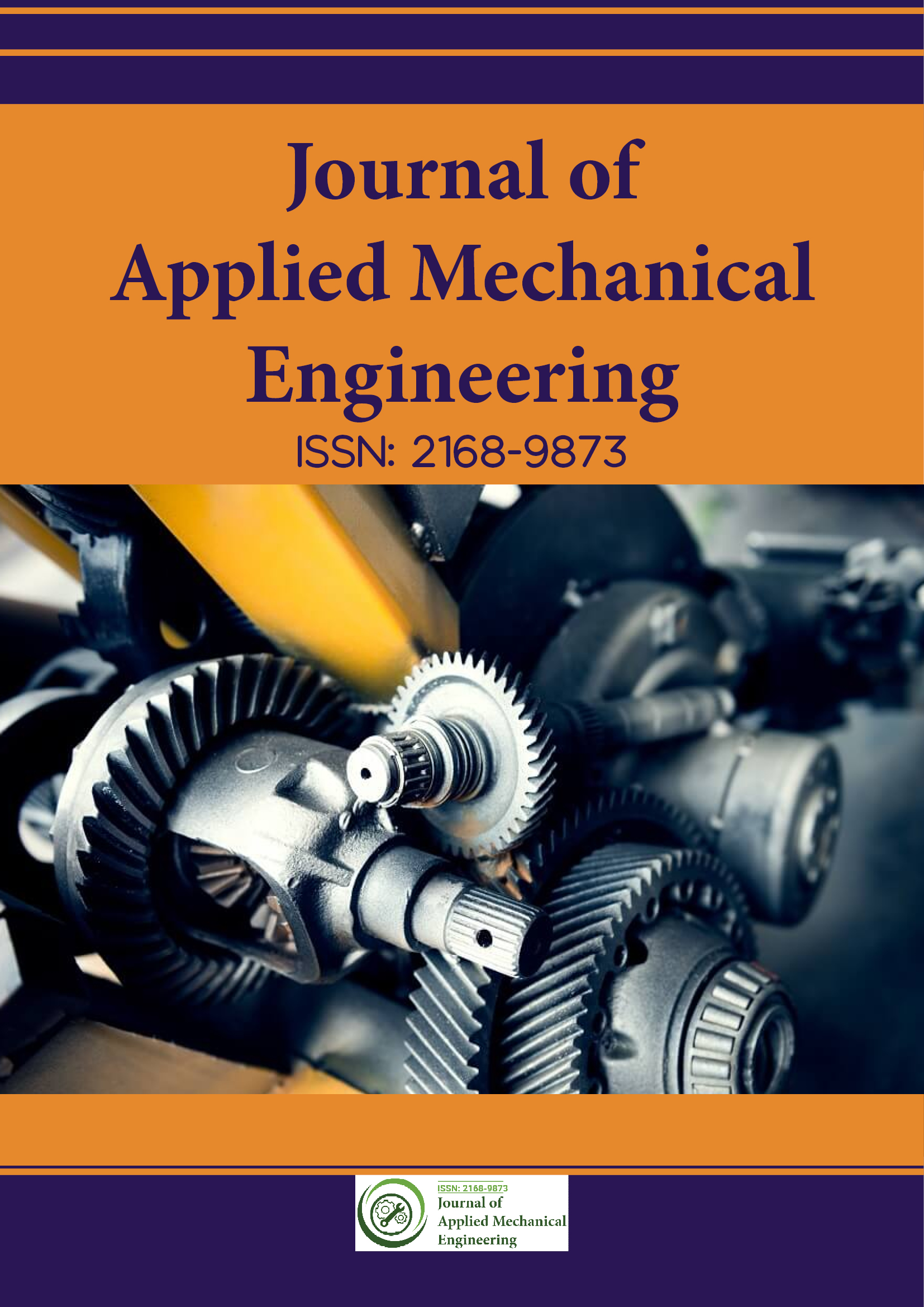
Open Access Journals
- Agri and Aquaculture
- Biochemistry
- Bioinformatics & Systems Biology
- Business & Management
- Clinical Sciences
- Engineering
- Food & Nutrition
- General Science
- Genetics & Molecular Biology
- Immunology & Microbiology
- Medical Sciences
- Neuroscience & Psychology
- Nursing & Health Care
- Pharmaceutical Sciences
Perspective - (2022) Volume 11, Issue 7
- Download PDF
Significance of Nanotechnology in Mechanical Engineering
Received: 16-Jul-2022, Manuscript No. JAME-22-17821; Editor assigned: 18-Jul-2022, Pre QC No. JAME-22-17821 (PQ); Reviewed: 05-Aug-2022, QC No. JAME-22-17821; Revised: 16-Aug-2022, Manuscript No. JAME-22-17821 (R); Published: 26-Aug-2022, DOI: 10.35248/2168-9873.22.11.429
Description
The utilisation of matter on an atomic, molecular and supramolecular scale for industrial purposes is known as nanotechnology, usually abbreviated as nanotech. The first widely accepted definition of nanotechnology refers to the specific technological objective of accurately manipulating atoms and molecules for the creation of macroscale goods, which is now known as molecular nanotechnology. The National Nanotechnology Initiative later adopted a more broad definition of nanotechnology, defining it as the manipulation of matter with at least one dimension scaled from 1 to 100 nanometers. Because quantum mechanical effects are important at this quantum-realm scale, the definition has shifted from a specific technological goal to a research category that includes all types of research and technologies that deal with the special properties of matter that occur below the given size threshold. As a result, the plural form "nanotechnologies" as well as "nanoscale technologies" are frequently used to refer to the vast spectrum of research and applications that share the property of size.
Uses of nanotecnology in mechanical engineering
Nanotechnology in mechanical engineering and production is extremely beneficial to the industry. Nanotechnology can be utilised to extend the life of car components and parts. Nanotechnology has the potential to improve a wide range of materials. Nanomaterials have distinct physical and chemical features that improve manmade materials. There are improvements in magnetic characteristics, mechanical activity, and optical properties. Improvements are being made to improve the properties of the materials and to develop alternative precursors that can give the materials suitable features. Nanotechnology entails the ability to observe and control individual atoms and molecules; atoms make up everything on Earth.
The microscopes required to see things at the nanoscale were only recently invented. Despite the fact that modern nanoscience and nanotechnology are relatively new, tiny materials have been used for centuries. Today's scientists and engineers are experimenting with a wide range of nanomaterials to take advantage of their increased capabilities such as stronger strength, less weight, enhanced light spectrum control, and more chemical reactivity than their large-scale counterparts.
Application of nanotechnology
The world of materials is being transformed by nanotechnology. It has a significant impact on the development of a new generation of composites with improved functionality and a wide range of applications. The information on processing, characterisation, and applications assists researchers in comprehending and applying the unique chemical and material principles underpinning these cutting-edge polymer nanocomposites.
Citation: Rodrigues H (2022) Significance of Nanotechnology in Mechanical Engineering. J Appl Mech Eng. 11:429.
Copyright: © 2022 Rodrigues H. This is an open-access article distributed under the terms of the Creative Commons Attribution License, which permits unrestricted use, distribution, and reproduction in any medium, provided the original author and source are credited.
- +44 1687550021
- Guidelines for Featured Authors
- Consulting Services
- Privacy Policy
Advances in Engineering Advances in Engineering features breaking research judged by Advances in Engineering advisory team to be of key importance in the Engineering field. Papers are selected from over 10,000 published each week from most peer reviewed journals.

Nanotechnology Engineering
Enhanced photoemission efficiency of hot electrons in au nanodisk-cluster complexes on tio2.
July 13, 2024
Portable Triboelectric Electrostatic Tweezer for Precise Non-Contact Droplet Manipulation in Enclosed Systems
June 27, 2024

Dual-Wavelength Metasurfaces for Switchable 2D AND and XOR Logic Operations: Implications for Optical Computing and Information Encryption
Applying electric field to assemble nanostructured filaments for sustainable electronics.
June 11, 2024

Enhanced Persistent Luminescence and Uniformity of ZGGO Nanoparticles via Nonstoichiometric Reactions for Advanced Bioimaging and Biosensing Applications
June 9, 2024
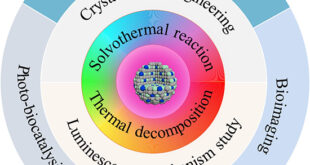
Advancements in 2D-Ordered Linear Chain Carbon for Next-Generation Sensing Technologies
June 6, 2024
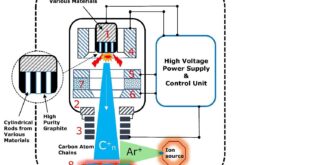
Breaking Bubbles: The Isothermal Illusion in Nanobubble Dynamics
June 2, 2024

Carbon Capture: Garlic Roots Power Breakthrough in Supercapacitive Swing Adsorption
June 1, 2024

Surfing the Nano Wave: Dramatic Leap in DNA Sensing with Surfactant-Coated Nanopores

Evolution of Molybdenum Catalysts in Carbon Nanotube Synthesis
Designing a quasi-liquid alloy interface for solid na-ion battery.
May 28, 2024

Revolutionizing Hydrogen Peroxide Detection: An Innovative Cascade Enzyme System for Enhanced Sensitivity and Range
May 27, 2024

A Nano-Composite Electrochemical Sensor for Detecting Reactive Oxygen Species (ROS)
May 25, 2024
Complex Polymer Nanoparticle Synthesis and Morphology Control Using an Inkjet Mixing System
May 24, 2024
Scalable Synthesis of 2D Silicon Oxide Nanomaterials
May 23, 2024


Powering the Future: Nanostructured Triboelectric Nanogenerators Revolutionize Energy Harvesting

Substrate Vibration Accelerates Film Dewetting: Insights from Molecular Dynamics Simulations
Thank you for visiting nature.com. You are using a browser version with limited support for CSS. To obtain the best experience, we recommend you use a more up to date browser (or turn off compatibility mode in Internet Explorer). In the meantime, to ensure continued support, we are displaying the site without styles and JavaScript.
- View all journals
- Explore content
- About the journal
- Publish with us
- Sign up for alerts
- Published: 24 July 2024
Electrically tunable space–time metasurfaces at optical frequencies
- Jared Sisler ORCID: orcid.org/0000-0002-0660-7909 1 na1 ,
- Prachi Thureja ORCID: orcid.org/0000-0003-3852-3395 1 na1 ,
- Meir Y. Grajower 1 ,
- Ruzan Sokhoyan ORCID: orcid.org/0000-0003-4599-6350 1 ,
- Ivy Huang ORCID: orcid.org/0000-0001-8911-3949 1 &
- Harry A. Atwater ORCID: orcid.org/0000-0001-9435-0201 1
Nature Nanotechnology ( 2024 ) Cite this article
2217 Accesses
132 Altmetric
Metrics details
- Metamaterials
- Nanophotonics and plasmonics
Active metasurfaces enable dynamic manipulation of the scattered electromagnetic wavefront by spatially varying the phase and amplitude across arrays of subwavelength scatterers, imparting momentum to outgoing light. Similarly, periodic temporal modulation of active metasurfaces allows for manipulation of the output frequency of light. Here we combine spatial and temporal modulation in electrically modulated reflective metasurfaces operating at 1,530 nm to generate and diffract a spectrum of sidebands at megahertz frequencies. Temporal modulation with tailored waveforms enables the design of a spectrum of sidebands. By impressing a spatial phase gradient on the metasurface, we can diffract selected combinations of sideband frequencies. Combining active temporal and spatial variation can enable unique optical functions, such as frequency mixing, harmonic beam steering or shaping, and breaking of Lorentz reciprocity.
This is a preview of subscription content, access via your institution
Access options
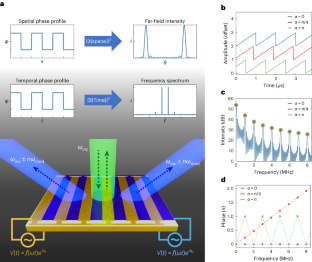
Similar content being viewed by others
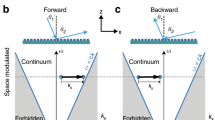
Nonreciprocal metasurface with space–time phase modulation
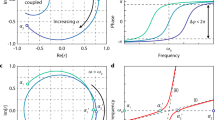
Full 2π tunable phase modulation using avoided crossing of resonances
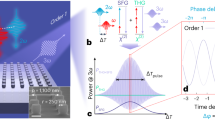
All-optical free-space routing of upconverted light by metasurfaces via nonlinear interferometry
Data availability.
The authors declare that data supporting the findings of this study are available within the article and its Supplementary Information files. All the relevant data are available from the corresponding author upon request.
Yu, N. & Capasso, F. Flat optics with designer metasurfaces. Nat. Mater. 13 , 139–150 (2014).
Article CAS PubMed Google Scholar
Sun, S. et al. High-efficiency broadband anomalous reflection by gradient meta-surfaces. Nano Lett. 12 , 6223–6229 (2012).
Wong, A. M. H. & Eleftheriades, G. V. Perfect anomalous reflection with a bipartite Huygens’ metasurface. Phys. Rev. X 8 , 011036 (2018).
CAS Google Scholar
Wang, X. et al. Simultaneous realization of anomalous reflection and transmission at two frequencies using bi-functional metasurfaces. Sci. Rep. 8 , 1876 (2018).
Article PubMed PubMed Central Google Scholar
Khorasaninejad, M. et al. Metalenses at visible wavelengths: diffraction-limited focusing and subwavelength resolution imaging. Science 352 , 1190–1194 (2016).
Chen, W. T. et al. A broadband achromatic metalens for focusing and imaging in the visible. Nat. Nanotechnol. 13 , 220–226 (2018).
Arbabi, A., Horie, Y., Ball, A. J., Bagheri, M. & Faraon, A. Subwavelength-thick lenses with high numerical apertures and large efficiency based on high-contrast transmitarrays. Nat. Commun. 6 , 7069 (2015).
Deng, Y., Wu, C., Meng, C., Bozhevolnyi, S. I. & Ding, F. Functional metasurface quarter-wave plates for simultaneous polarization conversion and beam steering. ACS Nano 15 , 18532–18540 (2021).
Devlin, R. C., Ambrosio, A., Rubin, N. A., Mueller, J. P. B. & Capasso, F. Arbitrary spin-to–orbital angular momentum conversion of light. Science 358 , 896–901 (2017).
Balthasar Mueller, J. P., Rubin, N. A., Devlin, R. C., Groever, B. & Capasso, F. Metasurface polarization optics: Independent phase control of arbitrary orthogonal states of polarization. Phys. Rev. Lett. 118 , 113901 (2017).
Hail, C. U., Michel, A. U., Poulikakos, D. & Eghlidi, H. Optical metasurfaces: evolving from passive to adaptive. Adv. Opt. Mater. 7 , 1801786 (2019).
Article Google Scholar
Thureja, P. et al. Toward a universal metasurface for optical imaging, communication, and computation. Nanophotonics 11 , 3745–3768 (2022).
Article CAS Google Scholar
Yang, J., Gurung, S., Bej, S., Ni, P. & Howard Lee, H. W. Active optical metasurfaces: comprehensive review on physics, mechanisms, and prospective applications. Rep. Prog. Phys. 85 , 036101 (2022).
Huang, Y. W. et al. Gate-tunable conducting oxide metasurfaces. Nano Lett. 16 , 5319–5325 (2016).
Wu, P. C. et al. Dynamic beam steering with all-dielectric electro-optic III–V multiple-quantum-well metasurfaces. Nat. Commun. 10 , 3654 (2019).
Shcherbakov, M. R. et al. Ultrafast all-optical tuning of direct-gap semiconductor metasurfaces. Nat. Commun. 8 , 17 (2017).
Klopfer, E., Delgado, H. C., Dagli, S., Lawrence, M. & Dionne, J. A. A thermally controlled high-Q metasurface lens. Appl. Phys. Lett. 122 , 221701 (2023).
Ou, J. Y., Plum, E., Jiang, L. & Zheludev, N. I. Reconfigurable photonic metamaterials. Nano Lett. 11 , 2142–2144 (2011).
Arbabi, E. et al. MEMS-tunable dielectric metasurface lens. Nat. Commun. 9 , 812 (2018).
Eaves-Rathert, J. et al. Dynamic color tuning with electrochemically actuated TiO 2 metasurfaces. Nano Lett. 22 , 1626–1632 (2022).
Shirmanesh, G. K., Sokhoyan, R., Wu, P. C. & Atwater, H. A. Electro-optically tunable multifunctional metasurfaces. ACS Nano 14 , 6912–6920 (2020).
Tirole, R. et al. Double-slit time diffraction at optical frequencies. Nat. Phys. 19 , 999–1002 (2023).
Wu, Z. & Grbic, A. Serrodyne frequency translation using time-modulated metasurfaces. IEEE Trans. Antennas Propag. 68 , 1599–1606 (2020).
Zhang, L. et al. Space–time-coding digital metasurfaces. Nat. Commun. 9 , 4334 (2018).
Cardin, A. E. et al. Surface-wave-assisted nonreciprocity in spatio-temporally modulated metasurfaces. Nat. Commun. 11 , 1469 (2020).
Article CAS PubMed PubMed Central Google Scholar
Guo, X., Ding, Y., Duan, Y. & Ni, X. Nonreciprocal metasurface with space–time phase modulation. Light. Sci. Appl. 8 , 123 (2019).
Shaltout, A., Kildishev, A. & Shalaev, V. Time-varying metasurfaces and Lorentz non-reciprocity. Opt. Mater. Express 5 , 2459 (2015).
Hadad, Y., Sounas, D. L. & Alu, A. Space–time gradient metasurfaces. Phys. Rev. B 92 , 100304(R) (2015).
Taravati, S. & Eleftheriades, G. V. Generalized space–time-periodic diffraction gratings: theory and applications. Phys. Rev. Appl. 12 , 024026 (2019).
Wang, X., Asadchy, V. S., Fan, S. & Tretyakov, S. A. Space–time metasurfaces for power combining of waves. ACS Photonics 8 , 3034–3041 (2021).
Salary, M. M. & Mosallaei, H. Time-modulated conducting oxide metasurfaces for adaptive multiple access optical communication. IEEE Trans. Antennas Propag. 68 , 1628–1642 (2020).
Sabri, R., Salary, M. M. & Mosallaei, H. Quasi‐static and time‐modulated optical phased arrays: beamforming analysis and comparative study. Adv. Photonics Res. 2 , 2100034 (2021).
Taravati, S., Chamanara, N. & Caloz, C. Nonreciprocal electromagnetic scattering from a periodically space–time modulated slab and application to a quasisonic isolator. Phys. Rev. B 96 , 165144 (2017).
Taravati, S. Aperiodic space–time modulation for pure frequency mixing. Phys. Rev. B 97 , 115131 (2018).
Tien, P. K. Parametric amplification and frequency mixing in propagating circuits. J. Appl. Phys. 29 , 1347–1357 (1958).
Zhang, L. & Cui, T. J. Space-time-coding digital metasurfaces: principles and applications. Research 2021 , 9802673 (2021).
Zhang, L. et al. Breaking reciprocity with space–time‐coding digital metasurfaces. Adv. Mater. 31 , 1904069 (2019).
Karimi, M., Alam, M. Z., Upham, J., Reshef, O. & Boyd, R. W. Time-varying gradient metasurface with applications in all-optical beam steering. Nanophotonics 12 , 1733–1740 (2023).
Lustig, E. et al. Time-refraction optics with single cycle modulation. Nanophotonics 12 , 2221–2230 (2023).
Salary, M. M., Farazi, S. & Mosallaei, H. A dynamically modulated all‐dielectric metasurface doublet for directional harmonic generation and manipulation in transmission. Adv. Opt. Mater. 7 , 1900843 (2019).
Kafaie Shirmanesh, G., Sokhoyan, R., Pala, R. A. & Atwater, H. A. Dual-gated active metasurface at 1550 nm with wide (>300°) phase tunability. Nano Lett. 18 , 2957–2963 (2018).
Amin, R. et al. Heterogeneously integrated ITO plasmonic Mach–Zehnder interferometric modulator on SOI. Sci. Rep. 11 , 1287 (2021).
Lu, B., Lv, H., Zhang, Y., Zhang, Y. & Liu, C. Comparison of HfAlO, HfO2/Al2O3, and HfO2 on n-type GaAs using atomic layer deposition. Superlattices Microstruct. 99 , 54–57 (2016).
Gui, Y. et al. 100 GHz micrometer-compact broadband monolithic ITO Mach–Zehnder interferometer modulator enabling 3500 times higher packing density. Nanophotonics 11 , 4001–4009 (2022).
Amin, R. et al. Sub-wavelength GHz-fast broadband ITO Mach–Zehnder modulator on silicon photonics. Optica 7 , 333 (2020).
Sabri, R., Salary, M. M. & Mosallaei, H. Broadband continuous beam-steering with time-modulated metasurfaces in the near-infrared spectral regime. APL Photonics 6 , 086109 (2021).
Castaldi, G. et al. Joint multi‐frequency beam shaping and steering via space–time‐coding digital metasurfaces. Adv. Funct. Mater. 31 , 2007620 (2021).
Xu, B. H. et al. Multichannel metasurfaces with frequency‐direction multiplexed amplitude and phase modulations. Adv. Opt. Mater. 11 , 2301299 (2023).
Sabri, R., Forouzmand, A. & Mosallaei, H. Multi-wavelength voltage-coded metasurface based on indium tin oxide: independently and dynamically controllable near-infrared multi-channels. Opt. Express 28 , 3464 (2020).
Article PubMed Google Scholar
Taravati, S. & Eleftheriades, G. V. Microwave space–time-modulated metasurfaces. ACS Photonics 9 , 305–318 (2022).
Hadad, Y., Soric, J. C. & Alu, A. Breaking temporal symmetries for emission and absorption. Proc. Natl Acad. Sci. USA 113 , 3471–3475 (2016).
Taravati, S. & Eleftheriades, G. V. Full-duplex nonreciprocal beam steering by time-modulated phase-gradient metasurfaces. Phys. Rev. Appl. 14 , 014027 (2020).
Benea-Chelmus, I.-C. et al. Gigahertz free-space electro-optic modulators based on Mie resonances. Nat. Commun. 13 , 3170 (2022).
Download references
Acknowledgements
This work was supported by the Air Force Office of Scientific Research Meta-Imaging MURI grant no. FA9550-21-1-0312 (J.S., P.T., M.G., R.S., I.H. and H.A.A.) and DARPA EXTREME (H.A.A.). J.S. acknowledges the support of the Natural Sciences and Engineering Research Council of Canada (NSERC) through the Postgraduate Scholarship – Doctoral program. P.T. acknowledges support from Meta Platforms, Inc., through the PhD fellowship #C-834952. We are grateful to Caltech colleagues O. Painter and A. Emami for support with waveform generation instrumentation. We further thank C. U. Hail, M. D. Kelzenberg and P. R. Jahelka for technical support and discussions.
Author information
These authors contributed equally: Jared Sisler, Prachi Thureja.
Authors and Affiliations
Thomas J. Watson Laboratories of Applied Physics, California Institute of Technology, Pasadena, CA, USA
Jared Sisler, Prachi Thureja, Meir Y. Grajower, Ruzan Sokhoyan, Ivy Huang & Harry A. Atwater
You can also search for this author in PubMed Google Scholar
Contributions
J.S., P.T., M.Y.G., R.S. and H.A.A. conceived the ideas for this research project. J.S. and P.T. fabricated and characterized the active metasurfaces. J.S. and M.Y.G. built the experimental setup for time-modulation. J.S. and P.T. performed quasi-static and time-modulated measurements. J.S. built the setup for and performed the space–time measurements. J.S. created the real-time waveform optimization algorithm, with inputs from P.T. J.S. and M.Y.G. performed the space–time calculations. I.H. and P.T. designed the electrical PCB. All authors contributed to the writing of the manuscript.
Corresponding author
Correspondence to Harry A. Atwater .
Ethics declarations
Competing interests.
The authors declare no competing interests.
Peer review
Peer review information.
Nature Nanotechnology thanks Patrice Genevet, Guangwei Hu and Xingjie Ni for their contribution to the peer review of this work.
Additional information
Publisher’s note Springer Nature remains neutral with regard to jurisdictional claims in published maps and institutional affiliations.
Supplementary information
Supplementary information.
Supplementary Sections 1–9 including detailed discussion and Supplementary Figs. 1–8.
Rights and permissions
Springer Nature or its licensor (e.g. a society or other partner) holds exclusive rights to this article under a publishing agreement with the author(s) or other rightsholder(s); author self-archiving of the accepted manuscript version of this article is solely governed by the terms of such publishing agreement and applicable law.
Reprints and permissions
About this article
Cite this article.
Sisler, J., Thureja, P., Grajower, M.Y. et al. Electrically tunable space–time metasurfaces at optical frequencies. Nat. Nanotechnol. (2024). https://doi.org/10.1038/s41565-024-01728-9
Download citation
Received : 04 November 2023
Accepted : 23 June 2024
Published : 24 July 2024
DOI : https://doi.org/10.1038/s41565-024-01728-9
Share this article
Anyone you share the following link with will be able to read this content:
Sorry, a shareable link is not currently available for this article.
Provided by the Springer Nature SharedIt content-sharing initiative
Quick links
- Explore articles by subject
- Guide to authors
- Editorial policies
Sign up for the Nature Briefing newsletter — what matters in science, free to your inbox daily.


An official website of the United States government
The .gov means it’s official. Federal government websites often end in .gov or .mil. Before sharing sensitive information, make sure you’re on a federal government site.
The site is secure. The https:// ensures that you are connecting to the official website and that any information you provide is encrypted and transmitted securely.
- Publications
- Account settings
Preview improvements coming to the PMC website in October 2024. Learn More or Try it out now .
- Advanced Search
- Journal List

Nanotechnology: A Revolution in Modern Industry
Shiza malik.
1 Bridging Health Foundation, Rawalpindi 46000, Pakistan
Khalid Muhammad
2 Department of Biology, College of Science, UAE University, Al Ain 15551, United Arab Emirates
Yasir Waheed
3 Office of Research, Innovation, and Commercialization (ORIC), Shaheed Zulfiqar Ali Bhutto Medical University (SZABMU), Islamabad 44000, Pakistan
4 Gilbert and Rose-Marie Chagoury School of Medicine, Lebanese American University, Byblos 1401, Lebanon
Associated Data
Not applicable.
Nanotechnology, contrary to its name, has massively revolutionized industries around the world. This paper predominantly deals with data regarding the applications of nanotechnology in the modernization of several industries. A comprehensive research strategy is adopted to incorporate the latest data driven from major science platforms. Resultantly, a broad-spectrum overview is presented which comprises the diverse applications of nanotechnology in modern industries. This study reveals that nanotechnology is not limited to research labs or small-scale manufacturing units of nanomedicine, but instead has taken a major share in different industries. Companies around the world are now trying to make their innovations more efficient in terms of structuring, working, and designing outlook and productivity by taking advantage of nanotechnology. From small-scale manufacturing and processing units such as those in agriculture, food, and medicine industries to larger-scale production units such as those operating in industries of automobiles, civil engineering, and environmental management, nanotechnology has manifested the modernization of almost every industrial domain on a global scale. With pronounced cooperation among researchers, industrialists, scientists, technologists, environmentalists, and educationists, the more sustainable development of nano-based industries can be predicted in the future.
1. Introduction
Nanotechnology has slowly yet deeply taken over different industries worldwide. This rapid pace of technological revolution can especially be seen in the developed world, where nano-scale markets have taken over rapidly in the past decade. Nanotechnology is not a new concept since it has now become a general-purpose technology. Four generations of nanomaterials have emerged on the surface and are used in interdisciplinary scientific fields; these are active and passive nanoassemblies, general nanosystems, and small-scale molecular nanosystems [ 1 ].
This rapid development of nanoscience is proof that, soon, nano-scale manufacturing will be incorporated into almost every domain of science and technology. This review article will cover the recent advanced applications of nanotechnology in different industries, mainly agriculture, food, cosmetics, medicine, healthcare, automotive, oil and gas industries, chemical, and mechanical industries [ 2 , 3 ]. Moreover, a brief glimpse of the drawbacks of nanotechnology will be highlighted for each industry to help the scientific community become aware of the ills and benefits of nanotechnology side by side. Nanotechnology is a process that combines the basic attributes of biological, physical, and chemical sciences. These processes occur at the minute scale of nanometers. Physically, the size is reduced; chemically, new bonds and chemical properties are governed; and biological actions are produced at the nano scale, such as drug bonding and delivery at particular sites [ 4 , 5 ].
Nanotechnology provides a link between classical and quantum mechanics in a gray area called a mesoscopic system. This mesoscopic system is being used to manufacture nanoassemblies of nature such as agricultural products, nanomedicine, and nanotools for treatment and diagnostic purposes in the medical industry [ 6 ]. Diseases that were previously untreatable are now being curtailed via nano-based medications and diagnostic kits. This technology has greatly affected bulk industrial manufacturing and production as well. Instead of manufacturing materials by cutting down on massive amounts of material, nanotechnology uses the reverse engineering principle, which operates in nature. It allows the manufacturing of products at the nano scale, such as atoms, and then develops products to work at a deeper scale [ 7 ].
Worldwide, millions and billions of dollars and euros are being spent in nanotechnology to utilize the great potential of this new science, especially in the developed world in Europe, China, and America [ 8 ]. However, developing nations are still lagging behind as they are not even able to meet the industrial progression of the previous decade [ 9 ]. This lag is mainly because these countries are still fighting economically, and they need some time to walk down the road of nanotechnology. However, it is pertinent to say that both the developed and developing world’s scientific communities agree that nanotechnology will be the next step in technological generation [ 10 ]. This will make further industrial upgrading and investment in the field of nanotechnology indispensable in the coming years.
With advances in science and technology, the scientific community adopts technologies and products that are relatively cheap, safe, and cleaner than previous technologies. Moreover, they are concerned about the financial standing of technologies, as natural resources in the world are shrinking excessively [ 11 ]. Nanotechnology thus provides a gateway to this problem. This technology is clear, cleaner, and more affordable compared to previous mass bulking and heavy machinery. Moreover, nanotechnology holds the potential to be implemented in every aspect of life. This will mainly include nanomaterial sciences, nanoelectronics, and nanomedicine, being inculcated in all dimensions of chemistry and the physical and biological world [ 12 ]. Thus, it is not wrong to predict that nanotechnology will become a compulsory field of study for future generations [ 13 ]. This review inculcates the basic applications of nanotechnology in vital industries worldwide and their implications for future industrial progress [ 14 ].
2. Nanotechnology Applications
2.1. applications of nanotechnology in different industries.
After thorough and careful analyses, a wide range of industries—in which nanotechnology is producing remarkable applications—have been studied, reviewed, and selected to be made part of this review. It should be notified that multiple subcategories of industrial links may be discussed under one heading to elaborate upon the wide-scale applications of nanotechnology in different industries. A graphical abstract at the beginning of this article indicates the different industries in which nanotechnology is imparting remarkable implications, details of which are briefly discussed under different headings in the next session.
2.2. Nanotechnology and Computer Industry
Nanotechnology has taken its origins from microengineering concepts in physics and material sciences [ 15 ]. Nanoscaling is not a new concept in the computer industry, as technologists and technicians have been working for a long time to design such modified forms of computer-based technologies that require minimum space for the most efficient work. Resultantly, the usage of nanotubes instead of silicon chips is being increasingly experimented upon in computer devices. Feynman and Drexler’s work has greatly inspired computer scientists to design revolutionary nanocomputers from which wide-scale advantages could be attained [ 13 ]. A few years ago, it was an unimaginable to consider laptops, mobiles, and other handy gadgets as thin as we have today, and it is impossible for even the common man to think that with the passage of time, more advanced, sophisticated, and lighter computer devices will be commonly used. Nanotechnology holds the potential to make this possible [ 16 ].
Energy-efficient, sustainable, and urbanized technologies have been emerging since the beginning of the 21st century. The improvement via nanotechnology in information and communication technology (ICT) is noteworthy in terms of the improvements achieved in interconnected communities, economic competitiveness, environmental stability during demographic shifts, and global development [ 17 ]. The major implications of renewable technology incorporate the roles of ICT and nanotechnology as enablers of environmental sustainability. The traditional methods of product resizing, re-functioning, and enhanced computational capabilities, due to their expensiveness and complicated manufacturing traits, have slowly been replaced by nanotechnological renovations. Novel technologies such as smart sensors logic elements, nanochips, memory storage nanodevices, optoelectronics, quantum computing, and lab-on-a-chip technologies are important in this regard [ 18 ].
Both private and public spending are increasing in the field of nanocomputing. The growth of marketing and industrialization in the biotechnology and computer industries are running in parallel, and their expected growth rates for the coming years are far higher. Researchers and technologists believe that by linking the advanced field of nanotechnology and informatics and computational industries, various problems in human society such as basic need fulfillment can be easily accomplished in line with the establishment of sustainable goals by the end of this decade [ 19 ]. The fourth industrial revolution is based upon the supporting pillars derived from hyperphysical systems including artificial intelligence, machine learning, the internet of things, robots, drones, cloud computing, fast internet technologies (5G and 6G), 3D printing, and block chain technologies [ 20 ].
Most of these technologies have a set basis in computing, nanotechnology, biotechnology, material science renovations, and satellite technologies. Nanotechnology offers useful alterations in the physiochemical, mechanical, magnetic, electrical, and optical properties of computing materials which enable innovative and newer products [ 21 ]. Thus, nanotechnology is providing a pathway for another broad-spectrum revolution in the field of automotive, aerospace, renewable energy, information technology, bioinformatics, and environmental management, all of which have root origins from nanotechnological improvements in computers. Sensors involved in software and data algorithms employ nanomaterials to induce greater sensitivity and processabilities with minimal margin-to-machine errors [ 22 ]. Nanomaterials provide better characteristics and robustness to sensor technologies which mean they are chemically inert, corrosion-resistant, and have greater tolerance profiles toward temperature and alkalinity [ 22 ].
Moreover, the use of semiconductor nanomaterials in the field of quantum computing has increased overall processing speeds with better accuracy and transmissibility. These technologies offer the creation of different components and communication protocols at the nano level, which is often called the internet of nano things [ 23 ]. This area is still in a continuous development and improvement phase with the potential for telecommunication, industrial, and medical applications. This field has taken its origin from the internet of things, which is a hyperphysical world of sensors, software, and other related technologies which allow broad-scale communication via internet operating devices [ 17 ]. The applications of these technologies range from being on the simple home scale to being on the complex industrial scale. The internet of things is mainly capable of gathering and distributing large-scale data via internet-based equipment and modern gadgets. In short, the internet of nano things is applicable to software, hardware, and network connection which could be used for data manipulation, collection, and sharing across the globe [ 24 ].
Another application of nanotechnology in the computer and information industry comes in the form of artificial intelligence, machine learning, and big data platforms which have set the basis for the fourth industrial revolution. Vast amounts of raw data are collected through interconnected robotic devices, sensors, and machines which have properties of nanomaterials [ 18 ]. After wide-scale data gathering, the next step is the amalgamation of the internet of things and the internet of people to prepare a greater analysis, understanding, and utilization of the gathered information for human benefit [ 4 ]. Such data complications can be easily understood through the use of big data in the medical industry, in which epidemiological data provide benefits for disease management [ 2 ]. Yet another example is the applications in business, where sales and retail-related data help to elucidate the target markets, sales industry, and consumer behavioral inferences for greater market consumption patterns [ 19 ].
Similarly, an important dimension of nanotechnology and computer combination comes in the form of drone and robotics technology. These technologies have a rising number of applications in maintenance, inspections, transportation, deliverability, and data inspection [ 25 ]. Drones, robots, and the internet of things are being perfectly amalgamated with the industrial sector to achieve greater goals. Drones tend to be more mobile but rely more on human control as compared to robots, which are less mobile but have larger potential for self-operation [ 26 ]. However, now, more mobile drones with better autonomous profiles are being developed to help out in the domain of manufacturing industries. These devices intensify and increase the pace of automation and precision in industries along with providing the benefits of lower costs and fewer errors [ 24 ]. The integrated fields of robotics, the internet of things, and nanotechnology are often called the internet of robotics and nano things. This field of nanorobotics is increasing the flexibility and dexterity in manufacturing processes compared to traditional robotics [ 25 ].
Drones, on the contrary, help to manage tasks that are otherwise difficult or dangerous to be managed by humans, such as working from a far distance or in dangerous regions. Nanosensors help to equip drones with the qualities of improved detection and sensation more precisely than previous sensor technologies [ 21 , 27 ]. Moreover, the over-potential of working hours, battery, and maintenance have also been improved with the operationalization of nano-based sensors in drone technology. These drones are inclusively used for various purposes such as maintaining operations, employing safety profiling, security surveys, and mapping areas [ 18 ]. However, limitations such as high speed, legal and ethical limitations, safety concerns, and greater automobility are some of the drawbacks of aerial and robotic drone technologies [ 26 ].
Three-dimensional printing is yet another important application of the nanocomputer industry, in which an integrated modus operandi works to help in production management [ 28 ]. Nanotechnology-based 3D printing offers the benefits of an autonomous, integrated, intelligent exchange network of information which enables wide-scale production benefits. These technologies have enabled a lesser need for industrial infrastructure, minimized post-processing operations, reduced waste material generation, and reduced need for human presence for overall industrial management [ 28 , 29 ]. Moreover, the benefits of 3D printing and similar technologies have potentially increased flexibility in terms of customized items, minimal environmental impacts, and sustainable practices with lower resource and energy consumption. The use of nano-scale and processed resins, metallic raw material, and thermoplastics along with other raw materials allow for customized properties of 3D printing technology [ 29 ].
The application of nanotechnology in computers cannot be distinguished from other industrial applications, because everything in modern industries is controlled by a systemic network in association with a network of computers and similar technologies. Thus, the fields of electronics, manufacturing, processing, and packaging, among several others, are interlinked with nanocomputer science [ 11 , 15 ]. Silicon tubes have had immense applications that revolutionized the industrial revolution in the 20th century; now, the industrial revolution is in yet another revolutionary phase based on nanostructures [ 16 ]. Silicon tubes have been slowly replaced with nanotubes, which are allowing a great deal of improvement and efficiency in computing technology. Similarly, lab-on-a-chip technology and memory chips are being formulated at nano scales to lessen the storage space but increase the storage volume within a small, flexible, and easily workable chip in computers for their subsequent applications in multiple other industries.
Hundreds of nanotechnology computer-related products have been marketed in the last 20 years of the nanotechnological revolution [ 30 ]. Modern industries such as textiles, automotive, civil engineering, construction, solar technologies, environmental applications, medicine, transportation agriculture, and food processing, among others are largely reaping the benefits of nano-scale computer chips and other devices. In simple terms, everything out there in nanoindustrial applications has something to do with computer-based applications in the nanoindustry [ 31 , 32 , 33 ]. Thus, all the applications discussed in this review more or less originate from nanocomputers. These applications are enabling considerable improvement and positive reports within the industrial sector. Having said that, it is hoped that computer scientists will remain engaged and will keep on collaborating with scientists in other fields to further explore the opportunities associated with nanocomputer sciences.
2.3. Nanotechnology and Bioprocessing Industries
Scientific and engineering rigor is being carried out to the link fields of nanotechnology with contributions to the bioprocessing industry. Researchers are interested in how the basics of nanomaterials could be used for the high-quality manufacturing of food and other biomaterials [ 15 , 34 ]. Pathogenic identification, food monitoring, biosensor devices, and smart packaging materials, especially those that are reusable and biodegradable, and the nanoencapsulation of active food compounds are only a few nanotechnological applications which have been the prime focus of the research community in recent years. Eventually, societal acceptability and dealing with social, cultural, and ethical concerns will allow the successful delivery of nano-based bio-processed products into the common markets for public usage [ 20 , 35 ].
With the increasing population worldwide, food requirements are increasing in addition to the concerns regarding the production of safe, healthy, and recurring food options. Sensors and diagnostic devices will help improve the sensitivity in food quality monitoring [ 36 ]. Moreover, the fake industrial application of food products could be easily scanned out of a system with the application of nanotechnology which could control brand protection throughout bio-processing [ 6 ]. The power usage in food production might also be controlled after a total nanotechnological application in the food industry. The decrease in power consumption would ultimately be positive for the environment. This could directly bring in the interplay of environment, food, and nanotechnology and would help to reduce environmental concerns in future [ 37 ].
One of the important implications of nanotechnology in bioprocessing industries can be accustomed to fermentation processes; these technologies are under usage for greater industrial demand and improved biomolecule production at a very low cost, unlike traditional fermentation processes [ 35 ]. The successful implementation and integration of fermentation and nanotechnology have allowed the development of biocompatible, safe, and nontoxic substances and nanostructures with wide-scale application in the field of food, bioprocessing, and winemaking industries [ 38 ]. Another important application is in the food monitoring and food supply chain management, present in various subsectors such as production, storage, distribution, and toxicity management. Nanodevices and nanomaterials are incorporated into chemical and biological sensor technologies to improve overall analytical performance with regard to parameters such as response time, sensitivity, selectivity, accuracy, and reliability [ 39 ]. The conventional methods of food monitoring are slowly being replaced with modern nano-based materials such as nanowires, nanocomposites, nanotubes, nanorods, nanosheets, and other materials that function to immobilize and label components [ 40 ]. These methods are either electrochemically or optically managed. For food monitoring, several assays are proposed and implemented with their roots in nano-based technologies; they may include molecular and diagnostic assays, immunological assays, and electrochemical and optical assays such as surface-enhanced Raman scattering and colorimetry technologies [ 34 ]. Materials ranging from heavy materials to microorganisms, pesticides, allergens, and antibiotics are easily monitored during commercial processing and bioprocessing in industries.
Additionally, nanotechnology has presented marvelous transformations in bio-composting materials. With the rising demand for biodegradable composites worldwide to reduce the environmental impact and increase the efficiency of industrial output, there is an increasing need for sustainable technologies [ 41 ]. Nanocomposites are thus being formulated with valuable mechanical properties better than conventional polymers, thus establishing their applicability in industries. The improved properties include optical, mechanical, catalytic, electrochemical, and electrical ones [ 42 ]. These biodegradable polymers are not only used in bioprocessing industries to create food products with relevant benefits but are also being deployed in the biomedical field, therapeutic industries, biotechnology base tissue engineering field, packing, sensor industries, drug delivery technology, water remediation, food industries, and cosmetics industries as well [ 2 , 24 , 34 , 43 ]. These nanocomposites have outstanding characteristics of biocompatibility, lower toxicities, antimicrobial activity, thermal resistance, and overall improved biodegradation properties which make them worthy of applications in products [ 44 ]. However, it is still imperative to conduct wide-scale toxicity and safety profiling for these and other nanomaterials to ensure the safety requirements, customer satisfaction, and public benefit are met [ 44 ].
Moreover, the advancement of nanotechnology has also been conferred to the development of functional food items. The exposure and integration of nanotechnology and the food industry have resulted in larger quantities of sustainable, safer, and healthier food products for human consumption, which is a growing need for the rising population worldwide [ 45 ]. The overall positive impact of nanotechnology in food processing, manufacturing, packing, pathogenic detection, monitoring, and production profiles necessitates the wide-scale application of this technology in the food industry worldwide [ 4 , 41 ]. Recent research has shown how the delivery of bioactive compounds and essential ingredients is and can be improved by the application of nanomaterials (nanoencapsulation) in food products [ 46 ]. These technologies improve the protection performance and sensitivity of bioactive ingredients while preventing unnecessary interaction with other constituents of foods, thus establishing clear-cut improved bioactivity and solubility profiles of nanofoods, thereby improving human health benefits. However, it should be kept in mind that the safety regards of these food should be carefully regulated with safety profiling, as they directly interact with human bodies [ 47 ].
2.4. Nanotechnology and Agri-Industries
Agriculture is the backbone of the economies of various nations around the globe. It is a major contributing factor to the world economy in general and plays a critical role in population maintenance by providing nutritional needs to them. As global weather patterns are changing owing to the dramatic changes caused by global warming, it is accepted that agriculture will be greatly affected [ 48 ]. Under this scenario, it is always better to take proactive measures to make agricultural practices more secure and sustainable than before. Modern technology is thus being employed worldwide. Nanotechnology has also come to play an effective role in this interplay of sustainable technologies. It plays an important role during the production, processing, storing, packaging, and transport of agricultural industrial products [ 49 ].
Nanotechnology has introduced certain precision farming techniques to enhance plant nutrients’ absorbance, alongside better pathogenic detection against agricultural diseases. Fertilizers are being improved by the application of nanoclays and zeolites which play effective roles in soil nutrient broths and in the restoration soil fertility [ 49 ]. Modern concepts of smart seeds and seed banks are also programmed to germinate under favorable conditions for their survival; nanopolymeric mixtures are used for coating in these scenarios [ 50 ]. Herbicides, pesticides, fungicides, and insecticides are also being revolutionized through nanotechnology applications. It has also been considered to upgrade linked fields of poultry and animal husbandry via the application of nanotechnology in treatment and disinfection practices.
2.5. Nanotechnology and Food Industry
The applications of nanotechnology in the food industry are immense and include food manufacturing, packaging, safety measures, drug delivery to specific sites [ 51 ], smart diets, and other modern preservatives, as summarized in Figure 1 . Nanomaterials such as polymer/clay nanocomposites are used in packing materials due to their high barrier properties against environmental impacts [ 52 ]. Similarly, nanoparticle mixtures are used as antimicrobial agents to protect stored food products against rapid microbial decay, especially in canned products. Similarly, several nanosensor and nano-assembly-based assays are used for microbial detection processes in food storage and manufacturing industries [ 53 ].

Nanotechnology applications in food and interconnected industries.
Nanoassemblies hold the potential to detect small gasses and organic and inorganic residues alongside microscopic pathogenic entities [ 54 ]. It should, however, be kept in mind that most of these nanoparticles are not directly added to food species because of the risk of toxicity that may be attached to such metallic nanoparticles. Work is being carried out to predict the toxicity attached, so that in the future, these products’ market acceptability could be increased [ 55 ]. With this, it is pertinent to say that nanotechnology is rapidly taking steps into the food industry for packing, sensing, storage, and antimicrobial applications [ 56 ].
Nanotechnology is also revolutionizing the dairy industry worldwide [ 57 ]. An outline of potential applications of nanotechnology in the dairy industry may include: improved processing methods, improved food contact and mixing, better yields, the increased shelf life and safety of dairy-based products, improved packaging, and antimicrobial resistance [ 58 ]. Additionally, nanocarriers are increasingly applied to transfer biologically active substances, drugs, enhanced flavors, colors, odors, and other food characteristics to dairy products [ 59 ].
These compounds exhibit higher delivery, solubility, and absorption properties to their targeted system. However, the problem of public acceptability due to the fear of unknown or potential side effects associated with nano-based dairy and food products needs to be addressed for the wider-scale commercialization of these products [ 60 ].
2.5.1. Nanotechnology, Poultry and Meat Industry
The poultry industry is a big chunk of the food industry and contributes millions of dollars every year to food industries around the world. Various commercial food chains are running throughout the world, the bases of which start from healthy poultry industries. The incidence of widespread foodborne diseases that originate from poultry, milk, and meat farms is a great concern for the food industry. Nanobiotechnology is certainly playing a productive role in tackling food pathogens such as those which procreate from Salmonella and Campylobacter infections by allowing increased poultry consumption while maintaining the affordability and safety of manufactured chicken products [ 61 ]. Several nano-based tools and materials such as nano-enabled disinfectants, surface biocides, protective clothing, air and water filters, packaging materials, biosensors, and detective devices are being used to confirm the authenticity and traceability of poultry products [ 62 ]. Moreover, nano-based materials are used to reduce foodborne pathogens and spoilage organisms before the food becomes part of the supply chain [ 63 ].
2.5.2. Nanotechnology—Fruit and Vegetable Industry
As already described, nanotechnology has made its way far ahead in the food industry. The agricultural, medicinal, and fruit and vegetable industries cannot remain unaffected under this scenario. Scientists are trying to increase the shelf life of fresh organic products to fulfill the nutritional needs of a growing population. From horticulture to food processing, packaging, and pathogenic detection technology, nanotechnology plays a vital role in the safety and production of vegetables and fruits [ 64 ].
Conventional technologies are now being replaced with nanotechnology due to their benefits of cost-effectiveness, satisfactory results, and overall shelf life improvement compared to past practices. Although some risks may be attached, nanotechnology has not yet reported high-grade toxicity to organic fresh green products. These technologies serve the purpose of providing safe and sufficient food sources to customers while reducing postharvest wastage, which is a major concern in developing nations [ 55 ]. Nanopackaging provides the benefits of lower humidity, oxygen passage, and optimal water vapor transmission rates. Hence, in the longer run, the shelf life of such products is increased to the desired level using nanotechnology [ 65 ].
2.5.3. Nanotechnology and Winemaking Industry
The winemaking industry is a big commercial application of the food industry worldwide. The usage of nanotechnology is also expanding in this industry. Nanotechnology serves the purpose of sensing technology through employment as nanoelectronics, nanoelectrochemical, and biological, amperometric, or fluorimetric sensors. These nanomaterials help to analyze the wine components, including polyphenols, organic acids, biogenic amines, or sulfur dioxide, and ensure they are at appropriate levels during the production of wine and complete processing [ 66 ].
Efforts are being made to further improve sensing nanotechnology to increase the accuracy, selectivity, sensitivity, and rapid response rate for wine sampling, production, and treatment procedures [ 53 ]. Specific nanoassemblies that are used in winemaking industries include carbon nanorods, nanodots, nanotubes, and metallic nanoparticles such as gold, silver, zinc oxide, iron oxide, and other types of nanocomposites. Recent research studies have introduced the concept of electronic tongues, nanoliquid chromatography, mesoporous silica, and applications of magnetic nanoparticles in winemaking products [ 67 ]. An elaborative account of these nanomaterials is out of the scope of the present study; however, on a broader scale, it is not wrong to say that nanotechnology is successfully reaping in the field of enology.
2.6. Nanotechnology and Packaging Industries
The packaging industry is continuously under improvement since the issue of environmentalism has been raised around the globe. Several different concerns are linked to the packaging industry; primarily, packaging should provide food safety to deliver the best quality to the consumer end. In addition, packaging needs to be environmentally friendly to reduce the food-waste-related pollution concern and to make the industrial processes more sustainable. Trials are being carried out to reduce the burden by replacing non-biodegradable plastic packaging materials with eco-friendly organic biopolymer-based materials which are processed at the nano scale to incur the beneficial properties of nanotechnology [ 68 ].
The nanomanufacturing of packaging biomaterials has proven effective in food packaging industries, as nanomanufacturing not only contributes to increasing food safety and production but also tackles environmental issues [ 69 ]. Some examples of these packaging nanomaterials may include anticaking agents, nanoadditives, delivery systems for nutraceuticals, and many more. The nanocompositions of packing materials are formed by mixing nanofillers and biopolymers to enhance packaging’s functionality [ 70 ]. Nanomaterials with antimicrobial properties are preferred in these cases, and they are mixed with a polymer to prevent the contamination of the packaged material. It is important to mention here that this technology is not only limited to food packaging; instead, packaging nanotechnology is now also being introduced in certain other industries such as textile, leather, and cosmetic industries in which it is providing large benefits to those industries [ 64 ].
2.7. Nanotechnology and Construction Industry and Civil Engineering
Efficient construction is the new normal application for sustainable development. The incorporation of nanomaterials in the construction industry is increasing to further the sustainability concern [ 71 ]. Nanomaterials are added to act as binding agents in cement. These nanoparticles enhance the chemical and physical properties of strength, durability, and workability for the long-lasting potential of the construction industry. Materials such as silicon dioxide which were previously also in use are now manufactured at the nano scale [ 71 ]. These nanostructures along with polymeric additives increase the density and stability of construction suspension [ 72 ]. The aspect of sustainable development is being applied to the manufacture of modern technologies coupled with beneficial applications of nanotechnology. This concept has produced novel isolative and smart window technologies which have driven roots in nanoengineering, such as vacuum insulation panels (VIPs) and phase change materials (PCMs), which provide thermal insulation effects and thus save energy and improve indoor air quality in homes [ 73 ].
A few of the unique properties of nanomaterials in construction include light structure, strengthened structural composition, low maintenance requirements, resistant coatings, improved pipe and bridge joining materials, improved cementitious materials, extensive fire resistance, sound absorption, and insulation properties, as well as the enhanced reflectivity of glass surfaces [ 74 ]. As elaborated under the heading of civil engineering applications, concrete’s properties are the most commonly discussed and widely changing in the construction industry because of concrete’s minute structure, which can be easily converted to the nano scale [ 75 ]. More specifically, the combination of nano-SiO 2 in cement could improve its performance in terms of compressiveness, large volumes with increased compressiveness, improved pore size distribution, and texture strength [ 76 ].
Moreover, some studies are also being carried out to improve the cracking properties of concrete by the application of microencapsulated healing polymers, which reduce the cracking properties of cement [ 77 ]. Moreover, some other construction materials, such as steel, are undergoing research to change their structural composites through nano-scale manufacturing. This nanoscaling improves steel’s properties such as improved corrosion resistance, increased weldability, the ease of handling for designing building materials, and construction work [ 78 ]. Additionally, coating materials have been improved by being manufactured at the nano scale. This has led to different improved coating properties such as functional improvement; anticorrosive action; high-temperature, fire, scratch, and abrasion resistance; antibacterial and antifouling self-healing capabilities; and self-assembly, among other useful applications [ 79 ].
Nanotechnology improves the compressive flexural properties of cement and reduces its porosity, making it absorb less water compared to traditional cementation preparations. This is because of the high surface-to-volume ratio of nanosized particles. Such an approach helps in reducing the amount of cement in concrete, making it more cost-effective, more strengthening, and eco-friendly, known as ‘green concrete’. Besides concrete, the revolutionary characteristics of nanotechnology are now also being adopted in other construction materials such as steel, glass, paper, wood, and multiple other engineering materials to upgrade the construction industry [ 80 ].
Similarly, carbon nanotubes, nanorods, and nanofibers are rapidly replacing steel constructions. These nanostructures along with nanoclay formations increase the mechanical properties and thus have paved the way for a new branch of civil engineering in terms of nanoengineering [ 80 ]. Apart from cement formulations, nanoparticles are included in repair mortars and concrete with healing properties that help in crack recovery in buildings. Furthermore, nanostructures, titanium dioxide, zinc, and other metallic oxides are being employed for the production of photocatalytic products with antipathogenic, self-cleaning, and water- and germ-repellent built-in technologies [ 33 ]. Similarly, quantum dot technologies are progressively employed for solar energy generation (a concept discussed later). These photovoltaic cells contribute to saving the maximum amount of solar energy [ 81 ].
2.8. Nanotechnology and Textiles Industry
The textile industry achieved glory in the 21st century with enormous outgrowth through social media platforms. Large brands have taken over the market worldwide, and millions are earned every year through textile industries. With the passing of time, nanotechnology is being slowly incorporated into the textile fiber industry owing to its unique and valuable properties. Previously, fabrics manufactured via conventional methods often curtailed the temporary effects of durability and quality [ 82 ]. However, the age of nanotechnology has allowed these fabric industries to employ nanotechnology to provide high durability, flexibility, and quality to clothes which is not lost upon laundering and wearing. The high surface-to-volume ratio of nanomaterials keeps high surface energy and thus provides better affinity to their fabrics, leading to long-term durability [ 82 ]. Moreover, a thin layering and coating of nanoparticles on the fabric make them breathable and make them smooth to the touch. This layering is carried out by processes such as printing, washing, padding, rinsing, drying, and curing to attach nanoparticles on the fabric surface. These processes are carried out to impart the properties of water repellence, soil resistance, flame resistance, hydrophobicity, wrinkle resistance, antibacterial and antistatic properties, and increased dyeability to the clothes [ 83 ].
The unique properties of nanomaterials in textile industries have attracted large-scale businesses for the financial benefits attached to their application. For this reason, competitors are increasing in nanotextile industry speedily, which may make the conventional textile industry sidelined in the near future [ 84 ]. Some benefits associated with nanotextile engineering and industry may include: improved cleaning surfaces, soil, wrinkle, stain, and color damage resistance, higher wettability and strike-through characteristics, malodor- and soil-removal abilities, abrasion resistance, a modified version of surface friction, and color enhancement through nanomaterials [ 85 ].
These characteristics have hugely improved the functionality and performance characteristics of textile and fiber materials [ 86 ]. Based upon the numerous advantages, nanotextile technology is increasingly being used in various inter-related fields, including in medical clothes, geotextiles, shock-resistant textiles, and fire-resistant and water-resistant textiles [ 87 ]. These textiles and fibers help overcome severe environmental conditions in special industries where high temperatures, pressure, and other conditions are adjusted for manufacturing purposes. These textiles are now increasingly called smart clothes due to renewed nanotechnological application to traditional methods [ 88 ].
The increasing demand for durable, appealing, and functionally outstanding textile products with a couple of factors of sustainability has allowed science to incorporate nanotechnology in the textile sector. These nano-based materials offer textile properties such as stain-repellent, wrinkle-free textures and fibers’ electrical conductivity alongside guaranteeing comfort and flexibility in clothing [ 82 ]. The characteristics of nanomaterials are also exhibited in the form of connected garments creation that undergo sensations to respond to external stimuli through electrical, colorant, or physiological signals. Thus, a kind of interconnection develops between the fields of photonic, electrical, textile and nanotechnologies [ 89 ]. Their interconnected applications confer the properties of high-scale performance, lasting durability, and connectivity in textile fibers. However, the concerns of nanotoxicity, the chances of the release of nanomaterials during washing, and the overall environmental impact of nanotextiles are important challenges that need to be ascertained and dealt with successfully in the coming years to ensure wide-scale acceptance and the global broad-spectrum application of nanotextiles [ 90 ].
The global market for the textile industry is constantly on the rise; with so many new brands, the competition is rising in regard to pricing, material, product outlook, and market exposure. Under this scenario, nanotechnology has contributed in terms of value addition to textiles by contributing the properties of water repellence, self-cleaning, and protection from radiation and UV light, along with safety against flames and microorganisms [ 82 ]. A whole new market of smart clothes is slowly taking our international markets along with improvements in textile machinery and economic standing. These advances have effectively established the sustainable character of the textile industry and have created grounds to meet the customer’s demand [ 91 ]. Some important examples of smart clothing originating from the nanotextile industry can be seen in products such as bulletproof jackets, fabric coatings, and advanced nanofibers. Fabric coatings and pressure pads can exhibit characteristics of invisibility and entail a silver, nickel, or gold nanoparticle-based material with inherent antimicrobial properties [ 92 ]. Such materials are effectively being utilized and introduced into the medical industry for bandages, dressings, etc. [ 92 ].
Similarly, woven optical fibers are already making progress in the textile and IT industry. With the incorporation of nanomaterials, optical fibers are being utilized for a range of purposes such as light transmission, sensing technologies, deformation, improved formational characteristic detection, and long-range data transmission. These optical fibers with phase-changing material properties can also be utilized for thermostability maintenance in the fiber industry. Thus, these fibers have combined applications in the computer, IT, and textile sectors [ 93 ]. In addition, the nano cellulosic material that is naturally obtained from plants confers properties of stiffness, strength, durability, and large surface area to volume ratios, which is acquired through the large number of surface hydroxyl groups embedded in nanocellulose particles [ 94 ]. Moreover, the characteristics of high resistance, lower weight, cost-effectiveness, and electrical conductivity are some additional benefits which are also linked to these nanocellulosic fibers [ 93 ]. The aforementioned technologies will allow industrialists to manufacture fabrics based on nanomaterials through a variety of chemical, physical, and biological processes. The scope of improvement in the textile properties, cost, and production methods is making the nanotextile industry a strong field of interest for future industrial investments.
2.9. Nanotechnology and Transport and Automobile Industry
The automotive industry is always improving its production. Nanotechnology is one such tool that could impart the automotive industry with a totally new approach to manufacturing. Automobile shaping could be improved greatly without any changes to the raw materials used. The replacement of conventional fabrication procedures with advanced nanomanufacturing is required to achieve the required outcome. Nanotechnology intends to partly renovate the automobile industry by enhancing the technical performance and reducing production costs excessively. However, there is a gap in fully harnessing the potential of nanomaterials in the automotive industry. Industrialists who were previously strict about automotive industrial principles are ready to employ novelties attached to nanotechnology to create successful applications to automobiles in the future [ 95 ]. Nanotechnology could provide assistance in manufacturing methods with an impartment of extended life properties. Cars that have been manufactured with nanotechnology applications have shown lower failure rates and enhanced self-repairing properties. Although the initial investment in the nanoautomated industry is high, the outcomes are enormous.
The concept of sustainable transport could also be applied to the manufacturing of such nano-based technology which is CO 2 free and imparts safe driving and quiet, clean, and wider-screen cars, which, in the future, may be called nanocars. The major interplay of nanotechnology and the automotive industry comes in the manufacturing of car parts, engines, paints, coating materials, suspensions, breaks, lubrication, and exhaust systems [ 32 ]. These properties are largely imparted via carbon nanotubes and carbon black, which renders new functionalities to automobiles. These products were previously in use, but nanoscaling and nanocoating allow for enhanced environmental, thermal, and mechanical stability to be imparted to the new generation of automobiles. In simple terms, automobiles manufactured with principal nanonovelties could result in cars with less wearing risk, better gliding potential, thinner coating lubrication requirements, and long service bodies with weight reductions [ 31 ]. These properties will ultimately reduce costs and will impart more space for improved automobile manufacturing in the future. Similarly, the development of electric cars and cars built on super capacitor technology is increasingly based on nanotechnology. The implications of nanotechnology in the form of rubber fillers, body frames made of light alloys, nanoelectronic components, nanocoatings of the interior and exterior of cars, self-repairing materials against external pressure, nanotextiles for interiors, and nanosensors are some of the nanotechnological-based implications of the automotive industry [ 96 ]. Owing to these properties, nanotechnology ventures are rapidly progressing in the automobile industry. It is expected that, soon, the automobile industry will commercialize nanotechnological perspectives on their branding strategies.
2.10. Nanotechnology, Healthcare, and Medical Industry
The genesis of nanomedicine simply cannot be ignored when we talk about the large fields of biological sciences, biotechnology, and medicine. Nanotechnology is already making its way beyond the imagination in the broader vision of nanobiotechnology. The quality of human life is continuously improved by the successful applications of nanotechnology in medicine, and resultantly, the entire new field of nanomedicine has come to the surface, which has allowed scientists to create upgraded versions of diagnostics, treatment, screening, sequencing, disease prevention, and proactive actions for healthcare [ 97 ]. These practices may also involve drug manufacturing, designing, conjugation, and efficient delivery options with advances in nano-based genomics, tissue engineering, and gene therapy. With this, it could be predicted that soon, nanomedicine will be the foremost research interest for the coming generation of biologists to study the useful impacts and risks that might be associated with them [ 98 ]. As illustrated in Figure 2 , we summarized the applications of nanotechnology in different subfields of the medical industry.

Nanotechnology applications in medical industry. Nanotechnology has a broad range of applications in various diagnostics and treatments using nanorobotics and drug delivery systems.
In various medical procedures, scientists are exploring the potential benefits of nanotechnology. In the field of medical tools, various robotic characters have been applied which have their origins in nano-scale computers, such as diagnostic surfaces, sensor technologies, and sample purification kits [ 99 ]. Similarly, some modifications are being accepted in diagnostics with the development of devices that are capable of working, responding, and modifying within the human body with the sole purpose of early diagnosis and treatment. Regenerative medicine has led to nanomanufacturing applications in addition to cell therapy and tissue engineering [ 100 ]. Similarly, some latest technologies in the form of ‘lab-on-a-chip’, as elaborated upon earlier, are being introduced with large implications in different fields such as nanomedicine, diagnostics, dentistry, and cosmetics industries [ 101 ]. Some updated nanotechnology applications in genomics and proteomics fields have developed molecular insights into antimicrobial diseases. Moreover, medicine, programming, nanoengineering, and biotechnology are being merged to create applications such as surgical nanorobotics, nanobioelectrics, and drug delivery methods [ 102 ]. All of these together help scientists and clinicians to better understand the pathophysiology of diseases and to bring about better treatment solutions in the future.
Specifically, the field of nanocomputers and linked devices help to control activation responses and their rates in mechanical procedures [ 2 ]. Through these mechanical devices, specific actions of medical and dental procedures are executed accurately. Moreover, programmed nanomachines and nanorobots allow medical practitioners to carry out medical procedures precisely at even sub-cellular levels [ 4 ]. In diagnostics fields, the use of such nanodevices is expanding rapidly, which allows predictions to be made about disease etiology and helps to regulate treatment options [ 103 ]. The use of in vitro diagnosis allows increased efficiency in disease apprehension. Meanwhile, in in vivo diagnoses, such devices have been made which carry out the screening of diseased states and respond to any kind of toxicities or carcinogenic or pathological irregularities that the body faces [ 104 ].
Similarly, the field of regenerative medicine is employing nanomaterials in various medical procedures such as cell therapy, tissue engineering, and gene sequencing for the greater outlook of treatment and reparation of cells, tissues, and organs. Nanoassemblies have been recorded in research for applications in powerful tissue regeneration technologies with properties of cell adhesion, migration, and cellular differentiation [ 102 ]. Additionally, nanotechnology is being applied in antimicrobial (antibacterial and antiviral) fields. The microscopic abilities of these pathogens are determined through nano-scale technologies [ 100 ]. Greek medicinal practices have long been using metals to cure pathogenic diseases, but the field of nanotechnology has presented a new method to improve such traditional medical practices; for example, nanosized silver nanomaterials are being used to cure burn wounds owing to the easy penetration of nanomaterials at the cellular level [ 102 , 105 ].
In the field of bioinformatics and computational biology, genomic and proteomic technologies are elucidating molecular insights into disease management [ 106 ]. The scope of targeted and personalized therapies related to pathogenic and pathophysiological diseases have greatly provided spaces for nanotechnological innovative technologies [ 107 , 108 ]. They also incorporate the benefits of cost-effectiveness and time saving [ 109 ]. Similarly, nanosensors and nanomicrobivores are utilized for military purposes such as the detection of airborne chemical agents which could cause serious toxic outcomes otherwise [ 102 ]. Some nanosensors also serve a purpose similar to phagocytes to clear toxic pathogens from the bloodstream without causing septic shock conditions, especially due to the inhalation of prohibited drugs and banned substances [ 100 , 105 , 110 ]. These technologies are also used for dose specifications and to neutralize overdosing incidences [ 110 ] Nano-scale molecules work as anticancer and antiviral nucleoside analogs with or without other adjuvants [ 21 ].
Another application of nanotechnology in the medical industry is in bone regeneration technology. Scientists are working on bone graft technology for bone reformation and muscular re-structuring [ 111 , 112 ]. Principle investigations of biomineralization, collagen mimic coatings, collagen fibers, and artificial muscles and joints are being conducted to revolutionize the field of osteology and bone tissue engineering [ 113 , 114 ]. Similarly, drug delivery technologies are excessively considering nanoscaling options to improve drug delivery stability and pharmacodynamic and pharmacokinetic profiles at a large scale [ 110 ]. The use of nanorobots is an important step that allows drugs to travel across the circulatory system and deliver drug entities to specifically targeted sites [ 99 , 115 ]. Scientists are even working on nanorobots-based wireless intracellular and intra-nucleolar nano-scale surgeries for multiple malignancies, which otherwise remain incurable [ 102 ]. These nanorobotics can work at such a minute level that they can even cut a single neuronic dendrite without causing harm to complex neuronal networks [ 116 ].
Another important application of nanotechnology in the medical field is oncology. Nanotechnology is providing a good opportunity for researchers to develop such nanoagents, fluorescent materials, molecular diagnostics kits, and specific targeted drugs that may help to diagnose and cure carcinogenesis [ 104 ]. Scientists are trying various protocols of adjoining already-available drugs with nanoparticulate conjugation to enhance drug specificity and targeting in organs [ 104 , 107 , 117 ]. Nanomedicine acts as the carrier of hundreds of specific anticancerous molecules that could be projected at tumor sites; moreover, the tumor imaging and immunotherapy approaches linked with nanomedicine are also a potential field of interest when it comes to cancer treatment management [ 112 , 117 ]. A focus is also being drawn toward lessening the impact of chemotherapeutic drugs by increasing their tumor-targeting efficiency and improving their pharmacokinetic and pharmacodynamic properties [ 112 ]. Similarly, heat-induced ablation treatment against cancer cells alongside gene therapy protocols is also being coupled with nanorobotics [ 99 , 118 ]. Anticancerous drugs may utilize the Enhanced Permeation and Retention Effect (EPR effect) by applications of nano assemblies such as liposomes, albumin nanospheres, micelles, and gold nanoparticles, which confirms effective treatment strategies against cancer [ 119 ]. Such advances in nanomedicine will bring about a more calculated, outlined, and technically programmed field of nanomedicine through association and cooperation between physicians, clinicians, researchers, and technologies.
2.10.1. Nanoindustry and Dentistry
Nanodentistry is yet another subfield of nanomedicine that involves broad-scale applications of nanotechnology ranging from diagnosis, prevention, cure, prognosis, and treatment options for dental care [ 120 ]. Some important applications in oral nanotechnology include dentition denaturalization, hypersensitivity cure, orthodontic realignment problems, and modernized enameling options for the maintenance of oral health [ 2 , 121 ]. Similarly, mechanical dentifrobots work to sensitize nerve impulse traffic at the core of a tooth in real-time calculation and hence could regulate tooth tissue penetration and maintenance for normal functioning [ 122 ]. The functioning is coupled with programmed nanocomputers to execute an action from external stimuli via connection with localized internal nerve stimuli. Similarly, there are other broad-range applications of nanotechnology in tooth repair, hypersensitivity treatment, tooth repositioning, and denaturalization technologies [ 4 , 118 , 120 , 121 ]. Some of the applications of nanotechnology in the field of dentistry are elaborated upon in Figure 3 .

Nanotechnology applications in field of dentistry. Nanotechnology can be largely used in dentistry to repair and treat dental issues.
2.10.2. Nanotechnology and Cosmetics Industry
The cosmetics industry, as part of the greater healthcare industry, is continuously evolving. Nanotechnology-based renovations are progressively incorporated into cosmetics industries as well. Products are designed with novel formulations, therapeutic benefits, and aesthetic output [ 123 ]. The nanocosmetics industry employs the usage of lipid nanocarrier systems, polymeric or metallic nanoparticles, nanocapsules, nanosponges, nanoemulsions, nanogels, liposomes, aquasomes, niosomes, dendrimers, and fullerenes, etc., among other such nanoparticles [ 101 ]. These nanomaterials bring about specific characteristics such as drug delivery, enhanced absorption, improved esthetic value, and enhanced shelf life. The benefits of nanotechnology are greatly captured in the improvement of skin, hair, nail, lip, and dental care products, and those associated with hygienic concerns. Changes to the skin barrier have been largely curtailed owing to the function of the nano scale of materials. The nanosize of active ingredients allows them to easily permeate skin barriers and generate the required dermal effect [ 124 ].
More profoundly, nanomaterials’ application is encouraged in the production of sun-protective cosmetics products such as sunblock lotions and creams. The main ingredient used is the rational combination of cinnamates (derived from carnauba wax) and titanium dioxide nanosuspensions which provide sun-protective effects in cosmetics products [ 125 ]. Similarly, nanoparticle suspensions are being applied in nanostructured lipid carriers (NLCs) for dermal and pharmaceutical applications [ 126 ]. They exhibit the properties of controlled drug-carrying and realizing properties, along with direct drug targeting, occlusion, and increased penetration and absorption to the skin surface. Moreover, these carrier nanoemulsions exhibit excellent tolerability to intense environmental and body conditions [ 127 ]. Moreover, these lipid nanocarriers have been researched and declared safe for potential cosmetic and pharmaceutical applications. However, more research is still required to assess the risk/benefit ratio of their excessive application [ 128 ].
2.11. Nanotechnology Industries and Environment
The environment, society, and technology are becoming excessively linked under a common slogan of sustainable development. Nanotechnology plays a key role in the 21st century to modify the technical and experimental outlook of various industries. Environmental applications cannot stand still against revolutionary applications of nanotechnology. Since the environment has much to do with the physical and chemical world around a living being, the nano scale of products greatly changes and affects environmental sustainability [ 129 ]. The subsequent introduction of nanomaterials in chemistry, physics, biotechnology, computer science, and space, food, and chemical industries, in general, directly impacts environmental sciences.
With regard to environmental applications, the remarkable research and applications of nanotechnology are increasing in the processing of raw materials, product manufacturing, contaminate treatment, soil and wastewater treatment, energy storage, and hazardous waste management [ 130 ]. In developed nations, it is now widely suggested that nanotechnology could play an effective role in tackling environmental issues. In fact, the application of nanotechnology could be implemented for water and cell cleaning technologies, drinking safety measures, and the detoxification of contaminants and pollutants from the environment such as heavy metals, organochlorine pesticides, and solvents, etc., which may involve reprocessing although nanofiltration. Moreover, the efficiency and durability of materials can be increased with mechanical stress and weathering phenomena. Similarly, the use of nanocage-based emulsions is being used for optical imaging techniques [ 131 ].
In short, the literature provides immense relevance to how nanotechnology is proving itself through groundbreaking innovative technologies in environmental sciences. The focus, for now, is kept on remediation technologies with prime attention on water treatment, since water scarcity is being faced worldwide and is becoming critical with time. There is a need for the scientific community to actively conduct research on comprehending the properties of nanomaterials for their high surface area, related chemical properties, high mobility, and unique mechanical and magnetic properties which could be used for to achieve a sustainable environment [ 132 ].
2.12. Nanotechnology—Oil and Gas Industry
The oil and gas industry makes up a big part of the fossil industry, which is slowly depleting with the rising consumption. Although nanotechnology has been successfully applied to the fields of construction, medicine, and computer science, its application in the oil and gas industry is still limited, especially in exploration and production technologies [ 133 ]. The major issue in this industry is to improve oil recovery and the further exploitation of alternative energy sources. This is because the cost of oil production and further purification is immense compared to crude oil prices. Nanotechnologists believe that they could overcome the technological barriers to developing such nanomaterials that would help in curtailing these problems.
Governments are putting millions of dollars into the exploration, drilling, production, refining, wastewater treatment, and transport of crude oil and gas. Nanotechnology can provide assistance in the precise measurement of reservoir conditions. Similarly, nanofluids have been proven to exhibit better performance in oil production industries. Nanocatalyses enhance the separation processing of oil, water, and gases, thus bringing an efficient impurity removal process to the oil and gas industry. Nanofabrication and nanomembrane technologies are excessively being utilized for the separation and purification of fossil materials [ 134 ]. Finally, functional and modified nanomaterials can produce smart, cost-effective, and durable equipment for the processing and manufacturing of oil and gas. In short, there is immense ground for the improvement of the fossil fuel industry if nanotechnology could be correctly directed in this industry [ 135 ].
2.13. Nanotechnology and Renewable Energy (Solar) Industry
Renewable energy sources are the solutions to many environmental problems in today’s world. This makes the renewable energy industry a major part of the environmental industry. Subsequently, nanotechnology needs to be considered in the energy affairs of the world. Nanotechnologies are increasingly applied in solar, hydrogen, biomass, geothermal, and tidal wave energy production. Although, scientists are convinced that much more needs to be discovered before enhancing the benefits of coupled nanotechnology and renewable energy [ 136 ].
Nanotechnology has procured its application way down the road of renewable energy sources. Solar collectors have been specifically given much importance since their usage is encouraged throughout the world, and with events of intense solar radiation, the production and dependence of solar energy will be helpful for fulfilling future energy needs. Research data are available regarding the theoretical, numerical, and experimental approaches adopted for upgrading solar collectors with the employment of nanotechnologies [ 137 ].
These applications include the nanoengineering of flat solar plates, direct absorption plates, parabolic troughs, and wavy plates and heat pipes. In most of these instruments and solar collection devices, the use of nanofluids is becoming common and plays a crucial role in increasing the working efficiency of these devices. A gap, however, exists concerning the usage of nanomaterials in the useful manufacturing design of solar panels and their associated possible efficiencies which could be brought to the solar panel industry. Moreover, work needs to be done regarding the cost-effectiveness and efficiency analyses of traditional and nanotechnology-based solar devices so that appropriate measures could be adopted for the future generation of nanosolar collectors [ 138 ].
2.14. Nanotechnology and Wood Industry
The wood industry is one of the main economic drivers in various countries where forest growth is immense and heavy industrial setups rely on manufacturing and selling wood-based products [ 139 ]. However, the rising environmental concerns against deforestation are a major cause for researchers to think about a method for the sustainable usage of wood products. Hence, nanotechnology has set its foot in the wood industry in various applications such as the production of biodegradable materials in the paper and pulp industry, timber and furniture industry, wood preservatives, wood composites, and applications in lignocellulosic-based materials [ 140 ]. Resultantly, new products are introduced into the market with enhanced performance (stronger yet lighter products), increased economic potential, and reduced environmental impact.
One method of nano-based application in the wood industry is the derivation of nanomaterials directly from the forest, which is now called nanocellulose material, known broadly for its sustainable characteristics [ 141 ]. This factor has pushed the wood industry to convert cellulosic material to nanocellulose with increased strength, low weight, and increased electromagnetic response along with a larger surface area [ 142 ]. These characteristics are then further used as reinforcing agents in different subcategories of wood-based industries, including substrate, stabilizer, electronics, batteries, sensor technologies, food, medicine, and cosmetics industries [ 143 ]. Moreover, functional characteristics such as the durability, UV absorption, fire resistance, and decreased water absorption of wood-based biodegradable products are also being improved with the application of nanomaterials such as nanozinc oxide or nanotitanium oxide [ 144 ]. Similarly, wood biodegradable properties are reduced through the application of nanoencapsulated preservatives to improve the impregnation of wood with the increasing penetration of applied chemicals and a reduced leaching effect.
Cellulosic nanomaterials exhibit nanofibrillar structures which can be made multifunctional for application in construction, furniture, food, pharmaceuticals, and other wood-based industries [ 145 ]. Research is emerging in which promising results are predicted in different industries in which nanofibers, nanofillers, nanoemulsions, nanocomposites, and nano-scaled chemical materials are used to increase the potential advantages of manufactured wood products [ 146 ]. The outstanding properties of nanocellulusice materials have largely curtailed the environmental concerns in the wood industry in the form of their potential renewable characteristics, self-assembling properties, and well-defined architecture. However, there are a few challenges related to such industries, such as cost/benefit analyses, a lack of compatibility and acceptability from the public owing to a lack of proper commercialization, and a persistent knowledge gap in some places [ 145 ]. Therefore, more effort is required to increase the applications and acceptability of nano-based wood products in the market worldwide.
2.15. Nanotechnology and Chemical Industries
Nanotechnology can be easily applied to various chemical compositions such as polymeric substances; this application can bring about structural and functional changes in those chemical materials and can address various industrial applications including medicine, physics, electronics, chemical, and material industries, among others [ 76 , 132 , 138 ]. One such industrial application is in electricity production, in which different nanomaterials driven from silver, golden, and organic sources could be utilized to make the overall production process cheaper and effective [ 147 ]. Another effective application is in the coatings and textile industry, which has already been discussed briefly. In these industries, enzymatic catalysis in combination with nanotechnology accelerates reaction times, saving money and bringing about high-quality final products. Similarly, the water cleaning industry can utilize the benefits of nanomaterials in the form of silver and magnetic nanoparticles to create strong forces of attraction that easily separate heavy material from untreated water [ 148 ]. Similarly, there is a wide range of chemicals that can be potentially upgraded, although the nano scale for application in biomedical industries is discussed under the heading of nanotechnology and medicine.
Another major application of nanotechnology in the chemical industry includes the surfactant industry, which is used for cleaning paper, inks, agrochemicals, drugs, pharmaceuticals, and some food products [ 149 ]. The traditional surfactant application was of great environmental and health concern, but with the newer and improved manufacturing and nanoscaling of surfactants, environmentally friendly applications have been made possible. These newer types may include biosurfactants obtained via the process of fermentation and bio-based surfactants produced through organic manufacturing. More research is required to establish the risks and side effects of these nanochemical agents [ 3 ].
3. Closing Remarks
Nanotechnology, within a short period, has taken over all disciplinary fields of science, whether it is physics, biology, or chemistry. Now, it is predicted to enormously impact manufacturing technology owing to the evidential and proven benefits of micro scaling. Every field of industry, such as computing, information technology, engineering, medicine, agriculture, and food, among others, is now originating an entire new field in association with nanotechnology. These industries are widely known as nanocomputer, nanoengineering, nanoinformatics, nanobiotechnology, nanomedicine, nanoagriculture, and nanofood industries. The most brilliant discoveries are being made in nanomedicine, while the most cost-effective and vibrant technologies are being introduced in materials and mechanical sciences.
The very purpose of nanotechnology, in layman’s terms, is to ease out the manufacturing process and improve the quality of end products and processes. In this regard, it is easy and predictable that it is not difficult for nanotechnology to slowly take out most of the manufacturing process for industrial improvement. With every coming year, more high-tech and more effective-looking nanotechnologies are being introduced. This is smoothing out the basis of a whole new era of nanomindustries. However, the constructive need is to expand the research basis of nanoapplications to entail the rigorous possible pros of this technology and simultaneously figure out a method to deal with the cons of the said technology.
The miniaturization of computer devices has continued for many years and is now being processed at the nanometer scale. However, a gap remains to explore further options for the nanoscaling of computers and complex electronic devices, including computer processors. Moreover, there is an immense need to enable the controlled production and usage of such nanotechnologies in the real world, because if not, they could threaten the world of technology. Scientists should keep on working on producing nanoelectronic devices with more power and energy efficiency. This is important in order to extract the maximum benefits from the hands of nanotechnology and computer sciences [ 5 ].
Under the influence of nanotechnology, food bioprocessing is showing improvement, as proven by several scientific types of research and industrial applications in food chain and agricultural fields. Moreover, the aspect of sustainability is being introduced to convert the environment, food chains, processing industries, and production methods to save some resources for future generations. The usage of precision farming technologies based upon nanoengineering, modern nano-scale fertilizers, and pesticides are of great importance in this regard. Moreover, a combined nanotechnological aspect is also being successfully applied to the food industry, affecting every dimension of packing, sensing, storage, manufacturing, and antimicrobial applications. It is pertinent to say that although the applications of nanotechnology in the food, agriculture, winemaking, poultry, and associated packaging industries are immense, the need is to accurately conduct the risk assessment and potential toxicity of nanomaterials to avoid any damage to the commercial food chains and animal husbandry practices [ 63 ].
The exposure of the nano-based building industry is immense for civil and mechanical engineers; now, we need to use these technologies to actually bring about changes in those countries in which the population is immense, construction material is depleting, and environmental sustainability problems are hovering upon the state. By carefully assessing the sustainability potential of these nanomaterials, their environmental, hazardous, and health risks could be controlled, and they could likely be removed from the construction and automobile industry all over the world with sincere scientific and technical rigor [ 150 ]. It is expected that soon, the construction and automobile industry will commercialize the nanotechnological perspectives alongside sustainability features in their branding strategies. These nano-scale materials could allow the lifecycle management of automotive and construction industries with the provision of sustainable, safe, comfortable, cost-effective, and more eco-friendly automobiles [ 32 ]. The need is to explore the unacknowledged and untapped potential of nanotechnology applications in these industry industries.
Similarly, nanotechnology-based applications in consumer products such as textile and esthetics industries are immense and impressive. Professional development involves the application of nanotechnology-based UV-protective coatings in clothes which are of utmost need with climatic changes [ 73 ]. The application of nanotechnology overcomes the limitations of conventional production methods and makes the process more suitable and green-technology-based. These properties have allowed the textile companies to effectively apply nanotechnology for the manufacture of better products [ 90 ]. With greater consumer acceptability and market demand, millions are spent in the cosmetic industry to enable the further usage of nanotechnology. Researchers are hopeful that nanotechnology would be used to further upgrade the cosmetics industry in the near future [ 123 ].
Furthermore, the breakthrough applications of nanomedicine are not hidden from the scientific community. If nanomedicine is accepted worldwide in the coming years, then the hope is that the domain of diagnosis and treatment will become more customized, personalized, and genetically targeted for individual patients. Treatment options will ultimately become excessive in number and more successful in accomplishment. However, these assumptions will stay a dream if the research remains limited to scientific understanding.
The real outcome will be the application of this research into the experimental domain and clinical practices to make them more productive and beneficial for the medical industry. For this cause, a combined effort of technical ability, professional skills, research, experimentation, and the cooperation of clinicians, physicians, researchers, and technology is imperative. However, despite all functional beneficial characteristics, work needs to be done and more exploration is required to learn more about nanotechnology and its potential in different industries, especially nanomedicine, and to take into account and curtail the risks and harms attached to the said domain of science.
Additionally, climatic conditions, as mentioned before, along with fossil fuel depletion, have pushed scientists to realize a low-energy-consuming and more productive technological renovation in the form of nanoengineered materials [ 48 ]. Now, they are employing nanomaterials to save energy and harvest the maximum remaining natural resources. There is immense ground for the improvement of the fossil fuel industry if nanotechnology could be correctly directed in this industry [ 135 ]. The beneficial applications within the solar industry, gas and oil industry, and conversion fields require comparative cost-effectiveness and efficiency analyses of traditional and nano-based technologies so that appropriate measures could be adopted for the future generation of nano-based products in said industries [ 138 ].
As every new technology is used in industries, linked social, ethical, environmental, and human safety issues arise to halt the pace of progress. These issues need to be addressed and analyzed along with improving nanotechnology so that this technology easily incorporates into different industries without creating social, moral, and ethical concerns. Wide-scale collaboration is needed among technologists, engineers, biologists, and industrials for a prospective future associated with the wide-scale application of nanotechnology in diversified fields.
4. Conclusions
Highly cost-effective and vibrant nanotechnologies are being introduced in materials and mechanical sciences. A comprehensive overview of such technologies has been covered in this study. This review will help researchers and professionals from different fields to delve deeper into the applications of nanotechnology in their particular areas of interest. Indeed, the applications of nanotechnology are immense, yet the risks attached to unlimited applications remain unclear and unpronounced. Thus, more work needs to be linked and carefully ascertained so that further solutions can be determined in the realm of nanotoxicology. Moreover, it is recommended that researchers, technicians, and industrialists should cooperate at the field and educational level to explore options and usefully exploit nanotechnology in field experiments. Additionally, more developments should be made and carefully assessed at the nano scale for a future world, so that we are aware of this massive technology. The magnificent applications of nanotechnology in the industrial world makes one think that soon, the offerings of nanotechnology will be incorporated into every possible industry. However, there is a need to take precautionary measures to be aware of and educate ourselves about the environmental and pollution concerns alongside health-related harms to living things that may arise due to the deviant use of nanotechnology. This is important because the aspect of sustainability is being increasingly considered throughout the world. So, by coupling the aspect of sustainability with nanotechnology, a prosperous future of nanotechnology can be guaranteed.
Funding Statement
K.M.’s work is supported by United Arab Emirates University-UPAR-Grant#G3458, SURE plus Grant#3908 and SDG research programme grant#4065.
Author Contributions
Conceptualization, Y.W. methodology, S.M. validation, S.M., K.M. and Y.W. formal analysis, S.M., K.M. and Y.W. investigation, S.M., K.M. and Y.W. resources, K.M. and Y.W. data curation, S.M., K.M. and Y.W. writing—original draft preparation, S.M. writing—review and editing, S.M., K.M. and Y.W. supervision, Y.W. project administration, K.M. and Y.W. funding acquisition, Y.W. and K.M. All authors have read and agreed to the published version of the manuscript.
Institutional Review Board Statement
Informed consent statement, data availability statement, conflicts of interest.
The authors declare no conflict of interest.
Disclaimer/Publisher’s Note: The statements, opinions and data contained in all publications are solely those of the individual author(s) and contributor(s) and not of MDPI and/or the editor(s). MDPI and/or the editor(s) disclaim responsibility for any injury to people or property resulting from any ideas, methods, instructions or products referred to in the content.
share this!
August 7, 2024
This article has been reviewed according to Science X's editorial process and policies . Editors have highlighted the following attributes while ensuring the content's credibility:
fact-checked
peer-reviewed publication
New system enhances mechanical stability of nanofiber-based bioelectrodes
by Seoul National University College of Engineering
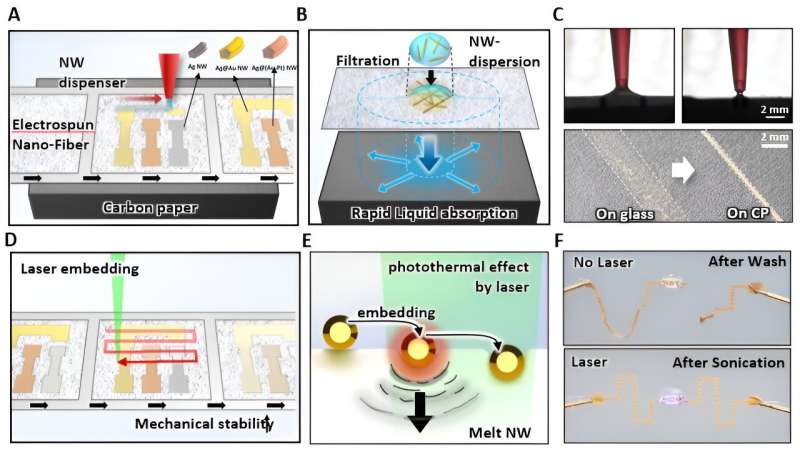
Flexible electronic devices based on electrospun nanofiber membranes (ENM) are attracting significant attention due to their high biocompatibility and excellent mechanical performance. However, patterning conductive materials on fiber substrates typically requires expensive vacuum equipment or additional processes to create separate masks.
To address this, a collaborative research team led by Professor Seung Hwan Ko of the Department of Mechanical Engineering at Seoul National University and Professor C-Yoon Kim of Konkuk University developed a system that induces efficient fluid flow using capillary action by placing a carbon paper support under the nanofiber membrane, enabling the filtration process without the need for vacuum equipment.
The research was published in Advanced Functional Materials on May 29.
This approach enhances mechanical stability by strongly bonding nanowires and substrates through the photothermal effects of lasers during the post-processing stage. In addition, the system demonstrated that circuits remained stable even under strong ultrasonic treatment and that the patterns on the substrate remained intact when manually pulled.
The team validated the strengths of their developed process system and outcomes through various applications, including an in vivo epicardial signal recording ECG electrode, an epidermal electrochemical biosensor, and customized epidermal electromyography (EMG)-based human–machine interface (HMI).
The potential of the electrospun nanofiber membrane (ENM)-based soft electronics in epidermal bioelectronics has gained huge attention with their conformal compatibility with the human body and associated performance improvements.
However, patterning conductive materials on fiber substrates typically requires expensive vacuum equipment or additional processes to create separate masks.
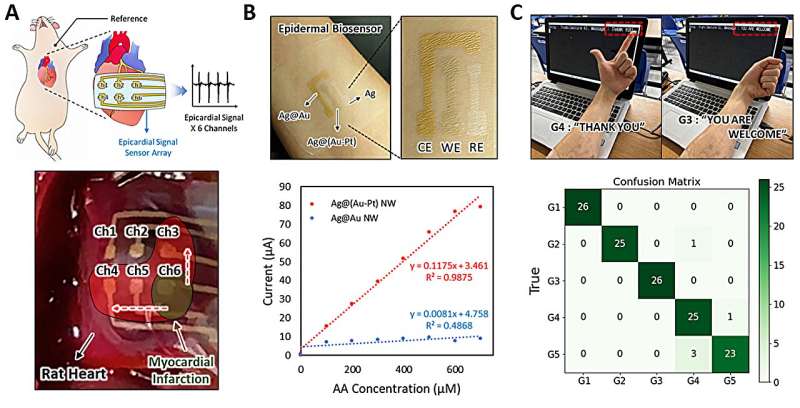
The research team developed a system that enables the filtration process without the need for costly vacuum equipment by placing a carbon paper support under the nanofiber membrane, inducing efficient fluid flow through capillary action.
Using this system, the nanowires and substrates can be strongly bonded through the photothermal effects of lasers during the post-processing stage, enhancing mechanical stability. The system also demonstrated that circuits remained stable under strong ultrasonic treatment and that the patterns on the substrate remained intact when manually pulled.
The research team validated the strengths of their developed process system and outcomes through various applications, including an in vivo epicardial signal recording ECG electrode, an epidermal electrochemical biosensor, and customized epidermal electromyography (EMG)-based human–machine interface (HMI).
Additionally, this research has opened up possibilities for efficiently fabricating electronic devices with high stretchability, breathability, and conductivity, demonstrating potential applications in various health care and medical fields.
Journal information: Advanced Functional Materials
Provided by Seoul National University College of Engineering
Explore further
Feedback to editors

Study reveals how plants decide between life and death
50 minutes ago

New research challenges conventional wisdom on wet surface adhesion
53 minutes ago

Lemurs use long-term memory, smell, and social cues to find food

Research confirms importance of symmetry in pre-ignition fusion experiments

NASA weighs SpaceX rescue for stranded Boeing Starliner crew

Fungi adapt cell walls to evade antifungal drugs

Smells may prime our gut to fight off infection

Scientists use carbon isotopes to track 'forever chemicals'

Newly-discovered star could provide new insights into the evolution of stars

Sichuan Province earthquake offers lessons for landslide prediction from GNSS observations
2 hours ago
Relevant PhysicsForums posts
Need help with pem fuel cell modeling using comsol.
Aug 6, 2024
Stresses along different planes in polycrystalline materials
Aug 2, 2024
What is the equivalent of 42CrMo4 and 42CrMo4V?
Jul 28, 2024
Negative Pressure Wave generation due to pipeline leak
Jul 18, 2024
Deriving displacement tensor from Hencky (true) strain tensor
Jul 15, 2024
Aluminium copper alloy vacuum outgassing
Jul 3, 2024
More from Materials and Chemical Engineering
Related Stories

An adhering, pure conducting polymer hydrogel for medical applications
May 28, 2024

Research team develop next-generation impact-resistant stretchable electronic component
Jun 25, 2024

Enhancing green hydrogen production with robust carbon fabric electrocatalysts
Jun 4, 2024

Chinese scientists develop highly robust stretchable electrode for E-skin applications
Apr 28, 2022

Researchers develop dry transfer printing for flexible electronics

Making seawater drinkable in minutes
Jun 29, 2021
Recommended for you

Researchers show that pesticide contamination is more than apple-skin deep
9 hours ago

Understanding the forces that regulate crystallization by particle attachment

Researchers develop soft gold nanowires for neural interfaces

Molecules get a boost from metallic carbon nanotubes
Aug 5, 2024

First 3D visualization of an aluminum nanocomposite for the auto industry

Investigation into the regime between the nano- and microscale could pave the way for nanoscale technologies
Aug 1, 2024
Let us know if there is a problem with our content
Use this form if you have come across a typo, inaccuracy or would like to send an edit request for the content on this page. For general inquiries, please use our contact form . For general feedback, use the public comments section below (please adhere to guidelines ).
Please select the most appropriate category to facilitate processing of your request
Thank you for taking time to provide your feedback to the editors.
Your feedback is important to us. However, we do not guarantee individual replies due to the high volume of messages.
E-mail the story
Your email address is used only to let the recipient know who sent the email. Neither your address nor the recipient's address will be used for any other purpose. The information you enter will appear in your e-mail message and is not retained by Phys.org in any form.
Newsletter sign up
Get weekly and/or daily updates delivered to your inbox. You can unsubscribe at any time and we'll never share your details to third parties.
More information Privacy policy
Donate and enjoy an ad-free experience
We keep our content available to everyone. Consider supporting Science X's mission by getting a premium account.
E-mail newsletter

Reaction Chemistry & Engineering
Facile fabrication of graphitic carbon nitride/polydopamine/polyurethane foam as a floating photocatalyst for synthetic dye remediation †.

* Corresponding authors
a Master Program in Materials Science and Engineering, Faculty of Mechanical and Aerospace Engineering, Institut Teknologi Bandung, Jl. Ganesha 10, Bandung 40132, West Java, Indonesia
b Graduate school of Science and Technology, University of Tsukuba 1-1-1 Tennodai, Tsukuba, Ibaraki, Japan
c Materials Science and Engineering Research Group, Faculty of Mechanical and Aerospace Engineering, Institut Teknologi Bandung, Jl. Ganesha 10, Bandung 40132, West Java, Indonesia E-mail: [email protected]
d Faculty of Pure and Applied Sciences, University of Tsukuba 1-1-1 Tennodai, Tsukuba, Ibaraki 305-8573, Japan
e Institute for Catalysis, Hokkaido University, Kita21, Nishi10, Kita-ku, Sapporo, Hokkaido 001-0021, Japan
f Research Center for Nanoscience and Nanotechnology, Institut Teknologi Bandung, Ganesha 10, Bandung 40132, West Java, Indonesia
Graphitic carbon nitride (GCN) has attracted much interest in photocatalytic wastewater treatment. However, GCN sinks when applied in wastewater and photogenerated electron–hole (e–h) pairs are easily recombined. In this work, a GCN-based floating photocatalyst with polyurethane foam (PUF) as a floating support and polydopamine (PDA) as the immobilization anchor and photogenerated electron acceptor was prepared via a one-step immobilization process. Compared to the sample prepared via a two-step immobilization process (PUF/PDA/GCN-2), the sample prepared through the one-step immobilization process (PUF/PDA/GCN-1) exhibited a more uniform distribution of GCN particles (as confirmed from SEM images) with a GCN loading content (5.0%) four times greater than that for PUF/PDA/GCN-2 (1.3%), as shown in TGA results. Interestingly, the addition of PDA could increase the photocatalytic performance more than twice that of the sample without PDA addition. Moreover, 4.7 × 10 −5 mmol dye could be degraded per mg of catalyst on PUF/PDA/GCN-1, which is superior to several existing GCN-based floating photocatalysts. This phenomenon was triggered by efficient e–h pair separation, as suggested by the photoluminescence (PL) spectrum and uniform small-sized GCN distribution. The interaction mechanism among PUF, PDA, and GCN is also proposed based on FTIR and XPS studies as well as the photocatalytic mechanism. The successful preparation of floating photocatalysts through a cheap and facile route was thus demonstrated and has potential for large-scale commercialization.
Supplementary files
- Supplementary information PDF (591K)
Article information
Download citation, permissions.

Facile fabrication of graphitic carbon nitride/polydopamine/polyurethane foam as a floating photocatalyst for synthetic dye remediation
I. J. Budiarso, S. Fujita, S. Saito, H. Judawisastra, K. Takeyasu and A. Wibowo, React. Chem. Eng. , 2024, Advance Article , DOI: 10.1039/D4RE00193A
To request permission to reproduce material from this article, please go to the Copyright Clearance Center request page .
If you are an author contributing to an RSC publication, you do not need to request permission provided correct acknowledgement is given.
If you are the author of this article, you do not need to request permission to reproduce figures and diagrams provided correct acknowledgement is given. If you want to reproduce the whole article in a third-party publication (excluding your thesis/dissertation for which permission is not required) please go to the Copyright Clearance Center request page .
Read more about how to correctly acknowledge RSC content .
Social activity
Search articles by author.
This article has not yet been cited.
Advertisements
New biomaterial regrows damaged cartilage in joints
- Feinberg School of Medicine
- Nanotechnology
- Weinberg College
Northwestern University scientists have developed a new bioactive material that successfully regenerated high-quality cartilage in the knee joints of a large-animal model.
Although it looks like a rubbery goo, the material is actually a complex network of molecular components, which work together to mimic cartilage’s natural environment in the body.
In the new study, the researchers applied the material to damaged cartilage in the animals’ knee joints. Within just six months, the researchers observed evidence of enhanced repair, including the growth of new cartilage containing the natural biopolymers (collagen II and proteoglycans), which enable pain-free mechanical resilience in joints.
With more work, the researchers say the new material someday could potentially be used to prevent full knee replacement surgeries, treat degenerative diseases like osteoarthritis and repair sports-related injuries like ACL tears.
The study will be published during the week of August 5 in the Proceedings of the National Academy of Sciences.
“Cartilage is a critical component in our joints,” said Northwestern’s Samuel I. Stupp , who led the study. “When cartilage becomes damaged or breaks down over time, it can have a great impact on people’s overall health and mobility. The problem is that, in adult humans, cartilage does not have an inherent ability to heal. Our new therapy can induce repair in a tissue that does not naturally regenerate. We think our treatment could help address a serious, unmet clinical need.”
A pioneer of regenerative nanomedicine, Stupp is Board of Trustees Professor of Materials Science and Engineering, Chemistry, Medicine and Biomedical Engineering at Northwestern, where he is founding director of the Simpson Querrey Institute for BioNanotechnology and its affiliated center, the Center for Regenerative Nanomedicine . Stupp has appointments in the McCormick School of Engineering , Weinberg College of Arts and Sciences and Feinberg School of Medicine . Jacob Lewis, a former Ph.D. student in Stupp’s laboratory, is the paper’s first author.
What’s in the material?
The new study follows recently published work from the Stupp laboratory, in which the team used “dancing molecules” to activate human cartilage cells to boost the production of proteins that build the tissue matrix. Instead of using dancing molecules, the new study evaluates a hybrid biomaterial also developed in Stupp’s lab. The new biomaterial comprises two components: a bioactive peptide that binds to transforming growth factor beta-1 (TGFb-1) — an essential protein for cartilage growth and maintenance — and modified hyaluronic acid, a natural polysaccharide present in cartilage and the lubricating synovial fluid in joints.
“Many people are familiar with hyaluronic acid because it’s a popular ingredient in skincare products,” Stupp said. “It’s also naturally found in many tissues throughout the human body, including the joints and brain. We chose it because it resembles the natural polymers found in cartilage.”
Stupp’s team integrated the bioactive peptide and chemically modified hyaluronic acid particles to drive the self-organization of nanoscale fibers into bundles that mimic the natural architecture of cartilage. The goal was to create an attractive scaffold for the body’s own cells to regenerate cartilage tissue. Using bioactive signals in the nanoscale fibers, the material encourages cartilage repair by the cells, which populate the scaffold.
Clinically relevant to humans
To evaluate the material’s effectiveness in promoting cartilage growth, the researchers tested it in sheep with cartilage defects in the stifle joint, a complex joint in the hind limbs similar to the human knee. This work was carried out in the laboratory of Mark Markel in the School of Veterinary Medicine at the University of Wisconsin–Madison.
According to Stupp, testing in a sheep model was vital. Much like humans, sheep cartilage is stubborn and incredibly difficult to regenerate. Sheep stifles and human knees also have similarities in weight bearing, size and mechanical loads.
“A study on a sheep model is more predictive of how the treatment will work in humans,” Stupp said. “In other smaller animals, cartilage regeneration occurs much more readily.”
In the study, researchers injected the thick, paste-like material into cartilage defects, where it transformed into a rubbery matrix. Not only did new cartilage grow to fill the defect as the scaffold degraded, but the repaired tissue was consistently higher quality compared to the control.
A lasting solution
In the future, Stupp imagines the new material could be applied to joints during open-joint or arthroscopic surgeries. The current standard of care is microfracture surgery, during which surgeons create tiny fractures in the underlying bone to induce new cartilage growth.
“The main issue with the microfracture approach is that it often results in the formation of fibrocartilage — the same cartilage in our ears — as opposed to hyaline cartilage, which is the one we need to have functional joints,” Stupp said. “By regenerating hyaline cartilage, our approach should be more resistant to wear and tear, fixing the problem of poor mobility and joint pain for the long term while also avoiding the need for joint reconstruction with large pieces of hardware.”
The study, “A bioactive supramolecular and covalent polymer scaffold for cartilage repair in a sheep model,” was supported by the Mike and Mary Sue Shannon Family Fund for Bio-Inspired and Bioactive Materials Systems for Musculoskeletal Regeneration.
Editor’s Picks
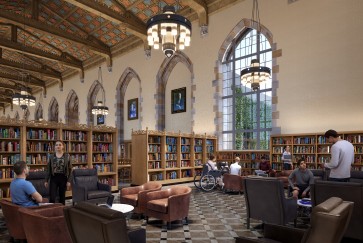
Deering Library undergoing major renovations
Northwestern celebrates the groundbreaking of new ryan field, forget imitation crab — researchers test snackable snails, related stories.
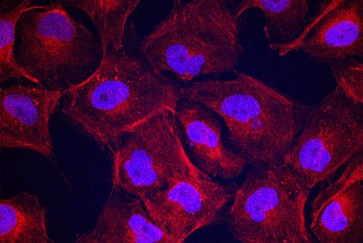
‘Dancing molecules’ heal cartilage damage
Chad mirkin awarded prestigious kavli prize in nanoscience, mark hersam elected to national academy of engineering.
Introducing Nanotechnology to Mechanical and Civil Engineering Students Through Materials Science Courses
- Journal of Nano Education 2(1-2):13-26
- 2(1-2):13-26

- Kennesaw State University

- Naval Postgraduate School

- Kansas State University

- University of New Mexico
Abstract and Figures

Discover the world's research
- 25+ million members
- 160+ million publication pages
- 2.3+ billion citations
- David H. K. Hoe
- Nasser Mahmoud Hasan
- Bianca Dall Gallo Marion

- M. Gail Jones

- Imelda Cossette

- Caleb Hanson

- Scott Miltenberger
- Shane Trinkle

- Philip G. Kosky

- Seyffie Maleki

- Nigel G Shrive

- Ravichandra S Mulukutla

- Mark A. Shannon
- Int J Eng Educ
- Timothy N. Chang
- Puttiphong Jaroonsiriphan
- Recruit researchers
- Join for free
- Login Email Tip: Most researchers use their institutional email address as their ResearchGate login Password Forgot password? Keep me logged in Log in or Continue with Google Welcome back! Please log in. Email · Hint Tip: Most researchers use their institutional email address as their ResearchGate login Password Forgot password? Keep me logged in Log in or Continue with Google No account? Sign up
Information
- Author Services
Initiatives
You are accessing a machine-readable page. In order to be human-readable, please install an RSS reader.
All articles published by MDPI are made immediately available worldwide under an open access license. No special permission is required to reuse all or part of the article published by MDPI, including figures and tables. For articles published under an open access Creative Common CC BY license, any part of the article may be reused without permission provided that the original article is clearly cited. For more information, please refer to https://www.mdpi.com/openaccess .
Feature papers represent the most advanced research with significant potential for high impact in the field. A Feature Paper should be a substantial original Article that involves several techniques or approaches, provides an outlook for future research directions and describes possible research applications.
Feature papers are submitted upon individual invitation or recommendation by the scientific editors and must receive positive feedback from the reviewers.
Editor’s Choice articles are based on recommendations by the scientific editors of MDPI journals from around the world. Editors select a small number of articles recently published in the journal that they believe will be particularly interesting to readers, or important in the respective research area. The aim is to provide a snapshot of some of the most exciting work published in the various research areas of the journal.
Original Submission Date Received: .
- Active Journals
- Find a Journal
- Proceedings Series
- For Authors
- For Reviewers
- For Editors
- For Librarians
- For Publishers
- For Societies
- For Conference Organizers
- Open Access Policy
- Institutional Open Access Program
- Special Issues Guidelines
- Editorial Process
- Research and Publication Ethics
- Article Processing Charges
- Testimonials
- Preprints.org
- SciProfiles
- Encyclopedia
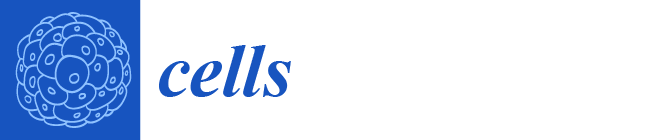
Article Menu
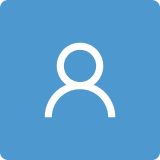
- Subscribe SciFeed
- Recommended Articles
- Google Scholar
- on Google Scholar
- Table of Contents
Find support for a specific problem in the support section of our website.
Please let us know what you think of our products and services.
Visit our dedicated information section to learn more about MDPI.
JSmol Viewer
A review of nanotechnology in microrna detection and drug delivery.

Graphical Abstract
1. Introduction
2. microrna, 3. nanotechnology in microrna detection, 3.1. inorganic nanoparticles, 3.1.1. magnetic nanoparticles, 3.1.2. gold nanoparticles, 3.1.3. quantum dots, 3.2. carbon-based nanoparticles, 3.3. nanopore technology, 4. drug delivery, 4.1. inorganic nanoparticles, 4.2. polymeric nanoparticles, 4.3. lipid nanoparticles, 4.4. carbon-based nanomaterials, 5. discussion, 6. conclusions, conflicts of interest, abbreviations.
CaCO | calcium carbonate |
Cdots | carbon dots |
CNTs | carbon nanotubes |
CQDs | carbon quantum dots |
chNPs | chitosan nanoparticles |
C18P | C18-peptide |
EGFR | epidermal growth factor receptor |
GO/IO | graphene-oxide-loaded iron oxide |
GQDs | graphene quantum dots |
GNPs | gold nanoparticles |
HCC | hepatocellular carcinoma |
IAVs | influenza A viruses |
LNA | locked nucleic acid |
KSHV | Kaposi’s sarcoma-associated herpesvirus |
MNPs | magnetic nanoparticles |
MSCs | mesenchymal stromal cells |
miRNAs | microRNAs |
f-GQDs | modified GQDs |
NDs | nanodiamonds |
ncRNA | non-coding RNAs |
NIR | near-infrared |
P-L-Arg | poly-L-arginine |
PCR | polymerase chain reaction |
PLGA | poly (lactic-co-glycolic acid) |
PLA | poly(l-lactide) |
PEG | polyethylene glycol |
PEL | primary effusion lymphoma |
qPCR | quantitative PCR |
RT-qPCR | quantitative polymerase chain reaction |
QDs | quantum dots |
RT-PCR | reverse transcription polymerase chain reaction |
SPCE | screen-printed carbon electrodes |
SPIONs | superparamagnetic iron-oxide-based nanoparticles |
- Bhushan, B. Introduction to nanotechnology. In Springer Handbook of Nanotechnology ; Springer: Berlin/Heidelberg, Germany, 2017; pp. 1–19. [ Google Scholar ]
- Abou Neel, E.A.; Bozec, L.; Perez, R.A.; Kim, H.-W.; Knowles, J.C. Nanotechnology in dentistry: Prevention, diagnosis, and therapy. Int. J. Nanomed. 2015 , 10 , 6371–6394. [ Google Scholar ] [ CrossRef ]
- McNeil, S.E. Nanotechnology for the biologist. J. Leukoc. Biol. 2005 , 78 , 585–594. [ Google Scholar ] [ CrossRef ] [ PubMed ]
- Pané, S.; Puigmartí-Luis, J.; Bergeles, C.; Chen, X.Z.; Pellicer, E.; Sort, J.; Pocepcova, V.; Ferreira, A.; Nelson, B.J. Imaging Technologies for Biomedical Micro- and Nanoswimmers. Adv. Mater. Technol.-US 2019 , 4 , 1800575. [ Google Scholar ] [ CrossRef ]
- Rajendran, A.K.; Kim, H.D.; Kim, J.-W.; Bae, J.W.; Hwang, N.S. Nanotechnology in tissue engineering and regenerative medicine. Korean J. Chem. Eng. 2023 , 40 , 286–301. [ Google Scholar ] [ CrossRef ]
- Wang, H. MicroRNAs, Parkinson’s disease, and diabetes mellitus. Int. J. Mol. Sci. 2021 , 22 , 2953. [ Google Scholar ] [ CrossRef ] [ PubMed ]
- Wang, H. MicroRNAs and apoptosis in colorectal cancer. Int. J. Mol. Sci. 2020 , 21 , 5353. [ Google Scholar ] [ CrossRef ] [ PubMed ]
- Lewis, B.P.; Burge, C.B.; Bartel, D.P. Conserved seed pairing, often flanked by adenosines, indicates that thousands of human genes are microRNA targets. Cell 2005 , 120 , 15–20. [ Google Scholar ] [ CrossRef ] [ PubMed ]
- Wang, H. Predicting cancer-related MiRNAs using expression profiles in tumor tissue. Curr. Pharm. Biotechnol. 2014 , 15 , 438–444. [ Google Scholar ] [ CrossRef ]
- Wang, H. Predicting microRNA biomarkers for cancer using phylogenetic tree and microarray analysis. Int. J. Mol. Sci. 2016 , 17 , 773. [ Google Scholar ] [ CrossRef ]
- Wang, H. microRNA Pathological Mechanisms between Parkinson’s Disease, Alzheimer’s Disease, Glaucoma, and Macular Degeneration. Expert Rev. Mol. Med. 2023 , 25 , e24. [ Google Scholar ] [ CrossRef ]
- Wang, H.; Taguchi, Y.; Liu, X. miRNAs and neurological diseases. Front. Neurol. 2021 , 12 , 662373. [ Google Scholar ]
- Gentile, G.; Morello, G.; La Cognata, V.; Guarnaccia, M.; Conforti, F.L.; Cavallaro, S. Dysregulated miRNAs as biomarkers and therapeutical targets in neurodegenerative diseases. J. Pers. Med. 2022 , 12 , 770. [ Google Scholar ] [ CrossRef ] [ PubMed ]
- Jung, H.J.; Lee, K.-P.; Kwon, K.-S.; Suh, Y. MicroRNAs in skeletal muscle aging: Current issues and perspectives. J. Gerontol. Ser. A 2019 , 74 , 1008–1014. [ Google Scholar ] [ CrossRef ] [ PubMed ]
- So, J.B.Y.; Kapoor, R.; Zhu, F.; Koh, C.; Zhou, L.; Zou, R.; Tang, Y.C.; Goo, P.C.; Rha, S.Y.; Chung, H.C. Development and validation of a serum microRNA biomarker panel for detecting gastric cancer in a high-risk population. Gut 2021 , 70 , 829–837. [ Google Scholar ] [ CrossRef ] [ PubMed ]
- Wang, N.; Wang, L.; Yang, Y.; Gong, L.; Xiao, B.; Liu, X. A serum exosomal microRNA panel as a potential biomarker test for gastric cancer. Biochem. Biophys. Res. Commun. 2017 , 493 , 1322–1328. [ Google Scholar ] [ CrossRef ] [ PubMed ]
- Wang, H. Phylogenetic analysis to explore the association between anti-NMDA receptor encephalitis and tumors based on microRNA biomarkers. Biomolecules 2019 , 9 , 572. [ Google Scholar ] [ CrossRef ] [ PubMed ]
- Wang, H. MicroRNAs, Multiple Sclerosis, and Depression. Int. J. Mol. Sci. 2021 , 22 , 7802. [ Google Scholar ] [ CrossRef ] [ PubMed ]
- Wang, H. MicroRNA, Diabetes Mellitus and Colorectal Cancer. Biomedicines 2020 , 8 , 530. [ Google Scholar ] [ CrossRef ]
- Ouyang, T.; Liu, Z.; Han, Z.; Ge, Q. MicroRNA detection specificity: Recent advances and future perspective. Anal. Chem. 2019 , 91 , 3179–3186. [ Google Scholar ] [ CrossRef ]
- Git, A.; Dvinge, H.; Salmon-Divon, M.; Osborne, M.; Kutter, C.; Hadfield, J.; Bertone, P.; Caldas, C. Systematic comparison of microarray profiling, real-time PCR, and next-generation sequencing technologies for measuring differential microRNA expression. RNA 2010 , 16 , 991–1006. [ Google Scholar ] [ CrossRef ]
- Shabaninejad, Z.; Yousefi, F.; Movahedpour, A.; Ghasemi, Y.; Dokanehiifard, S.; Rezaei, S.; Aryan, R.; Savardashtaki, A.; Mirzaei, H. Electrochemical-based biosensors for microRNA detection: Nanotechnology comes into view. Anal. Biochem. 2019 , 581 , 113349. [ Google Scholar ] [ CrossRef ] [ PubMed ]
- Alam, M.K. Nanocarrier-Based Drug Delivery Systems using Microfluidic-Assisted Techniques. Adv. NanoBiomed. Res. 2023 , 3 , 2300041. [ Google Scholar ] [ CrossRef ]
- Chandrasekaran, A.R.; Punnoose, J.A.; Zhou, L.; Dey, P.; Dey, B.K.; Halvorsen, K. DNA nanotechnology approaches for microRNA detection and diagnosis. Nucleic Acids Res. 2019 , 47 , 10489–10505. [ Google Scholar ] [ CrossRef ] [ PubMed ]
- Scheideler, M.; Vidakovic, I.; Prassl, R. Lipid nanocarriers for microRNA delivery. Chem. Phys. Lipids 2020 , 226 , 104837. [ Google Scholar ] [ CrossRef ] [ PubMed ]
- Sukocheva, O.A.; Liu, J.; Neganova, M.E.; Beeraka, N.M.; Aleksandrova, Y.R.; Manogaran, P.; Grigorevskikh, E.M.; Chubarev, V.N.; Fan, R. Perspectives of using microRNA-loaded nanocarriers for epigenetic reprogramming of drug resistant colorectal cancers. In Seminars in Cancer Biology ; Elsevier: Amsterdam, The Netherlands, 2022; pp. 358–375. [ Google Scholar ]
- Dasgupta, I.; Chatterjee, A. Recent advances in miRNA delivery systems. Methods Protoc. 2021 , 4 , 10. [ Google Scholar ] [ CrossRef ] [ PubMed ]
- Awasthi, R.; Rathbone, M.J.; Hansbro, P.M.; Bebawy, M.; Dua, K. Therapeutic prospects of microRNAs in cancer treatment through nanotechnology. Drug Deliv. Transl. Res. 2018 , 8 , 97–110. [ Google Scholar ] [ CrossRef ]
- Lee, R.C.; Feinbaum, R.L.; Ambros, V. The C. elegans heterochronic gene lin-4 encodes small RNAs with antisense complementarity to lin-14. Cell 1993 , 75 , 843–854. [ Google Scholar ] [ CrossRef ] [ PubMed ]
- Hammond, S.M. An overview of microRNAs. Adv. Drug Deliv. Rev. 2015 , 87 , 3–14. [ Google Scholar ] [ CrossRef ]
- Kozomara, A.; Birgaoanu, M.; Griffiths-Jones, S. miRBase: From microRNA sequences to function. Nucleic Acids Res. 2019 , 47 , D155–D162. [ Google Scholar ] [ CrossRef ]
- Fromm, B.; Domanska, D.; Høye, E.; Ovchinnikov, V.; Kang, W.; Aparicio-Puerta, E.; Johansen, M.; Flatmark, K.; Mathelier, A.; Hovig, E. MirGeneDB 2.0: The metazoan microRNA complement. Nucleic Acids Res. 2020 , 48 , D132–D141. [ Google Scholar ] [ CrossRef ]
- Condrat, C.E.; Thompson, D.C.; Barbu, M.G.; Bugnar, O.L.; Boboc, A.; Cretoiu, D.; Suciu, N.; Cretoiu, S.M.; Voinea, S.C. miRNAs as biomarkers in disease: Latest findings regarding their role in diagnosis and prognosis. Cells 2020 , 9 , 276. [ Google Scholar ] [ CrossRef ] [ PubMed ]
- Huang, W. MicroRNAs: Biomarkers, diagnostics, and therapeutics. In Bioinformatics in MicroRNA Research ; Springer: Berlin/Heidelberg, Germany, 2017; pp. 57–67. [ Google Scholar ]
- Guay, C.; Regazzi, R. Circulating microRNAs as novel biomarkers for diabetes mellitus. Nat. Rev. Endocrinol. 2013 , 9 , 513–521. [ Google Scholar ] [ CrossRef ] [ PubMed ]
- Chen, Y.-H.; Wang, H. The association between depression and gastroesophageal reflux based on phylogenetic analysis of miRNA biomarkers. Curr. Med. Chem. 2020 , 27 , 6536–6547. [ Google Scholar ] [ CrossRef ] [ PubMed ]
- Chen, Y.-H.; Wang, H. The association between migraine and depression based on miRNA biomarkers and cohort studies. Curr. Med. Chem. 2021 , 28 , 5648–5656. [ Google Scholar ] [ CrossRef ] [ PubMed ]
- Wang, H. The distance distribution of human microRNAs in MirGeneDB database. Sci. Rep. 2022 , 12 , 17696. [ Google Scholar ] [ CrossRef ] [ PubMed ]
- Wang, H.; Ho, C. The Human Pre-miRNA Distance Distribution for Exploring Disease Association. Int. J. Mol. Sci. 2023 , 24 , 1009. [ Google Scholar ] [ CrossRef ] [ PubMed ]
- Liu, K.; Tong, H.; Li, T.; Wang, X.; Chen, Y. Research progress in molecular biology related quantitative methods of MicroRNA. Am. J. Transl. Res. 2020 , 12 , 3198. [ Google Scholar ] [ PubMed ]
- Hunt, E.A.; Broyles, D.; Head, T.; Deo, S.K. MicroRNA detection: Current technology and research strategies. Annu. Rev. Anal. Chem. 2015 , 8 , 217–237. [ Google Scholar ]
- Li, W.; Ruan, K. MicroRNA detection by microarray. Anal. Bioanal. Chem. 2009 , 394 , 1117–1124. [ Google Scholar ] [ CrossRef ]
- Costa, V.; Angelini, C.; De Feis, I.; Ciccodicola, A. Uncovering the complexity of transcriptomes with RNA-Seq. BioMed Res. Int. 2010 , 2010 , 853916. [ Google Scholar ] [ CrossRef ]
- Hu, Y.; Lan, W.; Miller, D. Next-generation sequencing for MicroRNA expression profile. Bioinform. MicroRNA Res. 2017 , 1617 , 169–177. [ Google Scholar ]
- Willenbrock, H.; Salomon, J.; Søkilde, R.; Barken, K.B.; Hansen, T.N.; Nielsen, F.C.; Møller, S.; Litman, T. Quantitative miRNA expression analysis: Comparing microarrays with next-generation sequencing. RNA 2009 , 15 , 2028–2034. [ Google Scholar ] [ CrossRef ] [ PubMed ]
- Hurd, P.J.; Nelson, C.J. Advantages of next-generation sequencing versus the microarray in epigenetic research. Brief Funct. Genom. Proteomic 2009 , 8 , 174–183. [ Google Scholar ] [ CrossRef ] [ PubMed ]
- De la Rosa, C.; Reyes, J.L. Northern blot analysis of microRNAs and other small RNAs in plants. Plant MicroRNAs Methods Protoc. 2019 , 1932 , 121–129. [ Google Scholar ]
- Várallyay, E.; Burgyán, J.; Havelda, Z. MicroRNA detection by northern blotting using locked nucleic acid probes. Nat. Protoc. 2008 , 3 , 190–196. [ Google Scholar ] [ CrossRef ] [ PubMed ]
- Válóczi, A.; Hornyik, C.; Varga, N.; Burgyán, J.; Kauppinen, S.; Havelda, Z. Sensitive and specific detection of microRNAs by northern blot analysis using LNA-modified oligonucleotide probes. Nucleic Acids Res. 2004 , 32 , e175. [ Google Scholar ] [ CrossRef ] [ PubMed ]
- Várallyay, E.; Burgyán, J.; Havelda, Z. Detection of microRNAs by Northern blot analyses using LNA probes. Methods 2007 , 43 , 140–145. [ Google Scholar ] [ CrossRef ] [ PubMed ]
- Zhu, H.; Zhang, H.; Xu, Y.; Laššáková, S.; Korabečná, M.; Neužil, P. PCR past, present and future. Biotechniques 2020 , 69 , 317–325. [ Google Scholar ] [ CrossRef ]
- Schmittgen, T.D.; Lee, E.J.; Jiang, J.; Sarkar, A.; Yang, L.; Elton, T.S.; Chen, C. Real-time PCR quantification of precursor and mature microRNA. Methods 2008 , 44 , 31–38. [ Google Scholar ] [ CrossRef ]
- Dymond, J.S. Explanatory chapter: Quantitative PCR. In Methods in Enzymology ; Elsevier: Amsterdam, The Netherlands, 2013; Volume 529, pp. 279–289. [ Google Scholar ]
- Bustin, S.; Benes, V.; Nolan, T.; Pfaffl, M. Quantitative real-time RT-PCR–a perspective. J. Mol. Endocrinol. 2005 , 34 , 597–601. [ Google Scholar ]
- Adams, G. A beginner’s guide to RT-PCR, qPCR and RT-qPCR. Biochemist 2020 , 42 , 48–53. [ Google Scholar ] [ CrossRef ]
- Norouzi, M.; Yasamineh, S.; Montazeri, M.; Dadashpour, M.; Sheervalilou, R.; Abasi, M.; Pilehvar-Soltanahmadi, Y. Recent advances on nanomaterials-based fluorimetric approaches for microRNAs detection. Mater. Sci. Eng. C 2019 , 104 , 110007. [ Google Scholar ] [ CrossRef ] [ PubMed ]
- Zhang, S.; Cheng, Z.; Wang, Y.; Han, T. The risks of miRNA therapeutics: In a drug target perspective. Drug Des. Dev. Ther. 2021 , 15 , 721–733. [ Google Scholar ] [ CrossRef ] [ PubMed ]
- Asakiya, C.; Zhu, L.; Yuhan, J.; Zhu, L.; Huang, K.; Xu, W. Current progress of miRNA-derivative nucleotide drugs: Modifications, delivery systems, applications. Expert Opin. Drug Deliv. 2022 , 19 , 435–450. [ Google Scholar ] [ CrossRef ] [ PubMed ]
- Gessner, I.; Fries, J.W.; Brune, V.; Mathur, S. Magnetic nanoparticle-based amplification of microRNA detection in body fluids for early disease diagnosis. J. Mater. Chem. B 2021 , 9 , 9–22. [ Google Scholar ] [ CrossRef ] [ PubMed ]
- Xu, S.; Hossaini Nasr, S.; Chen, D.; Zhang, X.; Sun, L.; Huang, X.; Qian, C. MiRNA extraction from cell-free biofluid using protein corona formed around carboxyl magnetic nanoparticles. ACS Biomater. Sci. Eng. 2018 , 4 , 654–662. [ Google Scholar ] [ CrossRef ] [ PubMed ]
- Balaban Hanoglu, S.; Harmanci, D.; Ucar, N.; Evran, S.; Timur, S. Recent Approaches in Magnetic Nanoparticle-Based Biosensors of miRNA Detection. Magnetochemistry 2023 , 9 , 23. [ Google Scholar ] [ CrossRef ]
- Tavallaie, R.; McCarroll, J.; Le Grand, M.; Ariotti, N.; Schuhmann, W.; Bakker, E.; Tilley, R.D.; Hibbert, D.B.; Kavallaris, M.; Gooding, J.J. Nucleic acid hybridization on an electrically reconfigurable network of gold-coated magnetic nanoparticles enables microRNA detection in blood. Nat. Nanotechnol. 2018 , 13 , 1066–1071. [ Google Scholar ] [ CrossRef ]
- Yuan, Y.-H.; Wu, Y.-D.; Chi, B.-Z.; Wen, S.-H.; Liang, R.-P.; Qiu, J.-D. Simultaneously electrochemical detection of microRNAs based on multifunctional magnetic nanoparticles probe coupling with hybridization chain reaction. Biosens. Bioelectron. 2017 , 97 , 325–331. [ Google Scholar ] [ CrossRef ]
- Ali, A.; Zafar, H.; Zia, M.; ul Haq, I.; Phull, A.R.; Ali, J.S.; Hussain, A. Synthesis, characterization, applications, and challenges of iron oxide nanoparticles. Nanotechnol. Sci. Appl. 2016 , 9 , 49–67. [ Google Scholar ] [ CrossRef ]
- Islam, M.N.; Gorgannezhad, L.; Masud, M.K.; Tanaka, S.; Hossain, M.S.A.; Yamauchi, Y.; Nguyen, N.T.; Shiddiky, M.J. Graphene-oxide-loaded superparamagnetic iron oxide nanoparticles for ultrasensitive electrocatalytic detection of MicroRNA. ChemElectroChem 2018 , 5 , 2488–2495. [ Google Scholar ] [ CrossRef ]
- Dreaden, E.C.; Alkilany, A.M.; Huang, X.; Murphy, C.J.; El-Sayed, M.A. The golden age: Gold nanoparticles for biomedicine. Chem. Soc. Rev. 2012 , 41 , 2740–2779. [ Google Scholar ] [ CrossRef ] [ PubMed ]
- Sztandera, K.; Gorzkiewicz, M.; Klajnert-Maculewicz, B. Gold nanoparticles in cancer treatment. Mol. Pharm. 2018 , 16 , 1–23. [ Google Scholar ] [ CrossRef ] [ PubMed ]
- Guo, Y.; Wang, Y.; Yang, G.; Xu, J.-J.; Chen, H.-Y. MicroRNA-mediated signal amplification coupled with GNP/dendrimers on a mass-sensitive biosensor and its applications in intracellular microRNA quantification. Biosens. Bioelectron. 2016 , 85 , 897–902. [ Google Scholar ] [ CrossRef ]
- Mohammadniaei, M.; Yoon, J.; Lee, T.; Choi, J.-W. Spectroelectrochemical detection of microRNA-155 based on functional RNA immobilization onto ITO/GNP nanopattern. J. Biotechnol. 2018 , 274 , 40–46. [ Google Scholar ] [ CrossRef ] [ PubMed ]
- Coutinho, C.; Somoza, Á. MicroRNA sensors based on gold nanoparticles. Anal. Bioanal. Chem. 2019 , 411 , 1807–1824. [ Google Scholar ] [ CrossRef ] [ PubMed ]
- Zhou, J.; Yang, Y.; Zhang, C.-y. Toward biocompatible semiconductor quantum dots: From biosynthesis and bioconjugation to biomedical application. Chem. Rev. 2015 , 115 , 11669–11717. [ Google Scholar ] [ CrossRef ] [ PubMed ]
- Wang, L.; Xu, D.; Gao, J.; Chen, X.; Duo, Y.; Zhang, H. Semiconducting quantum dots: Modification and applications in biomedical science. Sci. China Mater. 2020 , 63 , 1631–1650. [ Google Scholar ] [ CrossRef ]
- Goryacheva, O.A.; Novikova, A.S.; Drozd, D.D.; Pidenko, P.S.; Ponomaryeva, T.S.; Bakal, A.A.; Mishra, P.K.; Beloglazova, N.V.; Goryacheva, I.Y. Water-dispersed luminescent quantum dots for miRNA detection. TrAC Trends Anal. Chem. 2019 , 111 , 197–205. [ Google Scholar ] [ CrossRef ]
- Goryacheva, O.; Mishra, P.; Goryacheva, I.Y. Luminescent quantum dots for miRNA detection. Talanta 2018 , 179 , 456–465. [ Google Scholar ] [ CrossRef ]
- Hu, O.; Li, Z.; Tong, Y.; Wang, Q.; Chen, Z. DNA functionalized double quantum dots-based fluorescence biosensor for one-step simultaneous detection of multiple microRNAs. Talanta 2021 , 235 , 122763. [ Google Scholar ] [ CrossRef ] [ PubMed ]
- Martins, C.S.; LaGrow, A.P.; Prior, J.o.A. Quantum dots for cancer-related miRNA monitoring. ACS Sens. 2022 , 7 , 1269–1299. [ Google Scholar ] [ CrossRef ]
- Ajgaonkar, R.; Lee, B.; Valimukhametova, A.; Nguyen, S.; Gonzalez-Rodriguez, R.; Coffer, J.; Akkaraju, G.R.; Naumov, A.V. Detection of pancreatic cancer miRNA with biocompatible nitrogen-doped graphene quantum dots. Materials 2022 , 15 , 5760. [ Google Scholar ] [ CrossRef ] [ PubMed ]
- Borghei, Y.S.; Hosseini, M.; Ganjali, M.R. A label-free luminescent light switching system for miRNA detection based on two color quantum dots. J. Photochem. Photobiol. A Chem. 2020 , 391 , 112351. [ Google Scholar ] [ CrossRef ]
- Ma, Y.; Zhang, Y.; William, W.Y. Near infrared emitting quantum dots: Synthesis, luminescence properties and applications. J. Mater. Chem. C 2019 , 7 , 13662–13679. [ Google Scholar ] [ CrossRef ]
- Ratre, P.; Nazeer, N.; Kumari, R.; Thareja, S.; Jain, B.; Tiwari, R.; Kamthan, A.; Srivastava, R.K.; Mishra, P.K. Carbon-based fluorescent nano-biosensors for the detection of cell-free circulating MicroRNAs. Biosensors 2023 , 13 , 226. [ Google Scholar ] [ CrossRef ]
- Hosseini, S.M.; Mohammadnejad, J.; Najafi-Taher, R.; Zadeh, Z.B.; Tanhaei, M.; Ramakrishna, S. Multifunctional carbon-based nanoparticles: Theranostic applications in cancer therapy and diagnosis. ACS Appl. Bio Mater. 2023 , 6 , 1323–1338. [ Google Scholar ] [ CrossRef ] [ PubMed ]
- Eivazzadeh-Keihan, R.; Noruzi, E.B.; Chidar, E.; Jafari, M.; Davoodi, F.; Kashtiaray, A.; Gorab, M.G.; Hashemi, S.M.; Javanshir, S.; Cohan, R.A. Applications of carbon-based conductive nanomaterials in biosensors. Chem. Eng. J. 2022 , 442 , 136183. [ Google Scholar ] [ CrossRef ]
- Wang, J.; Wen, J.; Yan, H. Recent applications of carbon nanomaterials for microRNA electrochemical sensing. Chem.–Asian J. 2021 , 16 , 114–128. [ Google Scholar ] [ CrossRef ]
- Gutiérrez-Gálvez, L.; García-Mendiola, T.; Gutiérrez-Sánchez, C.; Guerrero-Esteban, T.; García-Diego, C.; Buendía, I.; García-Bermejo, M.L.; Pariente, F.; Lorenzo, E. Carbon nanodot–based electrogenerated chemiluminescence biosensor for miRNA-21 detection. Microchim. Acta 2021 , 188 , 1–12. [ Google Scholar ] [ CrossRef ]
- Su, J.; Liu, W.; Chen, S.; Deng, W.; Dou, Y.; Zhao, Z.; Li, J.; Li, Z.; Yin, H.; Ding, X. A carbon-based DNA framework nano–bio interface for biosensing with high sensitivity and a high signal-to-noise ratio. ACS Sens. 2020 , 5 , 3979–3987. [ Google Scholar ] [ CrossRef ] [ PubMed ]
- Zhang, C.; Miao, P.; Sun, M.; Yan, M.; Liu, H. Progress in miRNA detection using graphene material–based biosensors. Small 2019 , 15 , 1901867. [ Google Scholar ] [ CrossRef ]
- Shuai, H.-L.; Huang, K.-J.; Xing, L.-L.; Chen, Y.-X. Ultrasensitive electrochemical sensing platform for microRNA based on tungsten oxide-graphene composites coupling with catalyzed hairpin assembly target recycling and enzyme signal amplification. Biosens. Bioelectron. 2016 , 86 , 337–345. [ Google Scholar ] [ CrossRef ] [ PubMed ]
- Shuai, H.-L.; Huang, K.-J.; Zhang, W.-J.; Cao, X.; Jia, M.-P. Sandwich-type microRNA biosensor based on magnesium oxide nanoflower and graphene oxide–gold nanoparticles hybrids coupling with enzyme signal amplification. Sens. Actuators B Chem. 2017 , 243 , 403–411. [ Google Scholar ] [ CrossRef ]
- Lin, B.; Hui, J.; Mao, H. Nanopore technology and its applications in gene sequencing. Biosensors 2021 , 11 , 214. [ Google Scholar ] [ CrossRef ]
- Ying, Y.-L.; Hu, Z.-L.; Zhang, S.; Qing, Y.; Fragasso, A.; Maglia, G.; Meller, A.; Bayley, H.; Dekker, C.; Long, Y.-T. Nanopore-based technologies beyond DNA sequencing. Nat. Nanotechnol. 2022 , 17 , 1136–1146. [ Google Scholar ] [ CrossRef ]
- Takeuchi, N.; Hiratani, M.; Kawano, R. Pattern recognition of microRNA expression in body fluids using nanopore decoding at subfemtomolar concentrations. JACS Au 2022 , 2 , 1829–1838. [ Google Scholar ] [ CrossRef ]
- Wanunu, M.; Dadosh, T.; Ray, V.; Jin, J.; McReynolds, L.; Drndić, M. Rapid electronic detection of probe-specific microRNAs using thin nanopore sensors. Nat. Nanotechnol. 2010 , 5 , 807–814. [ Google Scholar ] [ CrossRef ]
- Koch, C.; Reilly-O’Donnell, B.; Gutierrez, R.; Lucarelli, C.; Ng, F.S.; Gorelik, J.; Ivanov, A.P.; Edel, J.B. Nanopore sequencing of DNA-barcoded probes for highly multiplexed detection of microRNA, proteins and small biomarkers. Nat. Nanotechnol. 2023 , 18 , 1483–1491. [ Google Scholar ] [ CrossRef ]
- Huang, H.-C.; Barua, S.; Sharma, G.; Dey, S.K.; Rege, K. Inorganic nanoparticles for cancer imaging and therapy. J. Control. Release 2011 , 155 , 344–357. [ Google Scholar ] [ CrossRef ]
- Boca, S.; Gulei, D.; Zimta, A.-A.; Onaciu, A.; Magdo, L.; Tigu, A.B.; Ionescu, C.; Irimie, A.; Buiga, R.; Berindan-Neagoe, I. Nanoscale delivery systems for microRNAs in cancer therapy. Cell. Mol. Life Sci. 2020 , 77 , 1059–1086. [ Google Scholar ] [ CrossRef ] [ PubMed ]
- Yu, M.; Lei, B.; Gao, C.; Yan, J.; Ma, P.X. Optimizing surface-engineered ultra-small gold nanoparticles for highly efficient miRNA delivery to enhance osteogenic differentiation of bone mesenchymal stromal cells. Nano Res. 2017 , 10 , 49–63. [ Google Scholar ] [ CrossRef ]
- Schade, A.; Delyagina, E.; Scharfenberg, D.; Skorska, A.; Lux, C.; David, R.; Steinhoff, G. Innovative strategy for microRNA delivery in human mesenchymal stem cells via magnetic nanoparticles. Int. J. Mol. Sci. 2013 , 14 , 10710–10726. [ Google Scholar ] [ CrossRef ]
- Assali, A.; Akhavan, O.; Adeli, M.; Razzazan, S.; Dinarvand, R.; Zanganeh, S.; Soleimani, M.; Dinarvand, M.; Atyabi, F. Multifunctional core-shell nanoplatforms (gold@ graphene oxide) with mediated NIR thermal therapy to promote miRNA delivery. Nanomed. Nanotechnol. Biol. Med. 2018 , 14 , 1891–1903. [ Google Scholar ] [ CrossRef ] [ PubMed ]
- Kröger, N.; Poulsen, N. Diatoms—From cell wall biogenesis to nanotechnology. Annu. Rev. Genet. 2008 , 42 , 83–107. [ Google Scholar ] [ CrossRef ] [ PubMed ]
- Haddick, L.; Zhang, W.; Reinhard, S.; Möller, K.; Engelke, H.; Wagner, E.; Bein, T. Particle-size-dependent delivery of antitumoral miRNA using targeted mesoporous silica nanoparticles. Pharmaceutics 2020 , 12 , 505. [ Google Scholar ] [ CrossRef ] [ PubMed ]
- Wang, Y.; Xie, Y.; Kilchrist, K.V.; Li, J.; Duvall, C.L.; Oupický, D. Endosomolytic and tumor-penetrating mesoporous silica nanoparticles for siRNA/miRNA combination cancer therapy. ACS Appl. Mater. Interfaces 2020 , 12 , 4308–4322. [ Google Scholar ] [ CrossRef ] [ PubMed ]
- Yang, T.; Ao, Y.; Feng, J.; Wang, C.; Zhang, J. Biomineralization inspired synthesis of CaCO3-based DDS for pH-responsive release of anticancer drug. Mater. Today Commun. 2021 , 27 , 102256. [ Google Scholar ] [ CrossRef ]
- Roy, B.; Ghose, S.; Biswas, S. Therapeutic strategies for miRNA delivery to reduce hepatocellular carcinoma. In Seminars in Cell & Developmental Biology ; Elsevier: Amsterdam, The Netherlands, 2022; pp. 134–144. [ Google Scholar ]
- Zhao, P.; Li, M.; Wang, Y.; Chen, Y.; He, C.; Zhang, X.; Yang, T.; Lu, Y.; You, J.; Lee, R.J. Enhancing anti-tumor efficiency in hepatocellular carcinoma through the autophagy inhibition by miR-375/sorafenib in lipid-coated calcium carbonate nanoparticles. Acta Biomater. 2018 , 72 , 248–255. [ Google Scholar ] [ CrossRef ]
- Ding, Q.J.; Remy, M.T.; Upara, C.; Hu, J.; Mora Mata, A.; Haes, A.J.; Lanzel, E.; Sun, H.; Buchakjian, M.R.; Hong, L. CaCO3 Nanoparticles Delivering MicroRNA-200c Suppress Oral Squamous Cell Carcinoma. J. Dent. Res. 2024 , 103 , 147–155. [ Google Scholar ] [ CrossRef ]
- Yoo, S.S.; Razzak, R.; Bédard, E.; Guo, L.; Shaw, A.R.; Moore, R.B.; Roa, W.H. Layered gadolinium-based nanoparticle as a novel delivery platform for microRNA therapeutics. Nanotechnology 2014 , 25 , 425102. [ Google Scholar ] [ CrossRef ] [ PubMed ]
- Yin, P.T.; Shah, B.P.; Lee, K.-B. Combined magnetic nanoparticle-based microRNA and hyperthermia therapy to enhance apoptosis in brain cancer cells. Small (Weinh. Der Bergstr. Ger.) 2014 , 10 , 4106. [ Google Scholar ] [ CrossRef ] [ PubMed ]
- Kara, G.; Ozpolat, B. SPIONs: Superparamagnetic iron oxide-based nanoparticles for the delivery of microRNAi-therapeutics in cancer. Biomed. Microdevices 2024 , 26 , 16. [ Google Scholar ] [ CrossRef ]
- Reimondez-Troitiño, S.; González-Aramundiz, J.V.; Ruiz-Bañobre, J.; López-López, R.; Alonso, M.J.; Csaba, N.; de la Fuente, M. Versatile protamine nanocapsules to restore miR-145 levels and interfere tumor growth in colorectal cancer cells. Eur. J. Pharm. Biopharm. 2019 , 142 , 449–459. [ Google Scholar ] [ CrossRef ]
- Mohamed, A.; Kunda, N.K.; Ross, K.; Hutcheon, G.A.; Saleem, I.Y. Polymeric nanoparticles for the delivery of miRNA to treat Chronic Obstructive Pulmonary Disease (COPD). Eur. J. Pharm. Biopharm. 2019 , 136 , 1–8. [ Google Scholar ] [ CrossRef ]
- Zheng, B.; Chen, L.; Pan, C.-C.; Wang, J.-Z.; Lu, G.-R.; Yang, S.-X.; Xue, Z.-X.; Wang, F.-Y.; Xu, C.-L. Targeted delivery of miRNA-204-5p by PEGylated polymer nanoparticles for colon cancer therapy. Nanomedicine 2018 , 13 , 769–785. [ Google Scholar ] [ CrossRef ]
- Dhuri, K.; Vyas, R.N.; Blumenfeld, L.; Verma, R.; Bahal, R. Nanoparticle delivered anti-miR-141-3p for stroke therapy. Cells 2021 , 10 , 1011. [ Google Scholar ] [ CrossRef ]
- Lopez-Bertoni, H.; Kozielski, K.L.; Rui, Y.; Lal, B.; Vaughan, H.; Wilson, D.R.; Mihelson, N.; Eberhart, C.G.; Laterra, J.; Green, J.J. Bioreducible polymeric nanoparticles containing multiplexed cancer stem cell regulating miRNAs inhibit glioblastoma growth and prolong survival. Nano Lett. 2018 , 18 , 4086–4094. [ Google Scholar ] [ CrossRef ] [ PubMed ]
- Nguyen, M.-A.; Wyatt, H.; Susser, L.; Geoffrion, M.; Rasheed, A.; Duchez, A.-C.; Cottee, M.L.; Afolayan, E.; Farah, E.; Kahiel, Z. Delivery of microRNAs by chitosan nanoparticles to functionally alter macrophage cholesterol efflux in vitro and in vivo. ACS Nano 2019 , 13 , 6491–6505. [ Google Scholar ] [ CrossRef ]
- Plaza-Oliver, M.; Santander-Ortega, M.J.; Lozano, M.V. Current approaches in lipid-based nanocarriers for oral drug delivery. Drug Deliv. Transl. Res. 2021 , 11 , 471–497. [ Google Scholar ] [ CrossRef ]
- Campani, V.; De Rosa, G.; Misso, G.; Zarone, M.R.; Grimaldi, A. Lipid nanoparticles to deliver miRNA in cancer. Curr. Pharm. Biotechnol. 2016 , 17 , 741–749. [ Google Scholar ] [ CrossRef ]
- Lujan, H.; Griffin, W.C.; Taube, J.H.; Sayes, C.M. Synthesis and characterization of nanometer-sized liposomes for encapsulation and microRNA transfer to breast cancer cells. Int. J. Nanomed. 2019 , 14 , 5159–5173. [ Google Scholar ] [ CrossRef ]
- Hsu, S.-h.; Yu, B.; Wang, X.; Lu, Y.; Schmidt, C.R.; Lee, R.J.; Lee, L.J.; Jacob, S.T.; Ghoshal, K. Cationic lipid nanoparticles for therapeutic delivery of siRNA and miRNA to murine liver tumor. Nanomed. Nanotechnol. Biol. Med. 2013 , 9 , 1169–1180. [ Google Scholar ] [ CrossRef ]
- Taghavi, S.; Abnous, K.; Taghdisi, S.M.; Ramezani, M.; Alibolandi, M. Hybrid carbon-based materials for gene delivery in cancer therapy. J. Control. Release 2020 , 318 , 158–175. [ Google Scholar ] [ CrossRef ]
- Mohajeri, M.; Behnam, B.; Sahebkar, A. Biomedical applications of carbon nanomaterials: Drug and gene delivery potentials. J. Cell. Physiol. 2019 , 234 , 298–319. [ Google Scholar ] [ CrossRef ] [ PubMed ]
- Xu, J.-R.; Xie, Y.; Li, J.-W.; Liu, R.; Chen, M.; Ren, Y.-X.; Luo, Q.; Duan, J.-L.; Bao, C.-J.; Liu, Y.-X. Development of fullerene nanospherical miRNA and application in overcoming resistant breast cancer. Mater. Today Chem. 2022 , 26 , 101019. [ Google Scholar ] [ CrossRef ]
- Ju, E.; Li, T.; Liu, Z.; da Silva, S.R.; Wei, S.; Zhang, X.; Wang, X.; Gao, S.-J. Specific inhibition of viral MicroRNAs by carbon dots-mediated delivery of locked nucleic acids for therapy of virus-induced cancer. ACS Nano 2020 , 14 , 476–487. [ Google Scholar ] [ CrossRef ] [ PubMed ]
- Gao, G.; Guo, Q.; Zhi, J. Nanodiamond-based theranostic platform for drug delivery and bioimaging. Small 2019 , 15 , 1902238. [ Google Scholar ] [ CrossRef ] [ PubMed ]
- Perevedentseva, E.; Lin, Y.-C.; Jani, M.; Cheng, C.-L. Biomedical applications of nanodiamonds in imaging and therapy. Nanomedicine 2013 , 8 , 2041–2060. [ Google Scholar ] [ CrossRef ]
- Abate, M.; Lombardi, A.; Luce, A.; Porru, M.; Leonetti, C.; Bocchetti, M.; Campani, V.; De Rosa, G.; Graziano, S.F.; Nele, V. Fluorescent nanodiamonds as innovative delivery systems for MiR-34a replacement in breast cancer. Mol. Ther.-Nucleic Acids 2023 , 33 , 127–141. [ Google Scholar ] [ CrossRef ]
- Masotti, A.; Miller, M.R.; Celluzzi, A.; Rose, L.; Micciulla, F.; Hadoke, P.W.; Bellucci, S.; Caporali, A. Regulation of angiogenesis through the efficient delivery of microRNAs into endothelial cells using polyamine-coated carbon nanotubes. Nanomed. Nanotechnol. Biol. Med. 2016 , 12 , 1511–1522. [ Google Scholar ] [ CrossRef ] [ PubMed ]
- Nekoueian, K.; Amiri, M.; Sillanpää, M.; Marken, F.; Boukherroub, R.; Szunerits, S. Carbon-based quantum particles: An electroanalytical and biomedical perspective. Chem. Soc. Rev. 2019 , 48 , 4281–4316. [ Google Scholar ] [ CrossRef ] [ PubMed ]
- Dong, H.; Dai, W.; Ju, H.; Lu, H.; Wang, S.; Xu, L.; Zhou, S.-F.; Zhang, Y.; Zhang, X. Multifunctional poly (l-lactide)–polyethylene glycol-grafted graphene quantum dots for intracellular microRNA imaging and combined specific-gene-targeting agents delivery for improved therapeutics. ACS Appl. Mater. Interfaces 2015 , 7 , 11015–11023. [ Google Scholar ] [ CrossRef ] [ PubMed ]
- Liu, F.; Ding, N.; Huo, D.; Yang, G.; Wei, K.; Guan, G.; Li, Y.; Yang, J.; Wang, T.; Wang, Y. Surface-Engineered Monocyte Inhibits Atherosclerotic Plaque Destabilization via Graphene Quantum Dot-Mediated MicroRNA Delivery. Adv. Healthc. Mater. 2019 , 8 , 1900386. [ Google Scholar ] [ CrossRef ] [ PubMed ]
- Rupaimoole, R.; Slack, F.J. MicroRNA therapeutics: Towards a new era for the management of cancer and other diseases. Nat. Rev. Drug Discov. 2017 , 16 , 203–222. [ Google Scholar ] [ CrossRef ] [ PubMed ]
- Segal, M.; Slack, F.J. Challenges identifying efficacious miRNA therapeutics for cancer. Expert Opin. Drug Discov. 2020 , 15 , 987–991. [ Google Scholar ] [ CrossRef ] [ PubMed ]
- Fu, Z.; Wang, L.; Li, S.; Chen, F.; Au-Yeung, K.K.-W.; Shi, C. MicroRNA as an important target for anticancer drug development. Front. Pharmacol. 2021 , 12 , 736323. [ Google Scholar ] [ CrossRef ] [ PubMed ]
- Esquela-Kerscher, A.; Trang, P.; Wiggins, J.F.; Patrawala, L.; Cheng, A.; Ford, L.; Weidhaas, J.B.; Brown, D.; Bader, A.G.; Slack, F.J. The let-7 microRNA reduces tumor growth in mouse models of lung cancer. Cell Cycle 2008 , 7 , 759–764. [ Google Scholar ] [ CrossRef ] [ PubMed ]
- Lucas, T.; Schäfer, F.; Müller, P.; Eming, S.A.; Heckel, A.; Dimmeler, S. Light-inducible antimiR-92a as a therapeutic strategy to promote skin repair in healing-impaired diabetic mice. Nat. Commun. 2017 , 8 , 15162. [ Google Scholar ] [ CrossRef ]
- Fu, Y.; Chen, J.; Huang, Z. Recent progress in microRNA-based delivery systems for the treatment of human disease. ExRNA 2019 , 1 , 1–14. [ Google Scholar ] [ CrossRef ]
- Zhang, L.; Hou, D.; Chen, X.; Li, D.; Zhu, L.; Zhang, Y.; Li, J.; Bian, Z.; Liang, X.; Cai, X. Exogenous plant MIR168a specifically targets mammalian LDLRAP1: Evidence of cross-kingdom regulation by microRNA. Cell Res. 2012 , 22 , 107–126. [ Google Scholar ] [ CrossRef ] [ PubMed ]
- Zhou, Z.; Li, X.; Liu, J.; Dong, L.; Chen, Q.; Liu, J.; Kong, H.; Zhang, Q.; Qi, X.; Hou, D.; et al. Honeysuckle-encoded atypical microRNA2911 directly targets influenza A viruses. Cell Res. 2015 , 25 , 39–49. [ Google Scholar ] [ CrossRef ] [ PubMed ]
- Xu, T.; Zhu, Y.; Lin, Z.; Lei, J.; Li, L.; Zhu, W.; Wu, D. Evidence of Cross-Kingdom Gene Regulation by Plant MicroRNAs and Possible Reasons for Inconsistencies. J. Agric. Food Chem. 2024 , 72 , 4564–4573. [ Google Scholar ] [ CrossRef ] [ PubMed ]
- Li, Z.; Lei, Z.X.; Cai, Y.L.; Cheng, D.B.; Sun, T.L. MicroRNA therapeutics and nucleic acid nano-delivery systems in bacterial infection: A review. J. Mater. Chem. B 2023 , 11 , 7804–7833. [ Google Scholar ] [ CrossRef ] [ PubMed ]
- Feliu, N.; Docter, D.; Heine, M.; Del Pino, P.; Ashraf, S.; Kolosnjaj-Tabi, J.; Macchiarini, P.; Nielsen, P.; Alloyeau, D.; Gazeau, F. In vivo degeneration and the fate of inorganic nanoparticles. Chem. Soc. Rev. 2016 , 45 , 2440–2457. [ Google Scholar ] [ CrossRef ] [ PubMed ]
- Scala-Benuzzi, M.L.; Piguillem Palacios, S.V.; Takara, E.A.; Fernández-Baldo, M.A. Biomaterials and biopolymers for the development of biosensors. In Biomaterials-Based Sensors: Recent Advances and Applications ; Springer: Berlin/Heidelberg, Germany, 2023; pp. 3–24. [ Google Scholar ]
- Wang, H. Beneficial medicinal effects and material applications of rose. Heliyon 2024 , 10 , e23530. [ Google Scholar ] [ CrossRef ] [ PubMed ]
- Wang, H. Advantages of animal leather over alternatives and its medical applications. Eur. Polym. J. 2024 , 214 , 113153. [ Google Scholar ] [ CrossRef ]
- Jiang, Z.; Song, Z.; Cao, C.; Yan, M.; Liu, Z.; Cheng, X.; Wang, H.; Wang, Q.; Liu, H.; Chen, S. Multiple natural polymers in drug and gene delivery systems. Curr. Med. Chem. 2024 , 31 , 1691–1715. [ Google Scholar ] [ CrossRef ]
- Kučuk, N.; Primožič, M.; Knez, Ž.; Leitgeb, M. Sustainable biodegradable biopolymer-based nanoparticles for healthcare applications. Int. J. Mol. Sci. 2023 , 24 , 3188. [ Google Scholar ] [ CrossRef ]
- Nazari-Vanani, R.; Azarpira, N.; Heli, H. Development of self-nanoemulsifying drug delivery systems for oil extracts of Citrus aurantium L. blossoms and Rose damascena and evaluation of anticancer properties. J. Drug Deliv. Sci. Technol. 2018 , 47 , 330–336. [ Google Scholar ] [ CrossRef ]
Click here to enlarge figure
Nanotechnology | Advantage or Disadvantage |
---|---|
Inorganic Nanoparticles | |
Carbon-Based Nanoparticles | |
Nanopore Technology |
The statements, opinions and data contained in all publications are solely those of the individual author(s) and contributor(s) and not of MDPI and/or the editor(s). MDPI and/or the editor(s) disclaim responsibility for any injury to people or property resulting from any ideas, methods, instructions or products referred to in the content. |
Share and Cite
Wang, H. A Review of Nanotechnology in microRNA Detection and Drug Delivery. Cells 2024 , 13 , 1277. https://doi.org/10.3390/cells13151277
Wang H. A Review of Nanotechnology in microRNA Detection and Drug Delivery. Cells . 2024; 13(15):1277. https://doi.org/10.3390/cells13151277
Wang, Hsiuying. 2024. "A Review of Nanotechnology in microRNA Detection and Drug Delivery" Cells 13, no. 15: 1277. https://doi.org/10.3390/cells13151277
Article Metrics
Article access statistics, further information, mdpi initiatives, follow mdpi.
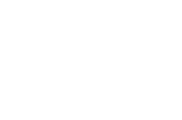
Subscribe to receive issue release notifications and newsletters from MDPI journals

IMAGES
VIDEO
COMMENTS
Nanotechnology is. science, engineering and technology conducted at the. nanoscale, which is about 1 to 100 nm where nano. denotes the scale range of 10-9 and nanotechnology. refers the properties ...
Nanotechnology is now a vital tool in the mechanical. engineering field. Its contributions have led to significant advancements in the areas of nano 3D. printing, performance improvement and ...
Nanoindentation of the microscale honeybee comb reveals a stiffness gradient that spans two orders of magnitude, which amplifies the catapult effect and facilitates solid particle repellency. By ...
a Center of Research Excellence in Desalination & Water Treatment, ... e Department of Mechanical Engineering, King Fahd University of Petroleum and Minerals, ... This review discusses a brief history of nanomaterials and their use throughout history to trigger advances in nanotechnology development. In particular, we describe and define ...
Nanotechnology is a relatively new field of science and technology that studies tiny objects (0.1-100 nm). Due to various positive attributes displayed by the biogenic synthesis of nanoparticles (NPs) such as cost-effectiveness, none to negligible environmental hazards, and biological reduction served as an attractive alternative to its counterpart chemical methods.
Nanotechnology contributes significantly to the growth and even revolutionization of many technical and industrial fields, namely, Electrical, agricultural, medical and electronic technologies, homeland security and food safety, transport and much more. Nanotechnology currently utilizes advances in chemical, mechanics, Materials research and biotechnology for the development of new materials ...
Science, technology, engineering, and mathematics (STEM) education is particularly crucial. Goal 5: Gender Equality: Gender studies and social sciences provide insights into the root causes of gender inequality. Research helps identify barriers and effective interventions to promote gender equality in various sectors. Goal 6: Clean Water and ...
1. INTRODUCTION. The future of nanotechnology with electric vehicles (EVs) is uncertain. Researchers and engineers use nano-manipulating materials to boost EVs' speed, efficiency and longevity [].Nanotechnology makes coatings for EVs, battery technology, energy harvesting, sensors, catalysis and lightweight materials possible [].By enhancing energy storage, charging speed, component weight ...
December 1-3, 2021, Belgrade, Serbia. 51. 9-5 Application of nanomaterials in mechanical engineering. Đ orđe Jovanović1, Mladen Krstić2, Branislav Milenković3. 1 Mathematical institute of ...
Device miniaturization is an emerging advanced technology in the 21st century. The miniaturization of devices in different fields requires production of micro- and nano-scale components. The features of these components range from the sub-micron to a few hundred microns with high tolerance to many engineering materials. These fields mainly include optics, electronics, medicine, bio-technology ...
Abstract. The transcendent technologies include nanotechnology, microelectronics, information technology and biotechnology as well as the enabling and supporting civil infrastructure systems and materials. These technologies are the primary drivers of the 21st century and the new economy. Mechanics and materials are essential elements in all of ...
Micro and Nanotechnology. There's a big future in small things. Nanotechnology is the new frontier of engineering, imagining new possibilities in manufacturing, fluid mechanics, robotics, combustion, biomedicine, measurements, heat transfer, and more. Purdue hosts the largest academic cleanroom in the world, the Birck Nanotechnology Center ...
Micro and Nanotechnology. The micro/nano area enables airbag sensors, disposable medical sensors, rapid DNA analysis, stronger and lighter materials for transportation, and energy-efficient conversion devices, to name a few. Research Includes: Micro/nano-enabled energy and power technology, nanobiotechnology, nano/micro design and manufacturing ...
Nanotechnology is one of the most promising key enabling technologies of the 21st century. The field of nanotechnology was foretold in Richard Feynman's famous 1959 lecture "There's Plenty of Room at the Bottom", and the term was formally defined in 1974 by Norio Taniguchi. Thus, the field is now approaching 50 years of research and application. It is a continuously expanding area of ...
Nanotechnology, contrary to its name, has massively revolutionized industries around the world. This paper predominantly deals with data regarding the applications of nanotechnology in the modernization of several industries. A comprehensive research strategy is adopted to incorporate the latest data driven from major science platforms. Resultantly, a broad-spectrum overview is presented which ...
With an atomic level knowledge of the energy carrier (photon, electron, phoning, and fluid particle) characteristics and behaviors, one is able to prompt upwards to design nano- and micro-structures with the desired size effects, or to synthesize new materials with the desired parts. Research at the Nano Thermo-Physical, Engineering Lab seeks ...
The Applications of Nanotechnology in Mechanical Engineering Mukesh Devpura, Post Guest Faculty, CTAE, Department of Mechanical Engineering, UdaiPura Abstract— Nanotechnology has become an all-embracing term, which means different things to different people. Nanotechnology is interface technologies that are include many different science and applications area.
Abstract. Nanotechnology is science, engineering and technology conducted at the nanoscale, which is about 1 to 100 nm where nano denotes the scale range of 10-9 and nanotechnology refers the ...
Nanotechnology in mechanical engineering and production is extremely beneficial to the industry. Nanotechnology can be utilised to extend the life of car components and parts. Nanotechnology has the potential to improve a wide range of materials. Nanomaterials have distinct physical and chemical features that improve manmade materials.
Advances in Engineering Advances in Engineering features breaking research judged by Advances in Engineering advisory team to be of key importance in the Engineering field. Papers are selected from over 10,000 published each week from most peer reviewed journals.
Active metasurfaces enable dynamic manipulation of the scattered electromagnetic wavefront by spatially varying the phase and amplitude across arrays of subwavelength scatterers, imparting ...
Abstract. Nanotechnology, contrary to its name, has massively revolutionized industries around the world. This paper predominantly deals with data regarding the applications of nanotechnology in the modernization of several industries. A comprehensive research strategy is adopted to incorporate the latest data driven from major science platforms.
The research team developed a system that enables the filtration process without the need for costly vacuum equipment by placing a carbon paper support under the nanofiber membrane, inducing ...
Download Citation | Nanotechnology in mechanical engineering | The textbook describes the theoretical foundations and technological methods of implementation of the unique properties of the ...
a Master Program in Materials Science and Engineering, Faculty of Mechanical and Aerospace Engineering, Institut Teknologi Bandung, Jl. Ganesha 10, ... c Materials Science and Engineering Research Group, Faculty of Mechanical and Aerospace Engineering, ... Paper. Submitted 08 Apr 2024. Accepted 24 Jul 2024. First published 07 Aug 2024. Download ...
In the new study, the researchers applied the material to damaged cartilage in the animals' knee joints. Within just six months, the researchers observed evidence of enhanced repair, including the growth of new cartilage containing the natural biopolymers (collagen II and proteoglycans), which enable pain-free mechanical resilience in joints.
To enhance the undergraduate and graduate engineering education for nanoscale materials, devices and systems, we report a multi-disciplinary course based on the integration of theory, hands-on ...
Feature papers represent the most advanced research with significant potential for high impact in the field. ... This paper reviews the nanotechnology applications for miRNA detection and miRNA drug delivery. ... H.D.; Kim, J.-W.; Bae, J.W.; Hwang, N.S. Nanotechnology in tissue engineering and regenerative medicine. Korean J. Chem. Eng. 2023 ...