Office: 919.785.1304 | Fax: 919.785.1306
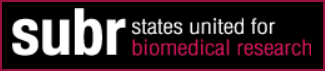
- Donate to SUBR
- Copyright and Privacy Statements
- Why Are Animals Needed in Research?
- What About Using Fewer Animals or No Animals?
- Product and Environmental Safety Research
- What is Biomedical Research?
- Biomedical Research Definitions
- Research Options
- How Animals Help
- Member Organizations

BIOMEDICAL RESEARCH DEFINITIONS
Biomedical research definitions Words used to describe different kinds of biomedical research
Biomedical Research: The area of science devoted to the study of the processes of life, the prevention and treatment of disease, and the genetic and environmental factors related to disease and health.
Basic or “pure” Research : Research conducted to increase the base knowledge and understanding of the physical, chemical, and functional mechanisms of life processes and disease. It is fundamental and not directed to solving any particular biomedical problem in humans or animals. This type of research often involves observing, describing, measuring, and experimental manipulation and provides the building blocks upon which the other types of research (applied and clinical) are based. A basic researcher seeks to add to the store of knowledge about how living things work. A basic researcher’s experiments add pieces to the immensely complex puzzles of life.
Examples of Basic Research: How do nerves convey signals to the brain via biochemicals? How do taste and smell change with age? How does an octopus’s body regenerate a severed tentacle?
Applied Research : Research that is directed towards specific objectives such as the development of a new drug, therapy, or surgical procedure. It involves the application of existing knowledge, much of which is obtained through basic research, to a specific biomedical problem. Applied research can be conducted with animals, nonanimal alternatives such as computer models or tissue cultures, or with humans.
Examples of Applied Research: What drug can be developed to help cure cancer of the skin? Can we “teach” a mouse’s body to regenerate a severed leg?
Clinical Research : Using the knowledge gained in basic and applied research to conduct research (generally with humans) in treating disease or dysfunction in a new way.
Research that takes place in a hospital or clinical setting and is focused on treating specific human and animal diseases and other ailments. Clinical research builds upon the knowledge learned through applied and basic research. Clinical research is conducted on human beings and takes shape in treatments and drugs that directly improve human healthcare.
Examples of Clinical Research: What are the side effects of a specific new drug?
Biological Models System: A system that can be observed instead of the original system, human or animal, that is of ultimate interest to the research.
Researchers use models because they help to answer questions that could not be answered using the original system with the technology and methods that exist. By using a model, researchers increase their ability to isolate and study certain features that would be too complex to study or impossible to isolate in the original system.
Types of Models Used in Biomedical Research: Whole living animals (human and non-human) Living systems composed of samples from the original animal (i.e., tissue culture) Non-living mechanical or molecular systems Mathematical models (i.e., computer simulations)
Why Pursue a PhD in Biomedical Science?
New section.
Information about what one can do with a PhD in Biomedical Science.
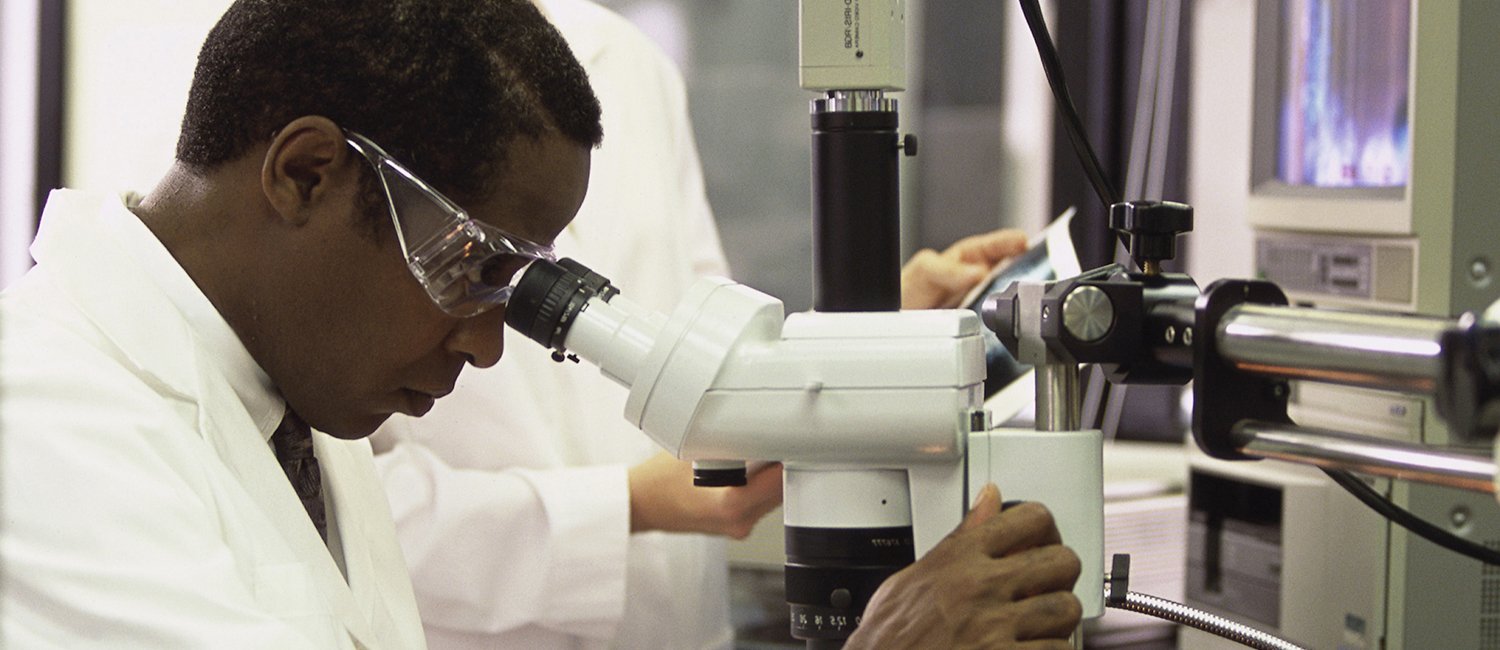
Biomedical Research: What is it?
Biomedical research is the exploration of processes that govern the functioning of molecules, cells, and organisms in health and disease.
Biomedical Scientists: Who are they?
Biomedical scientists bridge the gap between the basic sciences and medicine. The PhD degree is the gateway to a career in biomedical research.
Biomedical scientists:
- Think outside the box and are innovators.
- Are critical and analytical thinkers.
- Get excited by discovering new things.
- Look at biology and see previously unrecognized patterns.
- Enjoy the freedom to pursue interesting questions.
- Have the persistence to a see a project through from small beginnings to great discoveries.
- Want to improve the human condition through their work.
- See the power of biomedical research to change the world.
About This Site
The content on this site was developed by the Aspiring Biomedical Scientists Committee of the AAMC Graduate Research, Education and Training (GREAT) Group . The GREAT Group serves as a national forum to help PhD, MD-PhD, and postdoctoral training programs educate successful biomedical researchers.
- Follow us on Twitter
Helpful tools for those applying to medical PhD programs.
Upcoming short presentations will describe features of PhD training, alumni careers, and detailed logistics of the application process.
Learn about PhD Programs from program leaders.
Graduate schools in the biomedical sciences will generally provide a comprehensive funding package to their students.
PhD Programs by School
List of Postdoctoral Programs by School
Postbaccalaureate programs begin after an undergraduate degree and are designed to support the transition to professional school.
What does a biomedical scientist do?
Would you make a good biomedical scientist? Take our career test and find your match with over 800 careers.
What is a Biomedical Scientist?
Biomedical scientists uses scientific methods to investigate biological processes and diseases that affect humans and animals. They conduct experiments, analyze data, and interpret findings to improve our understanding of diseases and develop new treatments and cures. They also ensure the safety and efficacy of drugs and medical devices through clinical trials and regulatory processes.
The work of biomedical scientists covers a wide range of areas, including genetics, microbiology, immunology, and biochemistry. Various tools and techniques are used to study living organisms at the molecular and cellular levels, such as microscopy, DNA sequencing, and protein analysis. Biomedical scientists often collaborate with other healthcare professionals, such as physicians and nurses, to develop new diagnostics and treatments for diseases.
What does a Biomedical Scientist do?
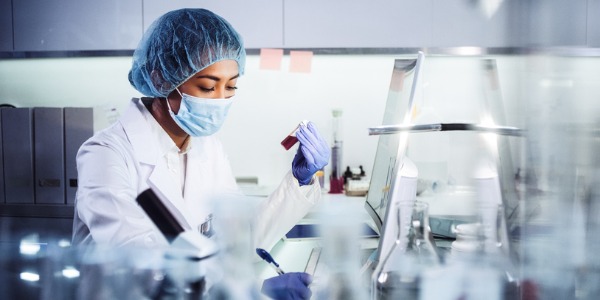
The work of biomedical scientists has a profound impact on human health and has contributed to the development of numerous life-saving medical advances.
Duties and Responsibilities The duties and responsibilities of a biomedical scientist vary depending on their area of specialization and the specific role they play within their organization. However, some common responsibilities of biomedical scientists include:
- Conducting Research: Biomedical scientists design and conduct experiments to investigate biological processes and diseases. They use various laboratory techniques, including microscopy, DNA sequencing, and protein analysis, to study living organisms at the molecular and cellular levels. They collect and analyze data, interpret findings, and communicate results to other scientists and healthcare professionals.
- Developing New Treatments: Biomedical scientists work to develop new drugs, therapies, and medical devices to treat diseases. They conduct preclinical studies to test the safety and efficacy of new treatments, and they work with clinicians to design and conduct clinical trials to evaluate the effectiveness of new treatments in humans.
- Analyzing Samples: Biomedical scientists analyze biological samples, such as blood, tissue, and urine, to diagnose diseases and monitor treatment. They use laboratory techniques to detect and quantify biomarkers, such as proteins and DNA, that are associated with specific diseases.
- Ensuring Quality Control: Biomedical scientists are responsible for ensuring the quality and accuracy of laboratory tests and procedures. They follow established protocols and standard operating procedures, maintain laboratory equipment, and monitor laboratory safety to ensure compliance with regulatory requirements.
- Managing Laboratory Operations: Biomedical scientists may be responsible for managing laboratory operations, including supervising staff, developing and implementing laboratory policies and procedures, and ensuring that laboratory equipment is properly maintained and calibrated.
- Collaborating with Other Healthcare Professionals: Biomedical scientists collaborate with other healthcare professionals, including physicians, nurses, and pharmacists, to develop and implement treatment plans for patients. They communicate laboratory results and provide expert advice on the interpretation of test results.
- Teaching and Mentoring: Biomedical scientists may be responsible for teaching and mentoring students and junior researchers. They may develop and deliver lectures, supervise laboratory activities, and provide guidance and mentorship to students and trainees.
Types of Biomedical Scientists There are several different types of biomedical scientists, each with their own area of specialization and focus. Here are some examples of different types of biomedical scientists and what they do:
- Microbiologists : Microbiologists study microorganisms, including bacteria, viruses, and fungi. They investigate how these organisms cause disease, develop new treatments to combat infections, and develop new diagnostic tests to identify infectious agents.
- Immunologists : Immunologists study the immune system and its role in fighting disease. They investigate how the immune system responds to infectious agents, cancer cells, and other foreign substances, and they develop new treatments that harness the immune system to fight disease.
- Geneticists : Geneticists study genes and their role in disease. They investigate the genetic basis of diseases, such as cancer, and develop new diagnostic tests and treatments that target specific genetic mutations.
- Biochemists : Biochemists study the chemical processes that occur in living organisms. They investigate how cells and tissues produce and use energy, and they develop new drugs and therapies that target specific metabolic pathways.
- Toxicologists : Toxicologists study the effects of toxic substances on the body. They investigate how chemicals, pollutants, and other environmental factors can cause disease, and they develop strategies to prevent and mitigate the harmful effects of toxic exposures.
- Pharmacologists: Pharmacologists study the effects of drugs on the body. They investigate how drugs interact with cells and tissues, and they develop new drugs and therapies to treat disease.
- Medical Laboratory Scientists: Medical laboratory scientists, also known as clinical laboratory scientists, perform laboratory tests on patient samples to diagnose diseases and monitor treatment. They analyze blood, urine, tissue, and other samples using various laboratory techniques and instruments.
What is the workplace of a Biomedical Scientist like?
Biomedical scientists work in diverse settings, contributing to advancements in medical research, healthcare, and the understanding of diseases. The workplace of a biomedical scientist can vary based on their specific role, specialization, and the nature of their work.
Academic and Research Institutions: Many biomedical scientists are employed in universities, medical schools, and research institutions. In these settings, they conduct cutting-edge research, lead laboratory teams, and contribute to scientific discoveries. Academic biomedical scientists often split their time between conducting research, teaching students, and publishing their findings in scientific journals.
Hospitals and Healthcare Settings: Biomedical scientists play a crucial role in healthcare, especially in clinical laboratories and diagnostic facilities. They may be involved in analyzing patient samples, conducting medical tests, and interpreting results to assist in the diagnosis and treatment of diseases. Biomedical scientists working in hospitals collaborate with clinicians and healthcare professionals to ensure accurate and timely diagnostic information.
Biotechnology and Pharmaceutical Companies: The biotechnology and pharmaceutical industries employ biomedical scientists to drive innovation in drug discovery, development, and testing. In these settings, scientists work on designing experiments, conducting preclinical and clinical trials, and developing new therapeutic interventions. Biomedical scientists may also be involved in quality control, ensuring the safety and efficacy of pharmaceutical products.
Government Agencies and Public Health Organizations: Biomedical scientists can work for government agencies such as the National Institutes of Health (NIH), the Centers for Disease Control and Prevention (CDC), or the Food and Drug Administration (FDA). In these roles, they contribute to public health research, policy development, and the regulation of healthcare products.
Nonprofit Research Organizations: Nonprofit organizations dedicated to medical research and public health also employ biomedical scientists. These organizations focus on specific diseases or health issues and work towards finding solutions, advancing knowledge, and advocating for improved healthcare practices.
Private Research Foundations: Biomedical scientists may work for private research foundations that fund and conduct medical research. These foundations often collaborate with academic institutions and industry partners to support innovative research projects with the potential to impact human health.
Collaborative and Interdisciplinary Teams: Biomedical scientists frequently collaborate with professionals from various disciplines, including bioinformaticians, clinicians, engineers, and statisticians. Interdisciplinary collaboration is common, especially in research projects that require a multifaceted approach to address complex health challenges.
Frequently Asked Questions
Biology related careers and degrees.
- Animal Scientist
- Bioinformatics Scientist
- Biomedical Scientist
- Biophysicist
- Biostatistician
- Cellular Biologist
- Comparative Anatomist
- Conservation Biologist
- Developmental Biologist
- Ecology Biologist
- Ecotoxicologist
- Entomologist
- Evolutionary Biologist
- Herpetologist
- Ichthyologist
- Immunologist
- Mammalogist
- Marine Biogeochemist
- Marine Biologist
- Marine Conservationist
- Marine Ecologist
- Marine Fisheries Biologist
- Marine Mammalogist
- Marine Microbiologist
- Microbiologist
- Molecular Biologist
- Neurobiologist
- Ornithologist
- Paleontologist
- Physiologist
- Systems Biologist
- Wildlife Biologist
- Wildlife Ecologist
- Zoo Endocrinologist
Related Degrees
- Animal Sciences
- Biostatistics
- Bioinformatics
- Cellular Biology
- Computational Biology
- Conservation Biology
- Evolutionary Biology
- Marine Biology
- Microbiology
- Molecular Biology
- Neurobiology
Continue reading
Science Related Careers and Degrees
- Anthropologist
- Archaeologist
- Astrophysicist
- Atmospheric Scientist
- Behavioral Scientist
- Biotechnician
- Biotechnologist
- Chemical Technician
- Climate Change Analyst
- Conservation Scientist
- Criminologist
- Cytogenetic Technologist
- Cytotechnologist
- Dairy Scientist
- Engineering Physicist
- Epidemiologist
- Food Science Technologist
- Food Scientist
- Forensic Pathologist
- Forensic Science Technician
- Forensic Scientist
- Geospatial Information Scientist
- Horticulturist
- Hydrologist
- Industrial Ecologist
- Materials Scientist
- Meteorologist
- Natural Sciences Manager
- Neuropsychologist
- Neuroscientist
- Oceanographer
- Particle Physicist
- Pathologist
- Pharmaceutical Scientist
- Political Scientist
- Poultry Scientist
- Social Scientist
- Sociologist
- Soil and Plant Scientist
- Soil and Water Conservationist
- Toxicologist
- Veterinary Pathologist
- Volcanologist
- Biochemistry
- Biomedical Sciences
- Criminology
- Dairy Science
- Environmental Science
- Food Science
- Horticulture
- Political Science
- Poultry Science
- Social Science
- Soil Science
- U.S. Department of Health & Human Services

- Virtual Tour
- Staff Directory
- En Español
You are here
Nih…turning discovery into health ®, the future of biomedicine.
To achieve its goal of turning discovery into health and to maintain its role as the world's premier biomedical research agency, NIH must support the best scientific ideas and brightest scientific minds. That means looking to the future and ensuring that we have a strong and diverse workforce to catalyze discoveries in all fields of biomedicine including emergent areas like data science.
tomorrow-science.jpg
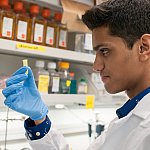
Tomorrow’s Scientists
Part of the NIH mission is supporting the next generation of scientists, funding thousands of graduate students and postdoctoral fellows across the United States.
diversity-nih.jpg
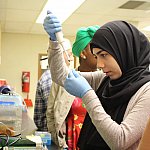
Innovation Through Diversity
Enhancing diversity in the NIH-funded workforce is urgent, given shifting U.S. demographics and the need to draw insights from all corners of America.
« Previous: A Healthy Mind Next: Transformative Technologies »
This page last reviewed on November 16, 2023
Connect with Us
- More Social Media from NIH

- Our Areas of Emphasis
- Our Key Programs
- Our Board Emeritus
- Our Founders
- Our State Affiliates
- Our Event Calendar
- Newsletters
- Our Members
- Value Proposition
- #ThanksResearch
- Annual Awards: Buster & Nancy D. Alvord, and Alvin J. Thompson
- Member Job Postings
- Sponsorship Opportunities
- 2024 IRB CONFERENCE - July 25, 2024
- 2024 IBC, Biomedical Data and IP Security, and IACUC Conferences
- 2023 #ThanksResearch Gala
- 2023 October 19th Communication Workshop
- Camp BIOmed
- Topical Webinars
- Community Events
- Conference and Event Policies
- 2024 Portland Clinical Research Professionals Conference
- 2024 IBC, Biomedical Data & IP Security, and IACUC Conferences
- 2023 Hybrid Communication Workshop
- Ethics Primer
- Bioethics 101
- Introductory Bioinformatics: Genetic Testing
- Advanced Bioinformatics: Genetic Research
- The Nature of Scientific Research
- Animals in Research
- Humans in Research
- Stem Cell Research
- HIV Vaccines
- Consumer Awareness
- For the Greater Good
- Education Strategies
- General Resources
- Student Resources
- Research Resources
- Useful Links
The Biomedical Research Process
What defines biomedical research? How do scientists ensure that new drugs are safe? What factors must be considered before enrolling patients in a clinical trial? How long does it take to develop new drugs? The sites below have been especially selected to address these issues and other ones related to the research process.
Overviews of the Biomedical Research Process
What Exactly is Biomedical Research? Our North Carolina Affiliate, NCABR, has compiled a useful overview explaining what biomedical research is and how it works.
Food and Drug Administration Basic questions and answers, information on protection of human subjects, and overview articles about clinical trials.
The Confusing World of Clinical Trials - Clinical Trial Information Summarizes what a clinical trial is, how trials proceed, and other related topics from the Multiple Sclerosis Association. Written in a very accessible manner.
The MD Anderson Cancer Center – Clinical Trials The MD Anderson Cancer Center at the University of Texas provides a helpful resource for understanding many facets of clinical trials.
The National Cancer Institute The NCI offers a wealth of information on clinical trials. Especially valuable are the Understanding Clinical Trials section and the Resources section. Be sure to check out the many free publications available, as well as the power point presentations on clinical trials available on-line.
Research and Drug Development
Beyond Discovery: The Path from Research to Human Benefit Real life case studies illustrate how recent medical and technological advances arose from basic laboratory research. Some of the areas covered are Hepatitis B, polymers, designer seeds, and human gene testing.
Center for Drug Evaluation and Research at the Food and Drug Administration
© NWABR.ORG 2024
Except where otherwise noted, content on this site is licensed under a Creative Commons Attribution 3.0 License. The Northwest Association for Biomedical Research, NWABR is a 501(c)3 organization.
- Skip to main content
- Skip to "About this site"
- Departments
Language selection
- Search and menus
Biomedical Research
Scenario #1: publishing your research.
You are a PhD student in a lab conducting research on Parkinson’s disease. Your PhD work has produced an interesting finding and your supervisor is eager for you to publish it. You have drafted the manuscript and it is ready for submission to a leading journal in your area. However, you are not happy with one of the digital images that you are submitting with the paper. You are concerned that the results presented in the paper are difficult to observe in the image and discuss the issue with a postdoctoral fellow from your lab. She suggests that you use a photo manipulation software to adjust the background in the image to increase the contrast, which would make the differences easier to observe.
You want to make your lab look good and keep your supervisor happy. You are also aware that your doctoral funding is running out, and you are keen to finish and defend your manuscript-based thesis as soon as possible. You do not want your manuscript to be rejected or delayed because of this one image. Should you use a photo manipulation software to adjust the image?
Discussion questions
- What are the ethical issues at stake in this case?
- What are the potential long-term effects of postponing publication for you, for your lab, and for your field of research?
- When does the beautification of data become falsifying data? Is beautifying data ever acceptable?
Scenario shift
You decide to use a photographic manipulation software to adjust the image and submit it to the journal. Your supervisor informs you that she has just been asked to act as a peer reviewer on a manuscript that shows similar data and draws similar conclusions to your manuscript. Your supervisor says she can delay her peer review for as long as possible, and give very harsh comments and criticisms of the paper in order to delay its publication. This will give you a chance to get your paper published first and avoid being “scooped”.
Additional discussion questions
- What should you/your supervisor do in this situation? How does your decision affect other researchers?
- What are some of the conflicts of interest that are at stake here? For you? For your supervisor?
Relevant ethics guidance documents
- CIHR Open Access Policy
- Committee on Publication Ethics (COPE)
- Uniform Requirements for Manuscripts Submitted to Biomedical Journals: Ethical Considerations in the Conduct and Reporting of Research: Conflicts of Interest
- The Tri-Agency Policy on Research Integrity (2016): Tri-Agency Framework: Responsible Conduct Of Research
Links to the Ethics Cycle conceptual framework
- Publish results (authors listed on papers and agreeing to co-author a paper leads to important ethical consequences);
- Review and select knowledge (publication bias is both a scientific and ethical issue);
- Sustain knowledge use (lost opportunity costs associated with delaying the publication of important findings have ethical implications for the author as well as other researchers).
Link to real life case study
- Image manipulation case studies on COPE
Links to relevant articles
- Dickersin, K., Min, Y.I., & Meinert, C.L. (1992). Factors influencing publication of research results . The Journal of the American Medical Association (JAMA), 267(3), 374.
- Pearn, J. (1995). Publication: An ethical imperative . BMJ, 310(6990), 1313.
- Blumenthal, D., Campbell, E.G., Anderson, M.S., Causino, N., & Louis, K.S. (1997). Withholding research results in academic life science . JAMA, 277(15), 1224.
Discussion Guide
- Ethical issues at stake include: conflict of interest and research integrity (including honesty, trustworthiness, respecting the research endeavor, fairness etc.).
- Long-term effects of postponing publication include: ineffective use/ waste of resources, undermining the research endeavor, delaying research result translation, missed career opportunities, financial hardship.
- Consider issues such as: whether modification omits an important factor/ finding; whether results would be interpreted differently with or without the change; whether modification leads the reader to a substantially different conclusion; accepted practices in your area of research (as defined by journals, professional bodies, etc.).
- You and your supervisor should be aware of the repercussions of such actions on others (including other researchers, the scientific community, funders, and the general public). These repercussions may be detrimental and unfair.
- Your supervisor should consider his or her roles as a peer reviewer and a supervisor/lab manager, and how these roles may result in conflicting interests. Other conflicts include the need/desire to publish prolifically versus research integrity/reporting of results in a timely fashion.
Scenario 2: Modifying Research Questions
You have just received funding from a stem cell research agency and CIHR to conduct induced pluripotent stem (iPS) cell research Footnote 1 without the use of viruses. It is hoped that this research will eventually inform treatments that benefit patients undergoing transplants, among other things. You are aware that ‘Company Science’ will be recruiting next fall and you would really like to work for them after you graduate. You know that they are interested in conducting transplantation research in a few select areas. These are important areas of research, but not the areas that you planned to address in your original research proposal approved by your supervisor and funders. If you add these questions to your original study, it will take longer to complete.
You are pretty sure that you could modify your original research questions or change a few of them altogether in order to address the company’s interests while still completing your research on time. A colleague tells you that it is common for students and postdocs to wander away from the questions and hypotheses in their original research proposals for a variety of reasons. What should you do?
- Is it wrong to change your research proposal after it is approved by your supervisor? Under what circumstances would it be acceptable, if any?
- Do you need to tell your supervisor about the reasons why you want to modify your research proposal? What if this case involved a PI on a project instead of a student? Would that make a difference? Who does a PI need to inform of modifications to a research proposal if anyone?
- In addition to your other funding, what if Company Science had offered you a stipend to conduct transplantation research in their area of concentration. What difference would this make to the case, if any?
You discuss changing your research proposal with your supervisor and she agrees for you to shift the focus of your research study. Your supervisor says that you do not need to discuss these changes with your funder as research proposals often change once put into practice. She explains that research results, design, methods, and populations may change without regard to what the granting agency agreed to fund.
- Is it wrong to change your research proposal after it is approved by your funders?
- How might your decision to change your research impact your funders? Other researchers? Future research studies?
- 2nd Edition of the Tri-Council Policy Statement: Ethical Conduct for Research Involving Humans (TCPS 2)
- Seek funding (this case explores the ethical obligations that a researcher has to their funder concerning the knowledge they create);
- Form research question (how researchers frame their questions impacts stakeholders of the research outcomes as well as other researchers);
- Toward next generation research and/or Toward continued KT (the research conducted (or not conducted) today will inform future research efforts).
- Questions to be considered when determining the acceptability of modifying research direction include: whether the underlying scope of research remains the same; if the research still fulfills university requirements to obtain your degree; whether new research/novel findings suggest a new mode of inquiry is more appropriate/fruitful; if the supervisor can continue to provide adequate advice/input/supervision; supervisors’ (and/or committee members) perspective; and if your choices undermine other obligations (e.g., associated with other funding/awards).
- Other interests, which may result in a conflict of interest, should be clearly outlined, discussed, and managed. Obligations to the supervisor, lab, funder, etc. must all be considered.
- Financial (and other) conflicts of interest are relevant to this case. The potential result of these conflicts should be considered (e.g., behavioural change, allegiances, transparency, influence on result reporting, etc.).
- Issues such as responsibilities to funders, research integrity, and scope of original project should be discussed. It is also important to consider how changes to a research proposal may impact stakeholders.
- The broader effect of changing research direction should be explored. For example, funders may have specific reasons for supporting research in your specific area, and may have concurrently funded other researchers, whose proposals will now overlap, resulting in wastage of resources. It is also important to consider what would happen if all researchers ignored their original research proposals and research questions. If everyone funded to do research about X instead conducted research about Y. Consider the impact on current and future research projects.
- Skip to primary navigation
- Skip to main content
- Skip to footer
Human Research Protection Program
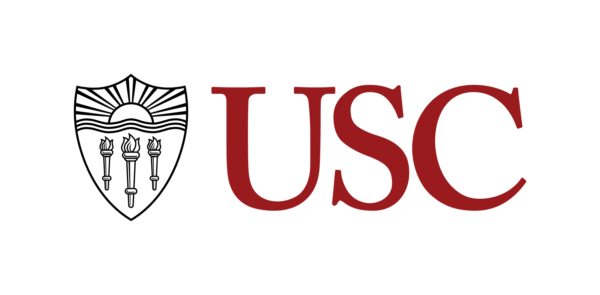
Biomedical Research
What is biomedical research.
Biomedical research is the study of human physiology and the treatment or understanding of disease.
Types and Methods
- research on therapies ( g. , drugs, surgical interventions, or medical devices)
- diagnostic procedures ( g. , imaging biomarkers)
- preventive measures ( g. , vaccines or diet)
- studies of the human body while exercising, fasting, eating, sleeping, or learning
- responding to such things as stress or sensory stimulation
- Studies comparing the functioning of a particular physiological system at different stages of development
- Studies defining normal childhood development so that deviations from normal can be identified
- Medical records and biospecimens research
- Research on specific disease processes to establish and improve methods of prevention, diagnoses, and treatment
- Research on the human genome and genetic markers
Risk is the probability of harm or injury (physical, psychological, social, or economic) occurring as the result of participation in a research study. Biomedical researchers must consider the following risks when conducting their study:
- Social, psychological, or economic harm
- possible side effects of drugs, biologics, or medical devices
- injury from medical procedures
The IRB is responsible for conducting a risk/benefit assessment and will want to know how such risks will be minimized or addressed.
When submitting an application in iStar you will see the following in section 1.5.

Genomic Data Sharing (GDS)/Stem Cell Research
- USC Stem Cell Regenerative Medicine Initiative
- USC Stem Cell Research Oversight Committee (SCRO)
- NIH Genomic Data Sharing Policy (formerly ‘GWAS’)
- California Institute for Regenerative Medicine (CIRM)
- Genetics and Public Policy Center
- NIH: Stem Cell Research
- U.K. Human Fertilisation and Embryological Authority
- The Hinxton Group: An International Consortium on Stem Cells, Ethics and Law
- The International Society for Stem Cell Research (ISSCR)
- Human Genome Project: Educational Kit
3720 S. Flower Street, Suite 325 Los Angeles, CA 90089
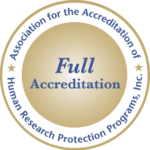
- Announcements
- Getting Started
- Internal Staff Webpage
- Education & Certification
- HRPP Performance & Metrics
- Policies and Procedures
- Post Approval Monitoring (PAM)
- Youtube Channel
- Biospecimen & Data Repositories
- Investigational Drugs and Devices
- Investigator-Initiated Trials
- Emergency Research
- SBIRB Social Behavioral Research
- Student Researchers
- Requesting USC to Rely on an External IRB
- Requesting USC IRB to Act as the sIRB
- Starting a Research Trial: the Basics
- Forms and Templates
- FWA and IRB Registration Numbers
- IRB Member Toolbox
- IRB Review: How to
- IRB Submission Guidelines
- Levels of IRB Review
- Not Human Subjects Research (NHSR)
- Post IRB Review and Approval
- Privacy, Confidentiality, and Anonymity in Human Subjects Research
- Urgent Review
Scientific understanding in biomedical research
- Original Research
- Open access
- Published: 02 August 2024
- Volume 204 , article number 66 , ( 2024 )
Cite this article
You have full access to this open access article
- Somogy Varga ORCID: orcid.org/0000-0001-9383-7843 1 , 2
281 Accesses
Explore all metrics
Motivated by a recent trend that advocates a reassessment of the aim of medical science and clinical practice, this paper investigates the epistemic aims of biomedical research. Drawing on contemporary discussions in epistemology and the philosophy of science, along with a recent study on scurvy, this paper (1) explores the concept of understanding as the aim of scientific inquiry and (2) establishes a framework that will guide the examination of its forms in biomedical research. Using the case of Tuberculosis (TB), (3) it is argued that grasping a mechanistic explanation is crucial for reaching a threshold of understanding at which we may speak of an objectual, biomedical understanding of TB.
Similar content being viewed by others
Analytic Philosophy for Biomedical Research: The Imperative of Applying Yesterday’s Timeless Messages to Today’s Impasses
The Empirical Turn in Bioethics – From Boundary Work to a Context-Sensitive, Transdisciplinary Field of Inquiry
Objectivity, Scientificity, and the Dualist Epistemology of Medicine
Avoid common mistakes on your manuscript.
Within just a few years, multiple editorials in prominent medical journals have issued a call to reflect on the aim of clinical medicine and medical science. Focusing on the latter matter, in a 2013 editorial, the editors of The Lancet clarified that they frequently confront not only queries about the rationale behind specific scientific studies but also broader inquiries regarding the overarching aim of medical science, which includes both clinical and medical laboratory research. They contend that the moment has come to rethink our approach to conducting and incentivizing research, and for this, “we need to remind ourselves about the real purpose of research” (The Lancet, 2013 , p. 347; see also Thornton, 2013 ). The authors express concern that a significant portion of the vast sums allocated annually to biomedical research fails to meet its true objectives. This shortfall is attributed not only to deficiencies in research design and methodology but also to a lack of “clinical meaningfulness.” Specifically, they highlight that many research projects pose questions that are not sufficiently aligned with clinical medicine and relevant to the treatment, control, prevention, or prediction of diseases. The authors note that the issues extend beyond merely reducing the potential impact of biomedical research; they suggest a fundamental misunderstanding of the very purpose of biomedical research, implying that such studies may not truly qualify as medical . Footnote 1
This short Lancet piece highlights significant, yet often overlooked, questions concerning the epistemic aim of medical research. This paper will address these questions, positing that medical science is fundamentally engaged in inquiries aimed at achieving what we shall refer to as biomedical understanding . To investigate and clarify what such understanding amounts to, the paper takes inspiration from two sources. On the one hand, it draws on contemporary discussions in philosophy of science and epistemology, which have seen a renewed interest in understanding as a distinct cognitive accomplishment (Grimm, 2021 ; Baumberger et al., 2017 ), as the epistemic aim of scientific inquiry, and the measure of progress (see e.g., Potochnik, 2015 ; De Regt & Dieks, 2005 ; Elgin, 2017 ). Acknowledging that what constitutes proper understanding can depend on the field, as noted by scholars in the field (Strevens, 2010 ; De Regt et al., 2009 ), this paper aims to specifically articulate what understanding entails within medical science. On the other hand, this paper draws on and employs several distinctions from a recent study on scurvy (Varga, 2023 ). However, while that study focused on a noncommunicable condition stemming from severe dietary deficiencies, this paper shifts our focus to Tuberculosis (TB), a multifaceted and emblematic infectious disease often accompanied by stigma (WHO, 2023 ). TB, which is one of the oldest known infectious diseases, is caused by the bacterium Mycobacterium tuberculosis (Kapur et al., 1994 ; Daniel, 2006 ). The bacteria are transmitted when an infected individual coughs, sneezes, or speaks, allowing another person to breathe in the pathogens. Symptoms of TB can include coughing, chest pain, fatigue, fever, and night sweats and although the condition is treatable with antibiotics it remains a major global health concern.
The paper is organized as follows. It (1) explores the idea of understanding as the aim of scientific inquiry and (2) lays down a framework of understanding that will subsequently guide our exploration of its forms in medicine. Using the case of Tuberculosis (TB), (3) it is argued that grasping a mechanistic explanation is crucial for reaching a threshold of understanding at which we may speak of an objectual, biomedical understanding of TB. If evidence can be gathered to support this argument, it would align with the previously mentioned research on a noncommunicable disease (scurvy), suggesting a recurring pattern across various contexts of medical research.
1 The aim of scientific inquiry: constitutive aim and truth
Scientific inquiries can be viewed as extensions of our day-to-day endeavors to gather information albeit executed in a more systematic manner (see e.g., Kelp, 2021 ). They are goal-directed activities, implying that there is some aim that inquiry strives to accomplish. It is quite natural to assume that this description also fits medical science; however, before delving into the question of what constitutes the epistemic aim of medical science, it is crucial to first briefly clarify what medical science refers to. What sets medical science apart and qualifies something as specifically medical science, rather than just science in general?
Medical science, which includes clinical research and laboratory research in medicine, is fundamentally based on the life sciences. Over the past two centuries, it has extensively leveraged discoveries in biology that have identified cellular, genetic, and molecular entities and processes that help explain the development and course of diseases. While some aspects of medical science may not differ essentially from laboratory sciences within contributing disciplines such as biology, biochemistry, and physiology, medical science cannot simply be reduced to the sum of these fields. One reason is that medical research is only deemed properly medical when it has a specific practical orientation—that is, when it is driven by the goal of contributing to clinical medicine, which primarily focuses on the diagnosis, prevention, and treatment of disease. Without this practical focus, research might be more accurately described as biological rather than medical. Take, for example, large-scale laboratory research that aims to chart the functions of specific limbic structures in the brain. Without a practical focus on clinical applications or health outcomes, such research might be more accurately described as neurobiological rather than medical. Of course, this research could potentially yield benefits for clinical medicine in the future, but without a direct and immediate practical orientation, it would not be classified as medical research. Moreover, if we were to classify such research as medical merely based on potential future benefits, the distinction between medical and non-medical research would collapse.
Of course, this practical orientation toward health outcomes is a characteristic that biomedical research shares with related fields such as public health. However, their epistemic aims are directed towards different objectives: biomedical research typically focuses on the biological and physiological aspects of diseases at a molecular or cellular level, aiming to elucidate disease mechanisms and develop new treatments, whereas public health is primarily concerned with improving the health of populations through prevention strategies, health education, surveillance, and improving access to health care. Public health aims encompass a wide array of objectives: ensuring safe environments by controlling hazards in air, water, and food, enhancing host resistance through balanced nutrition and immunization, promoting health-supportive behaviors, and improving equitable access to health and social services (White et al., 2013 ; Munthe, 2008 ). Footnote 2
Although both fields are dedicated to conducting research with the final aim to improve health outcomes, they operate with different priorities and methodologies, each aligned with their distinct epistemic goals. A biomedical researcher might delve into the genetic factors that contribute to the resistance of TB to antibiotics, focusing on molecular and cellular details. In contrast, public health initiatives may not require such knowledge; instead, they often concentrate on understanding societal or other health factors that hinder the implementation of vaccination programs or public campaigns aimed at increasing awareness and prevention of TB.
Having briefly clarified at least some of the aspects that set medical science apart, we can now turn to the question of its aim. As we begin to explore this, it is worth considering how plausible it is to claim that scientific inquiries in medicine are driven by a single aim. This consideration is crucial because the diversity of methods, approaches, and priorities within medical science suggests that its objectives might not be unified under a single overarching aim. In response, it is important to clarify that for the purposes of this paper, we do not assert that medical science is driven by a single aim. Instead, among potentially other aims, our objective is to explore the nature of medical science’s epistemic aim , which also determines what counts as progress at least in this limited sense. Thus, very roughly, if A is the aim of inquiry, then medical science makes progress when A accumulates or increases (for a discussion, see Bird, 2007 ; Varga, 2024 ).
So what is the epistemic aim of scientific inquiry in medicine? According to a plausible suggestion, the aim is simply to discover truths about health and disease and correct past errors (e.g., false beliefs about diseases like scurvy or depression being caused by humoral imbalance) that were based on tradition, cognitive errors, ideologies, or religious dogma. Correspondingly, progress consists in a cumulative acquisition of true beliefs. For example, until the nineteenth century, the prevailing belief was that TB was inherited or caused by environmental factors such as bad air or poor living conditions. But already in 1720, the English physician Benjamin Marten hypothesized that TB and its symptomatic lesions in the lungs are caused by “species of Animalcula or wonderfully minute living Creatures” that can be transmitted “by very frequently conversing so nearly as to draw inpart of the breath he emits from the lungs” (cited in Cambau & Drancourt, 2014 ; Daniel, 2006 ). Supporting this hypothesis, in 1865, the French physician Jean-Antoine Villemin provided experimental evidence that TB could be transmitted. He observed that TB was more prevalent in people living close and in poorly ventilated buildings, and he noted that while TB was common among troops in barracks, it decreased during military campaigns when soldiers were not housed (Daniel et al., 1994 ). Thus, Marten and Villemin unearthed truths regarding TB, rectified previous mistakes, and aided in the ongoing accumulation of accurate beliefs, which constitutes progress.
On its face, the suggestion that the aim of medical research is simply to discover truths is plausible. After all, it is often said that scientific inquiries are in the “truth business” (Pennock, 2019 ; Lipton, 2004 ), and it is difficult to imagine that contemporary medical science would be able to achieve what it does if its claims would not at least roughly correspond to how the world actually is. Nonetheless, the acquisition of true beliefs does not seem sufficient to constitute progress. Footnote 3 Take, for instance, a scenario where Marten and Villemin arrived at the same conclusion through unreliable methods and, coincidentally, the theory they came to accept happened to be true. In that case, Marten and Villemin would have acquired a true belief, but it would not have counted as genuine progress. What would be lacking is suitable justification for holding the relevant belief. In other words, the belief that they would have acquired would not qualify as knowledge .
1.1 Knowledge and understanding
What we learn from these considerations is that progress not only requires that our beliefs and theories be true but that we have attained adequate reasons for forming them. If this is correct, then it seems safe to conclude that the aim of inquiry is not merely truth, but knowledge (achieved by reliable means), which would mean that progress consists in the increase not of true beliefs, but of knowledge. Although this correction marks an improvement, it is necessary to supply some clarifications and caveats.
First, the aim of inquiry cannot simply be the mere accumulation of knowledge. Medical science has an expansive range of questions at its disposal, and it could potentially attain a vast pool of knowledge, but much of this potential knowledge might be trivial or inconsequential, lacking the impact or significance to be deemed progress. Imagine that researchers could come to know everything about some minor and transient symptom (e.g., a slight, transient change in nail coloration or longitudinal nail ridging) observed in a small subset of TB patients that are known not to have bearing on the disease’s diagnosis, progression, or response to treatment. While detailed knowledge of these symptoms might add to the clinical descriptions of TB, the reason this gained knowledge is not considered significant or constitutive of true progress likely stems from its limited impact on key areas of TB research and clinical management. It lacks the potential to advance our understanding of TB (or indeed other medically relevant conditions), uncover new treatment targets, enhance diagnostic methods, or deepen our understanding of disease transmission and resistance mechanisms.
If we accept this line of reasoning, then the aim of inquiry in medicine cannot be simply to amass knowledge, but rather a selective process that prioritizes the acquisition of certain kinds of significant knowledge. Hence, part of the scientific endeavor involves a critical evaluation process to identify which pieces of knowledge are significant and worth pursuing. This selection process is fundamental to progress, ensuring that scientific efforts are directed toward areas of genuine importance and potential impact (Kitcher, 2001 ; Dupré, 2016 ). Identifying and focusing on significant knowledge, therefore, becomes a crucial aspect of the scientific method, guiding researchers in making meaningful advancements rather than merely expanding the repository of human knowledge.
While the aim of inquiry is significant knowledge, the selection process to identify which pieces of knowledge count as significant cannot be extracted from nature and is largely relative to specific interests. As Kitcher ( 2001 , 61) stated regarding scientific inquiry in general, “significant science must be understood in the context of a particular group with particular practical interests and a particular history”. In the context of TB, it is far more plausible to suggest that what constitutes significant knowledge is closely interwoven with practical concerns related to the understanding and treatment of TB.
Having discussed the issue of significance, we are now faced with a final challenge that questions the notion that the goal of inquiry in medical science is best described as the pursuit of knowledge. In recent years, numerous philosophers of science have contended that framing the aim of inquiry in terms of understanding offers significant benefits over viewing progress merely as an accumulation of knowledge. The advantage with comprehending progress in terms of increased understanding is that it avoids the challenges faced by accounts measuring scientific progress in terms of knowledge (see e.g., Elgin, 2007 , 2017 ; De Regt & Dieks, 2005 ; Potochnik, 2017 ). Footnote 4 First, traditional accounts have problems explaining the significance of certain pragmatic virtues (e.g., simplicity) that do not affect the truth of claims, theories, and explanations. In contrast, an account of progress based on the notion of understanding does not face this problem, as these pragmatic virtues clearly affect the ability to understand (Dellsén, 2016 ). Second, traditional accounts of progress as knowledge accumulation have problems explaining abstractions, approximations, and idealizations. For example, in medicine, physiological accounts often offer idealized and simplified descriptions of organs and their functions (Ereshefsky, 2009 ). These provide computational tractability and improve understanding, but they also include aspects that are, strictly taken, inaccurate or false. However, such falsehoods are, as Elgin ( 2017 ) puts it, “felicitous”: although they involve false representations, they also exemplify significant aspects of phenomena in a tractable manner. Several philosophers have argued that science can increase understanding and contribute to progress even if it involves departing from the truth (e.g., Elgin, 2009a , b ; Strevens, 2017 ; Potochnik, 2015 ).
On an account of progress in terms of knowledge, the presence of manifest falsehoods seems incompatible with progress. However, an account of progress in terms of understanding fares better here, since understanding is compatible with a limited number of falsehoods, which are outweighed by practical advantages. Strevens argues that idealized models can provide understanding, but in a somewhat more limited way, showing why some causal factors are difference-makers and others are not (Strevens, 2017 ). Potochnik ( 2017 , 102; 2015 ) holds that while idealizations cannot be true or approximately true, they can be epistemically acceptable. Because such idealizations are rampant in science and they always detract from the truth, truth does not seem to be a good candidate for describing the aim of science. However, given that idealizations can support understanding, it is more adequate to suppose that understanding is what science aims at.
The latter is not susceptible to such worries, because, in contradistinction to knowledge, understanding is only quasi-factive: it can survive false beliefs if they are not absolutely vital to the phenomenon in question. For example, Marten hypothesized that TB was caused by “species of Animalcula or wonderfully minute living Creatures” (Doetsch, 1978 ; Daniel et al., 1994 ). Strictly taken, this is false: TB was not caused by such small creatures, but by the Mycobacterium tuberculosis bacteria, which Marten had no knowledge of. Nevertheless, it is hard to deny that some progress occurred and an increase in the (objectual) understanding of TB had been obtained.
In all, as opposed to truth or knowledge, the epistemic aim of scientific inquiry is best comprehended as understanding. Comprehending progress in terms of increased understanding dovetails more closely with the pragmatic nature of medicine and has the advantage of being resistant to some of the problems that haunt accounts that comprehend progress as knowledge accumulation. If the epistemic goal of inquiry is best framed as seeking understanding, this raises questions about what understanding is in medical research. The following sections will initially delve into theories of understanding, followed by an examination of the specific nature of understanding within the realm of medicine.
2 Forms of understanding
The debates on understanding have focused on three types of understanding: propositional understanding (understanding that something is the case), explanatory understanding (understanding why something is the case), and objectual understanding (understanding a particular topic or subject matter) (see e.g., Kvanvig, 2003 ; Hannon, 2021 ; Grimm, 2021 ). Footnote 5 In what follows, we are going to be focusing on explanatory and objectual understanding, in part because propositional understanding is often largely reducible to propositional knowledge or explanatory understanding. For example, saying “he understands that he needs to come to TB screening” could amount to the attribution of propositional knowledge (“he knows that he needs to come to TB screening”) or to explanatory understanding (“he understands why it is important for him to come to TB screening”). Of course, there are many other examples of how the term “understanding” is used. But many of them are either reducible to claims about knowledge, objectual understanding or explanatory understanding. For example, when we say that a person really understands how x works, then we are attributing to this person some degree of objectual understanding of x.
To illustrate the difference between knowledge and understanding, consider the example of TB. A student of medicine may attend a lecture on infectious diseases and come to know from a reliable source that TB is caused by Mycobacterium tuberculosis. Accepting the testimony from a reliable source and even double checking it in an encyclopedia of infectious diseases, the student gains causal knowledge. But while the student now knows a proposition that picks out the cause of TB, that is not enough for explanatory understanding, which not only requires knowledge of what caused the effect, but also grasping how that cause brings about the effect (Kvanvig, 2003 ; Pritchard, 2010a ), which many take to involves a type of “skill” (see e.g., De Regt, 2017 ). Understanding does not only require the possession of a theory or model, but also the skill or ability to use it to discern the causal relationship involved. One way to comprehend the difference is that unless explanatory understanding about how cause and effect are related is attained, she will be unable to address what-if-things-had-been-different questions or predict the outcomes of potential interventions (Grimm, 2011 ).
For another example, consider an utterly false theory leading to correct results. Charles Locock’s mid-19th century discovery of the anticonvulsant effect of potassium bromide. Locock, a physician working in London, shared the widely accepted theory among his contemporaries of a causal relationship between masturbation, convulsions, and epilepsy (Ban, 2006 ). As bromides were known to reduce the sex drive, Locock reasoned that the ingestion of potassium bromides would control convulsions by reducing the rate of masturbation. His account of the drug’s effectiveness was published in The Lancet in 1857, and subsequent independent studies confirmed potassium bromide’s antiepileptic efficacy, albeit evidently not by reducing masturbation frequency. Through observations and inference to the best explanation, Locock had attained knowledge that potassium bromide reduced convulsions, and such knowledge allowed the introduction of a relatively effective antiepileptic treatment into medical practice.
Still, in an important sense, such causal knowledge does not properly close the inquiry, which would require grasping a correct explanation and attaining understanding of what happens and how cause and effect are related. Locock did not understand why potassium bromide was effective, why it failed to be effective in some people, and so on. This meant that he lacked the ability to improve the efficiency of the intervention, since he was unable to counterfactually anticipate the effects of changes he could have made with respect to the treatment. More precisely, the lack of understanding means that Locock was unable to (i) predict the changes that would occur if the factors cited as explanatory were different and (ii) to draw correct inferences about similar situations under slightly varied conditions.
2.1 Explanatory and objectual understanding
Objectual and explanatory understanding differ in several ways (Kvanvig, 2003 , 2009 ; Hannon, 2019 , 2021 ). Explanatory understanding involves grasping why something is the case (e.g., uncovering the causal mechanisms or reasons behind phenomena) and its scope is less expansive than that of objectual understanding (Hannon, 2021 ). Objectual understanding, usually expressed using the verb “understands”, followed by a noun, as in the phrase “she understands TB”, entails a comprehensive grasp of a particular topic or subject matter, which includes incorporating these causal explanations into a broader context. While explanatory understanding is often necessary, it is not sufficient for objectual understanding, which requires integrating these explanatory insights within a larger framework.
To illustrate the difference, imagine that our student has now acquired knowledge of a vast number of isolated facts about TB, such that her peers would not hesitate to say that she has knowledge about TB. Nonetheless, this would not imply that the student understands TB, which would attribute to the student a more profound penetration of TB, a sort of epistemic acquaintance that is more profound than knowing particular propositions (Kvanvig, 2003 , p. 191; Strevens, 2017 ). Her objectual understanding of TB is gradable and can always become more profound along various dimensions (Bengson, 2017 ).
Often, achieving (full) objectual understanding is the aim of inquiry, and reaching it justifiably concludes the investigation of the topic (Kvanvig, 2013 ). If we think of medical research, objectual understanding seems to better capture the primary aim of inquiry and the conditions under which it can be concluded. To take the example of TB, researchers not only want to understand why it arises or why certain characteristic biochemical reactions occur but also why it leads to the characteristic symptoms, why it has varied effects on individuals, how it relates to other conditions, and so on. Even though single research projects cannot take on such a large task, the ultimate goal seems to go beyond obtaining explanatory understanding of features of TB to systematically understanding TB , which means attaining some level of coherence and completeness in terms of knowledge, as well as in taxonomies and classifications.
A prevalent perspective posits that achieving objectual understanding marks the endpoint of inquiry and legitimately closes the investigation into the subject (Kvanvig, 2013 ; Carter and Gordon 2014). This perspective aligns well with medicine, where an objectual understanding of a condition, rather than just its explanation, is often the ultimate aim. In their pursuit of understanding TB, researchers aim to grasp not just its origins, but also its manifestations, correlations with other conditions, its varied effects on individuals, and the most useful systematic categorization of its characteristic symptoms and signs.
Some argue that objectual understanding is not merely a subset of explanatory understanding, in part because it is possible to achieve objectual understanding of indeterministic systems where explanatory relations do not obtain (Kvanvig, 2009 ). But even if this turns out to be false (see e.g., Khalifa, 2013 , ch. 4), maintaining this distinction conserves the intuition that when we attribute to somebody objectual understanding of a subject matter (as opposed to explanatory understanding), we imply that the agent’s epistemic commitments relevant to the subject matter form a coherent network. Also, the distinction upholds the idea that objectual understanding’s factivity requirement is more lenient, making it less susceptible to peripheral falsehoods compared to explanatory understanding (see e.g., Elgin, 2017 ; Bamberger, Beisbart, & Brun 2017; Kvanvig, 2009 ).
2.2 Grasping explanations and context-dependency
Both explanatory and objectual understanding go beyond mere knowledge by encompassing an additional cognitive achievement, often referred to as a form of “grasping” (e.g., de Regt, 2009 ; Strevens, 2017 ; Grimm, 2014 ; Elgin, 2017 ; for a critique, see Khalifa, 2013 , ch. 3). The objects of grasping are “explanatory and other coherence-making relationships” (Kvanvig, 2003 , p. 192). There is no clear agreement on the precise meaning of “grasping” (Hannon, 2019 ), but for our purposes we might conceptualize it as a form of cognitive control that agents develop through the active engagement of their epistemic agency in delineating conceptual and explanatory links. Importantly, while grasping enables agents to mentally map a relational assembly (Grimm, 2014 ), it is not reducible to the experience of understanding (e.g., an “aha” moment): good explanations do not necessarily trigger a sense of understanding, while inadequate explanations sometimes do (Trout, 2002 ). While philosophers commonly concur that what is being grasped are explanations, aligning with the notion that the primary purpose of scientific explanation is to foster understanding (Lipton, 2001 ), opinions differ on what kind of explanations lead to understanding, such as deductive-nomological explanations (Hempel & Oppenheim, 1948 ), or mechanistic explanations, which explain phenomena by specifying the mechanisms that produce them (Salmon, 1984 ; Machamer et al., 2000 ). Footnote 6
Importantly, what counts as understanding, is – at least in a limited sense – context-sensitive . This can be interpreted in several ways. First, some argue that understanding is context-sensitive in the sense that the criteria for understanding can evolve even within a single scientific discipline (for historical examples, see De Regt, 2017 ; De Regt et al., 2009 ). This is in part because the capacity of an explanation to lead to understanding is partially contingent upon the disciplinary background and knowledge of individuals seeking to understand.
Second, and more importantly for our aims here, some hold that context-sensitivity is linked to the nature and aim of the particular scientific inquiry. For example, Craver ( 2013 ; Kendler et al., 2011 ) contends that mechanistic explanations are inherently contextual and “perspectival”, as they are framed within a specific explanatory framework that is chosen based on explanatory interests. While this point may be limited to mechanistic explanations, there are indications that objectual understanding displays some context-sensitivity across scientific fields. To illustrate this with a medical example, consider the study of cholesterol metabolism in medical science and chemistry. In medical science, a significant level of objectual understanding of cholesterol metabolism arguably encompasses an understanding of how cholesterol levels are regulated (e.g., by diet, genetics) and how they can be modified through interventions or lifestyle changes to reduce the risk of disease. From the perspective of chemistry, objectual understanding of cholesterol metabolism does not necessarily relate to cardiovascular health but instead focuses on explaining the biochemical pathways of cholesterol breakdown and synthesis, elucidating the precise molecular interactions involved. Thus, what constitutes some sufficient level of objectual understanding in medicine might differ from that in chemistry, primarily because the explanatory goals and interests in medicine are intrinsically tied to practical applications and clinical medicine. There is no inherent tension between context-sensitivity and objectual understanding: even if the threshold for sufficient objectual understanding can be consistent across disciplines, the kinds of explanations needed to reach this understanding vary according to the specific context and the explanatory, practical and other goals of each field.
3 Biomedical understanding
While the presented account of understanding does not purport to capture the intricacies of philosophical debates on the topic, it serves as a basis for exploring what it means to possess objectual understanding of a disease within the medical field. This will be referred to as biomedical understanding (see Varga, 2023 , 2024 ). To grasp what biomedical understanding entails, let us revisit the history of TB research.
Before the 19th century, tuberculosis (TB) was thought to result from heredity or environmental causes like bad air. Marten’s initial hypothesis that “minute living creatures” could spread TB was later validated by Villemin, who in 1865 provided experimental evidence of TB’s transmissibility. He linked its higher incidence to crowded, inadequately ventilated environments and noted a decrease in TB cases among soldiers when they were not confined to cramped barracks (Daniel et al., 1994 ; Bynum, 2012 ). Moreover, by removing liquid from tuberculous cavities of individuals who had died of TB and injecting it into healthy animals, Villemin successfully transmitted the disease from humans to rabbits, from cows to rabbits, and from rabbits to rabbits. Throughout his studies, he used the same amount of liquid and animals of similar origin, age, and habitat conditions, such that “everything indeed other than inoculation, were identical” (Villemin 1868/2015 , 257). While not all animals developed symptoms, autopsies three months later revealed that the vast majority developed extensive TB with massive dissemination of tubercles to the organs (Villemin 1868/2015 ; Barnes, 2000 ).
Clearly, Villemin’s findings helped distinguish between variables that had a direct effect on the development of TB and those that were correlated with it (e.g., certain professions, poverty, poor living conditions). However, while Villemin attained an important piece of explanatory understanding, it would be unwarranted to say that he obtained objectual understanding of TB in any noteworthy sense. Given that the explanatory goals and interests in medicine are closely tied to practical applications, such a claim might seem excessive because the explanatory understanding Villemin obtained did not form a coherent network that would have allowed him to consider how possible medical interventions could limit control the progression and spread of TB. After all, Villemin did not understand under what conditions TB developed, how it transmitted, and what the agent of the disease was, except that the tubercle (nodular lesion) contained it.
Let us now look closer at some shortcomings that could have prevented him from attaining objectual understanding of TB in any substantial sense. The first shortcoming stems from an incomplete understanding of the causal agent. Villemin lacked comprehension with respect to two critical aspects of the causal connection: stability and specificity (see Woodward, 2010 ). A causal link between the injected substance and TB is considered stable if the counterfactual dependence remains consistent across various background situations. Villemin’s studies did not provide much evidence with respect to stability, because they did not involve testing under different background circumstances. In addition, specificity refers to the grain level of counterfactual dependencies between the inoculated substance and TB. Because Villemin inoculated the same amount of substance in each case, his studies offered no knowledge about the extent to which the intensity of tuberculization depends on the amount of substance inoculated. Villemin had no way of determining whether the counterfactual dependencies between the inoculated substance and TB are fine-grained, in which case intervention on the inoculated substance would enable more precise control over how TB develops.
Moreover, Villemin’s incomplete understanding of the causal agent prevented him from ruling out the possibility that experimentally induced tuberculosis might follow a different pathway from ordinary TB or could even be a distinct disease altogether. When injecting liquids from organisms that succumbed from TB, one could argue that the effects obtained were not due to TB, but to the injection containing some “cadaveric material.” Although Villemin could show that the number and extent of lesions on the lungs are not correlated with the number and extent of lesions developed at the injection site, he himself noted a crucial limitation: “should we consider the entire chain of phenomena observed in experimental tuberculosis as the result of a traumatism due to inoculation? This is an enigma that we cannot resolve” (Villemin 1868/2015 , 259).
The second shortcoming concerns a lack of knowledge about the relevant mechanism. The causal knowledge Villemin attained did not permit “tracing” the causal process (Steel, 2008 ), which would have assisted grasping coherence-making relationships and comprehending how the elements of TB are configured. This seems to necessitate some degree of explanatory understanding and discerning the mechanism that is responsible for linking cause and effect. A mechanism for phenomenon P consists of parts and processes that are structured in a way such that they are responsible for P (Glennan et al., 2021 ). Explanations in the biomedical sciences are most frequently mechanistic, explaining a disease by identifying the spatiotemporal structure of a mechanism that is responsible for that disease and its symptoms (Thagard, 2005 ; Darrason, 2018 ; Williamson, 2019 ). Villemin’s study establishes a coarse-grained difference-making relationship, but it does not amount to biomedical understanding because it fails to discern the correct mechanism.
We could say that the lack of such a mechanism has crucially impacted Villemin’s ability to gather sufficient evidence for explanatory understanding. There are two possibilities here, depending on which thesis one subscribes to regarding the role of mechanisms in establishing causal claims (for discussions, see Russo & Williamson, 2007 ; Illari, 2011 ; Williamson, 2019 ). According to a strong thesis, establishing a causal relationship requires not only difference-making evidence but also evidence of a mechanism composed by entities (such as proteins) and processes (such as protein expression) that together link cause and effect. If one accepts the strong thesis, then Villemin has not met the criteria for establishing a causal relationship because he had no knowledge of the mechanism. According to a weaker thesis, difference-making can serve as evidence for a causal relationship. However, evidence of a mechanism, combined with difference-making evidence, significantly increases certainty that the observed correlation is not merely spurious and that the effect can be attributed to the experimental intervention rather than to confounding variables.
Having examined these two shortcomings, it appears likely that each has contributed to the failure to attain objectual understanding. However, it is unclear whether any of these factors are essential for achieving objectual understanding. In the sections that follow, we will explore the historical development of tuberculosis research to further investigate this issue.
3.1 Koch and beyond
A significant breakthrough with respect to the first two shortcomings came with Robert Koch’s 1882 discovery of the bacterium Mycobacterium tuberculosis (MTB) as the causative agent of TB (Keshavjee & Farmer, 2012 ). Footnote 7 Koch formalized a set of “postulates” for establishing causation, which required (a) coincidence of bacteria and disease, (b) isolation of bacteria in a pure culture, and (c) induction of disease by inoculation with bacteria from pure culture. As to (a), Koch was able to show that the MTB were always present in TB (but not in normal states), that they preceded tubercle formation, and that their number covaried with TB being progressive or quiescent. As to (b), Koch managed to isolate individual colonies of MTB in pure culture that allowed studying their growth characteristics. As to (c), he inoculated animals with MTB obtained from various origins (induced disease, spontaneous disease, and artificial culture). Koch found that injections led to the formation of tubercles with similar characteristics, and the number of tubercles corresponded to the amount of the inoculum used (Blevins & Bronze, 2010 ).
While Koch’s postulates can be interpreted in various ways (e.g., Broadbent, 2009 ), some have argued that Koch’s experimental distinction of causal from correlational relationships are best captured by the interventionist account of causation (Ross & Woodward, 2016 ). Interventionism posits that causal relationships are those that can be potentially harnessed for manipulation and control: very roughly, if intervening on C reliably leads to changes in E, then C is the cause of E. Woodward ( 2003 ) outlines the necessary and sufficient criteria for establishing causation as follows: C causes E if and only if (i) there is some possible intervention on C such that (ii) were this intervention to occur, there would be an association or correlation between C and E. The account highlights idealized experimental intervention as appropriate for the purposes of determining whether C causes E, as it eliminates possibility of confounding. As the induced change is not correlated with potential confounders, the presence of a correlation between C and E upon intervention on C means that C has a causal influence on E.
Interventionism fits Koch’s postulates, particularly his emphasis on (c), i.e., the induction of disease into a healthy animal by inoculation with bacteria from pure culture. In fact, Koch clearly maintains that determining causality between MTB and TB “can only be decided by inoculating pure bacilli,” thus step (c) (quoted in Ross & Woodward, 2016 , p. 44). Footnote 8 Of course, (b) can be seen as a procedure to ensure that (c) obtains the characteristics of a proper intervention: it excludes the possibility that confounding factors are contained in the inoculated material. Causal claims can only be established if the intervention is associated with a change in the incidence of TB (e.g., its presence, absence, rate of occurrence). In accordance with (M), if the inoculation of substances had not led to the occurrence of disease, Koch would not have identified them as the cause of the disease.
Although the discovery of the causal agent addressed the first shortcoming in Villemin’s research, it alone was insufficient to resolve the second shortcoming concerning the mechanism. However, this is clearly a significant issue, in part because it connects with important questions from a clinical perspective. Without an understanding of the mechanism, questions about what holds together the symptoms of TB, whether certain characteristics (e.g., diarrhea) are parts of TB or caused by TB, how MTB is disseminated to other organs, why most individuals with latent infection do not develop the disease, cannot be answered.

3.2 Twentieth-century discoveries
In the twentieth century, a notable breakthrough came with the identification of the mechanism through which MTB interacts intricately with the host’s immune system, leading to TB. Roughly, when MTB reaches the lungs, it is taken up by macrophages, which are immune cells that engulf and destroy foreign particles. However, MTB is able to survive and replicate within the macrophages, which leads to the formation of granulomas that surround the infected macrophages to contain the infection. MTB is sometimes able to resist destruction and containment, eventually causing the macrophages to burst and release more bacteria into the surrounding tissue. The infected tissue becomes inflamed, leading to the formation of the characteristic lesions, or granulomas, in the lungs and other organs. The granulomas can restrict the infection, leading to a latent TB infection, or they can break down, releasing MTB into the lungs, where it can be coughed up and spread to others (for reviews, see Delogu et al., 2013 ; Yan et al., 2022 ).
The mechanism was elucidated over several decades through the significant contributions of numerous researchers. Therefore, it is challenging to pinpoint exactly when and by which researchers a threshold was crossed, marking a stage at which we may speak of researchers having attained objectual understanding of TB. However, once a mechanistic explanation became available that referenced the configuration and activities of component entities, and identified both the normal functioning of macrophages and how MTB disrupts this process, it seems quite intuitive to say that researchers had achieved a significant level of objectual, biomedical understanding of TB. Researchers have progressed beyond merely explaining various aspects of TB; they have crossed a threshold into systematically, objectually understanding TB .
Of course, while this assertion may seem intuitively appealing, it alone raises a crucial question: what is it about mechanistic explanations that renders them necessary for achieving a significant level of objectual understanding? In what follows, the aim will be to show that mechanistic explanations have enabled achieving a level of coherence and integration, offering clear potential to refine theoretical frameworks and clinical practices, and to facilitate the development of more comprehensive taxonomies and classifications. But before doing so, it is worth emphasizing that a sufficient level of objectual biomedical understanding of TB has been achieved, not merely by grasping the relevant mechanistic explanations, but also by integrating this with other pieces of knowledge and understanding already obtained.
For this, we may start by noting how a mechanistic explanation not only overcomes the second shortcoming observed in the research of Villemin and Koch but also enables new insights that carry profound implications for diagnosis, treatment, and prevention strategies, directly affecting patient care and public health initiatives. This underscores an earlier argument that what constitutes a sufficient level of objectual understanding in medicine is context-sensitive and closely linked to a practical orientation. Let us now review a couple of important implications for research and clinical settings.
First, grasping the relevant mechanistic explanation, researchers were able to chart a much more fine-grained intricate web of counterfactual dependencies, which paves the road towards enhanced intervention possibilities concerning TB. Researchers can formulate new hypotheses around potential interventions, such as enhancing the macrophages’ capability to eradicate MTB or inhibiting MTB’s ability to prevent acidification within macrophages (for a review of current research, see e.g., Bo et al., 2023 ).
Second, comprehending the mechanism significantly enhances the ability to interpret and address a range of clinically relevant issues. It provides a unified view of TB, clarifying how its various elements are interrelated, and explaining how seemingly disparate symptoms are interconnected through a common cause. This comprehensive insight into the relationships between TB symptoms and the disease process improves diagnostic accuracy and aids in refining diagnostic criteria. It enables healthcare providers to more effectively differentiate TB from other conditions with similar symptoms, thereby reducing the risk of misdiagnosis. Moreover, this understanding is crucial in explaining why some individuals with latent TB infections do not progress to active disease, a key factor in managing public health risks.
Overall, comprehending the mechanism of TB has facilitated a significant milestone, crossing a threshold into what we may describe as an objectual, biomedical understanding of TB. This had key implications for identifying new treatment targets, enhancing diagnostic methods, and deepening our knowledge of disease transmission and resistance mechanisms—all of which are vital for improving clinical interventions and formulating effective public health strategies. Crossing this threshold is an important milestone, but it is entirely consistent with recognizing that further exploration and deeper understanding may still be necessary. It does not in any way imply that researchers have reached a final stage in their inquiry that would conclude investigation into TB. Indeed, as researcher recognize, many questions remain (for a recent review, see e.g., Bloom, 2023 ; WHO, 2023 ), driving increasingly detailed and nuanced insights to continuously refine existing approaches to treatment and prevention.
4 Concluding remarks
In light of the recent calls to reexamine the foundational aims of medicine, both in research and clinical practice, this paper emphasizes the importance of understanding as a unifying aim in these domains. As underscored by recent editorials cited in the introduction, there is an imperative to revisit not only the practical aims that medicine seeks, but also its epistemic aims. This is particularly salient in a time when the very essence of what constitutes medical science and clinical medicine is under scrutiny. Accordingly, this paper concentrated on the relevant epistemic aims. By exploring different forms of understanding, the paper uses TB as a focal point to argue that a grasp of mechanistic explanations is crucial for reaching a threshold of understanding at which we may speak of an objectual understanding of TB.
An important limitation of this paper is its focus on a single case: TB. Consequently, there are notable constraints on the breadth of conclusions that can be drawn. However, there are at least some reasons to believe that the findings may have broader applicability. One such reason is that an earlier study on noncommunicable diseases (Varga, 2023 ) have reached similar conclusion. That study revealed that in the case of scurvy, a mechanistic explanation of the condition is necessary for biomedical understanding, but this is not sufficient for understanding in a clinical setting. This earlier study, which examined an emblematic noncommunicable disease, reached a similar conclusion to the current study that focuses on a representative communicable disease. This suggests a potential pattern across various contexts of biomedical research. That said, additional research is required to reinforce this point by investigating whether these conclusions are applicable across a wide spectrum of diseases, including those that are rarer and less prominent. Additionally, it is worth noting that this might differ significantly for conditions where mechanistic explanations have proven challenging to establish. Mental disorders could serve as critical test cases to explore the applicability of our findings in contexts where the underlying mechanisms are less understood.
Interestingly, in an editorial published by the British Medical Journal (Marshall et al., 2018 ) the editors prompt a similar reflection on the purpose of clinical medicine. They challenge the prevailing emphasis on disease-centric care and encourage contemplation of whether a holistic therapeutic relationship with patients might better align with the true aim of medical practice. Though published separately, these editorials collectively highlight a growing movement towards a critical reevaluation of the aims and priorities of both medical science and clinical medicine. The question has sparked considerable interest, with various competing accounts proposing that there is a single, overarching aim (e.g., Broadbent, 2019 ) whereas others suggesting that medicine has multiple aims (e.g., Boorse, 2016 ; Brody & Miller, 1998 ; Schramme, 2017 ).
Munthe ( 2008 ) advocates for an integrated, multidimensional model, highlighting that recent decades have seen the introduction of new objectives focusing on autonomy and equality.
See Bird ( 2019 ) for a helpful discussion of an example from physics.
Other accounts maintain that progress in science occurs when theories come nearer to the truth or when it accumulates solutions to scientific puzzles that are neutral about questions of truth. For a critical review, see Bird ( 2007 ).
Practical understanding (“understanding-how”) typically involves skillful behaviors, relies often on non-propositional knowledge, and is neither explanatory nor susceptible to Gettier-style objections (Bengson, 2017 ). For example, a person may lack the resources to explain the workings of a device but may understand how the device works by way of her skill to adeptly use it.
A mechanism is typically defined as “a structure performing a function in virtue of its component parts, component operations, and their organization” (Bechtel & Abrahamsen, 2005 , p. 423).
For his research, Koch earned the Nobel Prize in 1905.
It makes sense to think that had Koch adhered to a view of causation as merely regularities involving necessary and sufficient conditions that could be discerned through observation, he would not have emphasized (c).
Ban, T. A. (2006). The role of serendipity in drug discovery. Dialogues in Clinical Neuroscience , 8:3 , 335–344.
Article Google Scholar
Barnes, D. S. (2000). Historical perspectives on the etiology of tuberculosis. Microbes and Infection , 2 (4), 431–440.
Baumberger, C., Beisbart, C., & Brun, G. (2017). What is understanding? An overview of recent debates in epistemology and philosophy of science. In Grimm, S., Bamberger, C, and Ammon, S. (Ed.). (2017). Explaining understanding: New perspectives from epistemology and philosophy of science London: Routledge. 1–34.
Bechtel, W., & Abrahamsen, A. (2005). Explanation: A mechanist alternative. Studies in History and Philosophy of Science Part C: Studies in History and Philosophy of Biological and Biomedical Sciences , 36 (2), 4214–4241.
Bengson, J. (2017). The unity of understanding. In S. Grimm (Ed.), Making sense of the world: New essays on the philosophy of understanding (pp. 14–53). Oxford University Press.
Bird, A. (2007). What Is Scientific Progress? Nous , 41(1), 64–89.
Bird, A. (2019). The aim of belief and the aim of science. Theoria: An International Journal for Theory History and Foundations of Science , 34 (2), 1711–1793.
Google Scholar
Blevins, S. M., & Bronze, M. S. (2010). Robert Koch and the ‘golden age’ of bacteriology. International Journal of Infectious Diseases , 14 (9), e744–e751.
Bloom, B. R. (2023). A half-century of research on tuberculosis: Successes and challenges. Journal of Experimental Medicine , 220 (9), e20230859.
Bo, H., Moure, U. A. E., Yang, Y., Pan, J., Li, L., Wang, M., & Cui, H. (2023). Mycobacterium tuberculosis-macrophage interaction: Molecular updates. Frontiers in Cellular and Infection Microbiology , 13 , 1062963.
Boorse, C. (2016). Goals of Medicine. In É. Giroux (Ed.), Naturalism in the Philosophy of Health (pp. 145–177). Springer International Publishing Carter and Gordon 2014).
Broadbent, A. (2009). Causation and models of disease in epidemiology. Studies in History and Philosophy of Science Part C: Studies in History and Philosophy of Biological and Biomedical Sciences , 40 (4), 302–311.
Broadbent, A. (2019). Philosophy of medicine . Oxford University Press.
Brody, H., & Miller, F. G. (1998). The internal morality of medicine: Explication and application to managed care. The Journal of Medicine and Philosophy , 23 (4), 384–410.
Bynum, H. (2012). Spitting blood: The history of tuberculosis . OUP Oxford.
Cambau, E., & Drancourt, M. (2014). Steps towards the discovery of Mycobacterium tuberculosis by Robert Koch, 1882. Clinical Microbiology and Infection , 20 (3), 196–201.
Craver, C. F. (2013). Functions and mechanisms: A perspectivalist view. Functions: Selection and mechanisms (pp. 133–158). Springer Netherlands.
Daniel, T. M. (2006). The history of tuberculosis. Respiratory Medicine , 100 (11), 1862–1870.
Daniel, T. M., Bates, J. H., & Downes, K. A. (1994). History of tuberculosis. In T. M. Daniel (Ed.), Tuberculosis: Pathogenesis, Protection, and control (pp. 13–24). American Society for Microbiology.
Darrason, M. (2018). Mechanistic and topological explanations in medicine: The case of medical genetics and network medicine. Synthese , 195 (1), 147–173.
De Regt, H. W. (2009). The epistemic value of understanding. Philosophy of Science , 76 (5), 585–597.
De Regt, H. W. (2017). Understanding scientific understanding . Oxford University Press.
De Regt, H. W., & Dieks, D. (2005). A contextual approach to scientific understanding. Synthese , 144 (1), 137–170.
De Regt, H. W., Leonelli, S., & Eigner, K. (2009). Focusing on scientific understanding. In, De Regt, H. W., Leonelli, S., and Eigner, K. (2009) Scientific understanding: Philosophical perspectives , Pittsburgh: University of Pittsburgh Press. 1–17.
Dellsén, F. (2016). Scientific progress: Knowledge versus understanding. Studies in History and Philosophy of Science Part A , 56 , 72–83.
Delogu, G., Sali, M., & Fadda, G. (2013). The biology of mycobacterium tuberculosis infection. Mediterranean Journal of Hematology and Infectious Diseases , 5 (1).
Doetsch, R. N. (1978). Benjamin Marten and his new theory of consumptions. Microbiological Reviews , 42 (3), 521–528.
Dupré, J. (2016). Towarda political philosophy of science. In M. Couch (Ed.), The philosophy of Philip Kitcher . Oxford University Press.
Elgin, C. (2007). Understanding and the facts. Philosophical Studies , 132 (1), 334–332.
Elgin, C. (2009a). Is understanding factive? In A. Haddock, A. Millar, & D. Pritchard (Eds.), Epistemic value (pp. 3223–3230). Oxford University Press.
Elgin, C. (2009b). Exemplification, idealization, and understanding. In M. Suárez (Ed.), Fictions in Science: Essays on idealization and modelling (pp. 779–770). Routledge.
Elgin, C. Z. (2017). True enough . MIT Press.
Ereshefsky, M. (2009). Defining ‘health’ and ‘disease’. Studies in History and Philosophy of Science Part C: Studies in History and Philosophy of Biological and Biomedical Sciences , 40 (3), 221–227.
Glennan, S., Illari, P., & Weber, E. (2021). Six theses on mechanisms and mechanistic science. Journal for General Philosophy of Science , 1–19.
Grimm, S. (2011). Understanding In S. Bernecker and D. Pritchard (Eds.), The Routledge Companion to Epistemology. New York: Routledge.
Grimm, S. (2014). Understanding as knowledge of causes. In A. Fairweather (Ed.), Virtue Epistemology Naturalized: Bridges between Virtue Epistemology and Philosophy of Science . Springer.
Grimm, S. (2021). Understanding, The Stanford Encyclopedia of Philosophy (Summer 2021 Edition), Edward N. Zalta (Ed.), https://plato.stanford.edu/archives/sum2021/entries/understanding/ .
Hannon, M. (2019). What’s the point of knowledge? A function-first Epistemology . Oxford University Press.
Hannon, M. (2021). Recent work in the epistemology of understanding. American Philosophical Quarterly , 58 (3), 269–290.
Hempel, C. G., & Oppenheim, P. (1948). Studies in the logic of explanation. Philosophy of Science , 15 (2), 1351–1375.
Illari, P., & McKay (2011). Disambiguating the russo–Williamson Thesis. International Studies in the Philosophy of Science , 25 , 139–157.
Kapur, V., Whittam, T. S., & Musser, J. M. (1994). Is Mycobacterium tuberculosis 15000 years old? Journal of Infectious Diseases , 170 , 1348–1349.
Kelp, C. (2021). Inquiry, knowledge and understanding. Synthese , 198 (7), 1583–1593.
Kendler, K. S., Zachar, P., & Craver, C. (2011). What kinds of things are Psychiatric disorders? Psychological Medicine , 41 (6), 1143–1115.
Keshavjee, S., & Farmer, P. E. (2012). Tuberculosis, drug resistance, and the history of modern medicine. New England Journal of Medicine , 367 (10), 931–936.
Khalifa, K. (2013). The role of explanation in understanding. The British Journal for the Philosophy of Science , 64 (1), 161–187.
Kitcher, P. (2001). Science, truth, and democracy . Oxford University Press.
Kvanvig, J. L. (2003). The value of knowledge and the pursuit of understanding . Cambridge University Press.
Kvanvig, J. (2009). The value of understanding. In A. Haddock, A. Millar, & D. Pritchard (Eds.), Epistemic value (pp. 95–111). Oxford University Press.
Kvanvig, J. (2013). Curiosity and the response-dependent special value of understanding. Knowledge, virtue and action: Putting epistemic virtues to work, 151–174.
Lipton, P. (2001). What good is an explanation? In G. Hon, & S. S. Rakover (Eds.), Explanation: Theoretical approaches and applications (pp. 53–59). Springer Science and Business Media.
Lipton, P. (2004). Inference to the best explanation . Routledge.
Machamer, P., Darden, L., & Craver, C. F. (2000). Thinking about mechanisms. Philosophy of Science , 67 (1), 12–15.
Marshall, M., Cornwell, J., & Collins, A. (2018). Rethinking medicine. bmj , 363 .
Munthe, C. (2008). The goals of publichealth: An integrated, multidimensional model. Public Health Ethics , 1 (1), 39–52.
Pennock, R. T. (2019). An instinct for truth: Curiosity and the moral character of science . MIT Press.
Potochnik, A. (2015). The diverse aims of science. Studies in History and Philosophy of Science Part A , 53 , 71–80.
Potochnik, A. (2017). Idealization and the aims of Science . University of Chicago Press.
Pritchard, D. (2010a). Knowledge and understanding., in Pritchard, D., Millar, A., and Haddock, A. (2010), The Nature and Value of. Knowledge: Three Investigations. Oxford: Oxford University Press
Ross, L. N., & Woodward, J. F. (2016). Koch’s postulates: An interventionist perspective. Studies in History and Philosophy of Science Part C: Studies in History and Philosophy of Biological and Biomedical Sciences , 59 , 35–46.
Russo, F., & Williamson, J. (2007). Interpreting causality in the Health sciences. International Studies in the Philosophy of Science , 21 , 157–170.
Salmon, W. C. (1984). Scientific explanation and the causal structure of the world . Princeton University Press.
Schramme, T. (2017). Goals of Medicine. In T. Schramme, & S. Edwards (Eds.), Handbook of the philosophy of Medicine (pp. 121–128). Springer Netherlands.
Steel, D. (2008). Across the boundaries, Extrapolation in Biology and Social Science . Oxford University Press.
Strevens, M. (2010). Varieties of Understanding. In: Pacific Division meeting of the American Philosophical Association , San Francisco, CA, March (Vol. 31).
Strevens, M. (2017). How idealizations provide understanding. In S. R. Grimm (Ed.), Explaining understanding: New perspectives from epistemology and philosophy of science . Routledge, Taylor and Francis Group.
Thagard, P. (2005). What is a medical theory? Studies in Multidisciplinarity , 3 , 476–472.
The Lancet. (2013). What is the purpose of medical research? The Lancet , 381 (9864), 347.
Thornton, H. (2013). We need to askwhat is the purpose of research? BMJ , 347 .
Trout, J. D. (2002). Scientific explanation and the sense of understanding. Philosophy of Science , 69 (2), 2122–2133.
Varga, S. (2023). Understanding in Medicine. Erkenntnis . https://doi.org/10.1007/s10670-023-00665-8 .
Varga, S. (2024). Science, Medicine, and the aims of Inquiry: A philosophical analysis . Cambridge University Press.
Villemin, D. J. (1868/2015). On the virulence and specificity of tuberculosis. The International Journal of Tuberculosis and Lung Disease , 19 (3), 256–266.
White, F., Stallones, L., & Last, J. (2013). History, aims, and Methods of Public Health. In F. White, L. Stallones, & J. M. Last (Eds.), Global public health: Ecological foundations . Oxford University Press.
WHO. (2023). Global tuberculosis report 2023 . World Health Organization.
Williamson, J. (2019). Establishing Causal claims in Medicine. International Studies in the Philosophy of Science , 32 (1), 33–61.
Woodward, J. (2003). Making things happen: A theory of causal explanation . Oxford University Press.
Woodward, J. (2010). Causation in Biology: Stability, specificity, and the choice of levels of explanation. Biology and Philosophy , 25 (3), 287–318.
Yan, W., Zheng, Y., Dou, C., Zhang, G., Arnaout, T., & Cheng, W. (2022). The pathogenic mechanism of Mycobacterium tuberculosis: Implication for new drug development. Molecular Biomedicine , 3 (1), 48.
Download references
Open access funding provided by Aarhus Universitet.
Open access funding provided by Aarhus Universitet
Author information
Authors and affiliations.
Department of Philosophy and History of Ideas, Aarhus University, Aarhus, Denmark
Somogy Varga
The Centre for Philosophy of Epidemiology, Medicine and Public Health, University of Johannesburg, Johannesburg, South Africa
You can also search for this author in PubMed Google Scholar
Corresponding author
Correspondence to Somogy Varga .
Additional information
Publisher’s note.
Springer Nature remains neutral with regard to jurisdictional claims in published maps and institutional affiliations.
Rights and permissions
Open Access This article is licensed under a Creative Commons Attribution 4.0 International License, which permits use, sharing, adaptation, distribution and reproduction in any medium or format, as long as you give appropriate credit to the original author(s) and the source, provide a link to the Creative Commons licence, and indicate if changes were made. The images or other third party material in this article are included in the article’s Creative Commons licence, unless indicated otherwise in a credit line to the material. If material is not included in the article’s Creative Commons licence and your intended use is not permitted by statutory regulation or exceeds the permitted use, you will need to obtain permission directly from the copyright holder. To view a copy of this licence, visit http://creativecommons.org/licenses/by/4.0/ .
Reprints and permissions
About this article
Varga, S. Scientific understanding in biomedical research. Synthese 204 , 66 (2024). https://doi.org/10.1007/s11229-024-04694-w
Download citation
Received : 12 October 2023
Accepted : 28 June 2024
Published : 02 August 2024
DOI : https://doi.org/10.1007/s11229-024-04694-w
Share this article
Anyone you share the following link with will be able to read this content:
Sorry, a shareable link is not currently available for this article.
Provided by the Springer Nature SharedIt content-sharing initiative
- Understanding
- Biomedical research
- Tuberculosis
- Clinical medicine
- Find a journal
- Publish with us
- Track your research
38 Scientists Helping Chart the Future of Biomedical Research
Pew’s 2022 scholars and fellows selected to explore the intricacies of health and medicine.
Navigate to:
- Table of Contents
Biomedical researchers are at the forefront of scientific innovation, seeking answers to the world’s most pressing questions in human health. For 37 years, The Pew Charitable Trusts has encouraged these pursuits by supporting promising, early-career biomedical scientists tackling these challenges and driving medical breakthroughs.
This year, a total of 38 researchers join the Pew Scholars Program in the Biomedical Sciences , Pew Latin American Fellows Program in the Biomedical Sciences , and Pew-Stewart Scholars Program for Cancer Research . They will receive multiyear grants to pursue scientific interests in the United States and Latin America at a time when biomedical science has never been more critical.
Infectious disease prevention and treatment
The COVID-19 pandemic put a spotlight on the immune system in the fight against infectious diseases.
Several members of the 2022 class are studying the intricacies of this essential defense mechanism. One scientist will explore how bacterial exposure changes the body’s circadian rhythm—the cellular clock regulating activities such as sleeping and eating—and influences immune system function, particularly in how the body fights infections. Another will investigate how parents pass on disease-fighting knowledge to their children through what is known as “immune memory,” a process that can enhance their offsprings’ responses when exposed to the same pathogen. And another scientist will study how structural changes in cell surface molecules drive white blood cells to curb infections.
A separate group will investigate how to prevent and treat certain diseases. One scientist will study how immune cells respond to viral hemorrhagic fevers, while another will examine how Ebola enters host cells. Others will explore a new, promising class of antibiotics that targets the production of essential bacterial proteins, as well as a potential “universal vaccine” for rapidly mutating viruses such as HIV, SARS-CoV-2, and influenza.
Wonders of the brain
From walking and talking to sleeping and socializing, the brain is critical to the human body’s most essential tasks. But much is still unknown about how this complex organ coordinates with other bodily systems.
Scientists in this year’s class will explore how the brain and gut work together to ensure that the body has needed nutrition, and also which neurological processes are at play when individuals experience physical pain. One researcher will use schooling fish to explore how the brain processes visual information to inform movement, while another will seek to determine how neurons detect pheromones to decipher social engagement cues.
Because the brain controls functions from learning and memory to social behaviors, disturbances to the neural system can cause dire consequences. Some scientists will investigate mechanisms that contribute to neuronal dysfunction and potential strategies to keep this from occurring. For example, one researcher will explore how memory-encoding cells that are activated by negative experiences affect the development of Alzheimer’s disease. Another will examine how mutations in enzymes involved in protein clearance disrupt neuronal function and survival.
Decoding cancer development
Cancers develop when abnormal cells proliferate uncontrollably and spread throughout the body unchecked. The 2022 class is studying different elements of this complex disease—exploring mechanisms that drive cancer initiation and novel strategies to control its progression.
Cancer cells are highly reliant on the metabolic process for the energy they need to grow and spread. One researcher will examine the dependence of cancer cells on the lysosome—a specialized cellular structure—to fuel their demand for nutrients. Another will build a novel system to study how cancer cells adapt to new metabolic pathways to evade therapy. And one is looking at the compounds produced in the breakdown of nutrients used by acute lymphoblastic leukemia cells for clues about how treatment resistance mechanisms arise.
Researchers also are studying different aspects of the immune system response to cancer. One class member is examining how sensory neurons regulate an immune response to lung cancer, while another is investigating the effect a new immunotherapy has on T-cells and their ability to attack skin cancer.
Finally, a group will explore the role of genetic variations in cancer. Members of the 2022 class will study how mutations in a cell receptor that binds sugars contributes to the formation of aggressive cancers and also how accumulation of mutations promotes the risk for blood cancer development. Another researcher will look at how genetic variation can help protect us from cancer—using wolves in the Chernobyl Exclusion Zone, surrounding the failed nuclear power plant, as a novel case study.
Unraveling the human genome
The genome is a tightly packed set of instructions that determines everything from peoples’ physical traits to their behavioral tendencies. Careful regulation is needed to maintain the integrity of our genetic material and prevent a myriad of human diseases.
Members of the 2022 class are studying mechanisms that regulate proper gene expression. One will examine how DNA structural rearrangements are coordinated, while another will study how some novel and overlooked structures within the genome contribute to the maintenance of our genetic code. And one researcher will examine specialized genome sequences to understand how variations of gene products arise.
Researchers are also investigating the cellular machines that help accurately distribute and separate chromosomes during cell division, as well as how these specialized complexes ensure that DNA in the mitochondria—the powerhouses of the cell—is replicated with fidelity. Finally, some scientists are studying how disruptions or defects in proteins that help safeguard and maintain the genome contribute to the development of blood and bone cancers.
Intestinal health and disease
The gut is home to trillions of bacteria, viruses, and fungi that coexist in a community, also known as the microbiome. Some components of the microbiome are present at birth, but exposure to environmental elements and diet as we age diversifies the makeup of this community.
One class member will explore how different lipids in human milk are processed by gut bacteria to promote health in babies. Another will examine how early antibiotic exposure can disrupt the composition of the microbiome and how these changes may contribute to weight gain later in life.
Gut health can be influenced by other factors as well. A member of the class will explore how brain signals may impact intestinal inflammation. Another scientist will investigate how factors such as age can alter nutrient transport by intestinal cells and lead to the development of metabolic and intestinal disturbances in the elderly.
Kara Coleman is the project director and Jennifer Villa is an officer with The Pew Charitable Trusts’ biomedical programs.
Don’t miss our latest facts, findings, and survey results in The Rundown
ADDITIONAL RESOURCES

Trust Magazine
More from pew.

- Why We Need Biomedical Research
- Putting It in Perspective
- Benefits of Animal Research
- Why Are Animals Needed
- Animal Rights vs. Animal Welfare
- Why is Product Safety Needed
- What is Laboratory Animal Science and the 3 Rs?
- Brochures and Other Outreach Tools
- "Celebrate Animals in Research and Education"
- Branch POE Award
- Ann Turner CARE Academy
- Careers in LAS
- AALAS Foundation Legacy Society
- Planned Giving Program
- AREA Program
- Corporate Contributors
- Branch Contributors
- Individual Contributors
- Memorial Wall
- Public Outreach
- About Animal Research
Biomedical research is the broad area of science that looks for ways to prevent and treat diseases that cause illness and death in people and in animals. This general field of research includes many areas of both the life and physical sciences. Utilizing biotechnology techniques, biomedical researchers study biological processes and diseases with the ultimate goal of developing effective treatments and cures. Biomedical research is an evolutionary process requiring careful experimentation by many scientists, including biologists and chemists. Discovery of new medicines and therapies requires careful scientific experimentation, development, and evaluation. -New Jersey Association for Biomedical Research . Every person in the United States has benefited from the results of biomedical research. From the development of new drugs, vaccines, or procedures to prevent or treat diseases; to the safety testing of products we use every day of our lives; scientists strive to better understand the causes and treatments of disease. Through the similarities between humans and laboratory animals, we have learned much about our bodies and how they work. We continue to learn more and are now able to apply that knowledge not only to humans, but to our pets, wildlife, and other animals. Due to medical research, life expectancy in the United States has improved dramatically, from an average of 49 years in 1900 to 69.3 years in 2004 (World Health Organization). By 2030, projections suggest one in five Americans will be 65 or older, and the number of people aged 85 and older—currently the fastest growing segment of the older population—could exceed 10 million (National Institute on Aging). The increase in the number of older Americans is mostly due medical advances that have been based on animal research.
The AALAS Foundation supports educational outreach on the essential role of responsible laboratory animal care and use in science to advance human and animal health.
Latest News
- $25 on the 25th Jan 11, 2024
- 2023 AALAS Foundation Appreciation Reception and Live Auction Sep 06, 2023
- 2023 Celebrate the Mouse Video Essay Contest Aug 29, 2023
Useful Links
- News Archive
© American Association for Laboratory Animal Science Foundation. All Rights Reserved. Privacy Policy | Disclaimer | Copyright

An official website of the United States government
The .gov means it’s official. Federal government websites often end in .gov or .mil. Before sharing sensitive information, make sure you’re on a federal government site.
The site is secure. The https:// ensures that you are connecting to the official website and that any information you provide is encrypted and transmitted securely.
- Publications
- Account settings
Preview improvements coming to the PMC website in October 2024. Learn More or Try it out now .
- Advanced Search
- Journal List
Categorizing biomedical research: the basics of translation
Jeffrey s. flier.
* Department of Neurobiology, Harvard Medical School, Boston, Massachusetts, USA
† Department of Medicine, Harvard Medical School, Boston, Massachusetts, USA;
‡ Department of Medicine, Beth Israel Deaconess Medical Center, Boston, Massachusetts, USA; and
Joseph Loscalzo
§ Department of Medicine, Brigham and Women’s Hospital, Boston, Massachusetts, USA
As biomedical research has evolved over the past century, the terminology employed to categorize it has failed to evolve in parallel to accommodate the implications of these changes. In particular, the terms basic research and translational research as used today in biomedicine seem especially problematic. Here we review the origins of these terms, analyze some of the conceptual confusions attendant to their current use, and assess some of the deleterious consequences of these confusions. We summarize that the distinction between basic and translational biomedical research is an anachronism. Elimination of this often contentious distinction would improve both the culture and the effectiveness of the scientific process, and its potential benefits to society.—Flier, J. S., Loscalzo, J. Categorizing biomedical research: the basics of translation.
There is power in language that often transcends the simplest of intentions in its construction. Such is the case for the term “translational research,” which is defined by the European Society of Translational Medicine as an interdisciplinary branch of biomedical science supported by 3 main pillars: benchside, bedside, and community ( 1 ). Defined in this way, translational research involves the application of scientific observations to the human condition, a process that involves many steps from conception of the problem to its ultimate application ( 2 ). “Basic research,” by contrast, refers to scientific research conducted without any particular practical purpose in mind a priori . There are, however, many nuances and confusions attendant to the use of these terms. To explore these distinctions and their implications for biomedical research, we should turn first to fundamental definitions
Research is based on intellectual investigation focusing on discovering, interpreting, and revising human knowledge of the world and as such, is a reflective endeavor. “Biomedical research,” as a subset of research is broad in scope, referring to activities spanning many disciplines of biology and medicine. Within these broad disciplines are experiments designed to understand reality by examining events at many different levels of organization, from the atomic level ( e.g. , structure of key biologic molecules), to the molecular and cellular levels ( e.g. , biochemistry, cell biology), to the organismal level ( e.g. , physiology and pathophysiology), and to the population level as well ( e.g. , population genetics, epidemiology, and public health). These domains are not tightly bounded: many fields of biomedical research, as self-defined or demarcated by professional organizations or academic departments, span many or even all of these levels of experimental inquiry.
Consider the discipline of neurobiology, with research addressing topics as diverse as the atomic structure of ion channels; signal transduction; development of the nervous system; systems properties of neural networks; the basis for the emergent properties of consciousness, cognition, and emotion; the molecular basis for diseases of the nervous system; and many others. Many such studies can be carried out in simple or complex models and increasingly in humans. Investigators can focus selectively on individual elements ( e.g ., ion channel structure and function), or integrate observations at multiple levels to answer a specific question. Consider a genetic disease of the nervous system in which a defined mutation causes a molecular alteration in a specific protein, the understanding of which requires studying the effects of the molecular defect on neuronal function ( e.g ., a channelopathy) and on complex neural circuitry ( i.e ., interneuronal communication) and behavior. Is there a clear line separating which component of such neuroscience research is basic and which is translational? The clarification of the system-wide (cellular or organismal) consequences of the mutation not only informs our understanding of disease pathogenesis but also informs the fundamental biology of the protein that could not be appreciated from studies of the protein in isolation.
Next, consider genetics, a field encompassing diverse, investigative efforts, spanning atomic resolution of DNA structure and DNA–protein interactions, the genetic basis for development, how changes in the genome cause altered function and disease, and the way in which genetic variation affects the fitness of populations. Each of these distinct aspects (and others) may be studied in different model systems, including organisms as diverse as yeast, worms, flies, mice, and most relevant to medicine, humans. Investigators interested in a specific biomedical problem ( e.g. , aging, metabolism) may carry out research spanning many of these levels of inquiry in more than one of these models. How can we distinguish basic from translational research in this context? Is research on the molecular details of DNA–protein interactions more basic than research on the role of DNA sequence variation in human health? Is research focusing on a specific protein in a simple organism more basic than research on the homologous protein in a human cell? Is a study at the atomic level more basic than a study of molecules, the latter more basic than a study of organelles and cells, and that, in turn, more basic than a study of complex organisms, just as some consider mathematics more basic than physics, physics more basic than chemistry, and chemistry more basic than biology? We think the answer to these questions is no.
Within all scientific endeavors, class distinctions can influence career choices and validate the perceived importance of one’s professional output. In a lecture one of us gives trainees on career development, a slide is presented, indicating one approach to hierarchies in science, in this case set by the importance and rigor of quantitative thinking in each discipline: pure mathematicians view themselves as scientifically superior to applied mathematicians and physicists, who view themselves as scientifically superior to chemists and biologists, who view themselves as scientifically superior to physician–scientists. This type of distinction between pure mathematicians and physicists was well illustrated by Peter Rowlett in a commentary in 2011 ( 3 ): In 1998, the engineer, Gordon Lang applied Thomas Hales’s 1970 solution to the Kepler conjecture (dating to 1611 and addressing the best way to pack spheres, which turned out to be the greengrocer strategy—6 in 2 dimensions, 12 in 3 dimensions, 24 in 4 dimensions, and 240 in 8 dimensions) to solve the problem of the optimal way to pack signals in transmission lines (modeled best as an 8-dimensional lattice). This solution opened up the internet for broad public use by maximizing the efficiency of signal transmission. When the mathematician Donald Coxeter, who helped Lang understand Hales’s mathematical solution, learned of Lang’s application, he was appalled that this beautiful theory had been sullied in this way. There are many other examples of this highly opinionated view of scientific hierarchies, not least of which is Ernest Rutherford’s comment that “all science is either physics or stamp collecting” ( 4 ).
Insofar as such self-affirming, hierarchical distinctions make us feel better about who we are, especially in a highly competitive environment, it is no wonder that the historical distinctions between basic and applied or translational research continue to exist in the minds of some faculty members, persisting well beyond their usefulness. When Michael Brown and Joseph Goldstein were awarded the Nobel Prize in Physiology or Medicine in 1985 for their work on cholesterol metabolism in which they identified the LDL receptor as defective in patients with familial hypercholesterolemia, many of us thought that the distinction between basic and applied biomedical research had become an anachronism and would (should) dissipate. To be sure, as modern medicine moved from an era of observation to the era of molecular biology, scientific questions, methods, analyses, and interpretations became increasingly conflated across the basic-applied spectrum. Clearly, both ends of the spectrum advance knowledge: basic investigation informs our understanding of pathobiology, and translational studies of disease mechanisms inform our understanding of basic biology. Examples of this latter point abound and have led to the New England Journal of Medicine series, “Basic Implications of Clinical Observations” ( 5 , 6 ). The Wall Street Journal contributor and author, Matt Ridley, has taken this perspective one step further and argued that basic scientific advances can be the consequence, rather than the cause, of applied technological advances (innovation) ( 7 ) ( e.g. , cryoelectron microscopy was developed to limit the consequences of radiation damage for biologic specimens and of structural collapse by dehydration under a vacuum; with the solution to these practical problems came a dramatic expansion of the field of structural biology, now to include high-resolution images of complex macromolecular structures that defied analysis by conventional X-ray crystallography and diffraction, and time-resolved changes in macromolecular structures or intermolecular interactions). Interpreted most generously, these examples illustrate that basic biomedical research and translational biomedical research have been coevolving successfully into a seamless continuum of investigation.
Given the diversity of questions and model systems being investigated within individual fields, can we identify criteria that might be used to facilitate labeling specific research activities as basic or translational? If so, this might clarify public discourse and enhance communication within the scientific community and between the scientific and lay communities.
POTENTIAL CRITERIA FOR CONSIDERING RESEARCH AS BASIC VS . TRANSLATIONAL
The identity of the institution and department in which the research is performed.
At most medical schools, many faculty members are members of what are institutionally denoted basic science departments, such as cell biology, genetics, biochemistry, and neurobiology, among others. Many other faculty members are based in school-affiliated hospitals and within departments in which the names reflect clinical fields, such as medicine, pediatrics, surgery, and neurology, among others. These organizational distinctions might suggest that faculty in basic science departments conduct basic research, whereas those in clinical departments, at least in the main, conduct applied translational or clinical research. But that is not always the case. In biomedical research today, much investigation takes place in academic health centers (or hospitals), and much of this work lies within clinical departments, such as medicine, pediatrics, and neurology. In some such departments, most of the research pursued is clinical research on human subjects, much of it involving the testing of therapies or devices. In other clinical departments, including those at our Harvard-affiliated institutions, research spans a broad array of topics, from general cellular mechanisms to disease mechanisms, and such research may also use organisms from worms and flies to mice and, of course, humans. Many researchers in these departments pursue research as a full-time or nearly full-time endeavor, many are not physicians, and substantial numbers might fit just as well, based on the work they do and where they publish it, in traditional basic science departments. For these reasons, we should not categorize research as being basic or translational based on the identity of the institution or department in which it is performed.
The motivation of the investigator
Should research qualify as basic because an investigator pursues a question purely for reasons of curiosity, without any interest in the potential practical applications of the work? Likewise, should research qualify as translational because an investigator is pursuing the solution to a practical biomedical problem, such as the treatment of a disease? Perhaps surprisingly, these differences in applicability or practical purpose are common distinctions used to define the following terms: “basic research” is conducted without any practical end in mind, although it may have unexpected results pointing to practical applications ( 1 ), whereas, “translational research” applies scientific observations to practical questions on the human condition.
Although many scientists choose to pursue particular questions ( e.g. , how a complex organism develops from a single cell, what molecular interactions determine cell division or death, how one nerve cell communicates with another, etc .), because they see them as challenging puzzles without consideration of practical applications, it seems unhelpful to label the research as basic or translational solely on the basis of the motivations of the scientists regarding potential, practical impact. A scientist may be motivated to pursue the same research question because of curiosity as to how the world works or because of an intuition that the understanding of a particular pathway or mechanism might lead to an understanding of and potential treatment for a disease. For example, one might study cell death pathways as a challenging biologic problem with relevance to developmental biology or because of a suspicion that such pathways might be relevant to cancer. Likewise, studies of adipocyte-specific gene expression in cell culture might be pursued as a means to understand regulated gene expression, or be motivated by a desire ultimately to understand obesity. Would such practical motivations on the part of the latter scientist render their inquiries less basic? We think not. Although research called basic today, often lacks a consciously applied, practical motivation, that seems not to be an essential reason to label the inquiry as basic. Likewise, research motivated by a desire to understand and eventually facilitate treatment of a disease should not, for that reason alone, be considered translational or less basic. Rather, one or more characteristics of the research itself should determine how it is categorized. This is distinct from the issue, raised by some observers, that current grant proposals force investigators to claim the potential impact of their work on future clinical application before such links are reasonable to assert ( 8 ).
The scientific importance of the work
Some discoveries, by virtue of their powerful capacity to change the way we think about an area, are more important and have greater generalizability and impact than others. Whereas some scientific observations provide new, explanatory frameworks and affect many unanticipated areas of inquiry, others provide more limited understanding, with little or no impact on other fields. The term basic could be used to describe research that is fundamental in this way. If this kind of importance or impact is what we mean when referring to research as being basic, then the term can be applied to many different kinds of research, from the purely theoretical to that dealing with events at the atomic, molecular, cellular, organismic, or population level. Likewise, by the criterion of scientific importance, basic research can involve organisms ranging from bacteria, to worms, to mice, or to humans, and some research on humans can readily be judged as more basic ( i.e. , more important) than some research on worms. Furthermore, molecular discoveries about a specific pathway that are novel but add incrementally to the field and lack impact outside it may be judged less basic in this sense than human studies, revealing new insights about a previously unexplained physiologic response or disease.
Thus, if the term basic is used to refer to research that is scientifically important, then disease-related research, wherever it is carried out, can be more (or less) basic than research conducted in basic science departments. At the very least, this analysis would suggest that the term basic is being used in different ways in these various situations.
Whether the research is disease related and/or conducted on humans or human tissues
Historically, research in basic science departments has not prominently involved human disease models or subjects (there are exceptions), and this fact (which could have evolved differently and might still in the future), combined with the fact that much human and/or disease-related research is conducted in hospitals, has created a cultural divide. In this context, it might not be surprising that research on humans would be viewed by some as less basic than research typically not involving humans or their tissues carried out in basic science departments. But does this idea, or related constructs, have merit? Research on human subjects or tissues may be basic, based on criteria of scientific importance, having great impact on understanding human biology and disease and leading to a fundamental understanding of general biologic principles. Of course, most researchers who conduct human studies are motivated by or are aware of potential applications to disease. Is this a sound basis for applying the term translational to the research activity rather than viewing it as basic? Perhaps so, provided that the use lacks hidden implications of relative scientific value. As departments redefine their scope of interests ( e.g. , departments of physiology focusing on whole-organ genomics in humans), these distinctions of purpose, application, and relative value truly become moot.
Beginning about a decade ago, the field of translational research was redefined and re-emphasized as the ultimate formalism for all biomedical research, regardless of the nature (purely basic or translational) of the initial scientific question posed. The intention of redefining the field of translation was both a noble one, emphasizing, as it does, our academic community’s efforts to apply fundamental observations to the human condition, as well as a practical one, serving to emphasize to our legislators, in an era of fiscal constraint, that even basic research funded by the U.S. National Institutes of Health (NIH) has (or may have) practical applications that may benefit human health. Yet, it appears that this type of nomenclature has had an unintended, divisive effect within the academic biomedical community. Why? The newfound emphasis on the translation of basic observations to the clinical arena evolved in the setting of a severely cost-constrained NIH budget and applied yet another criterion for the use of limited government funds ( viz. , the potential for clinical application of even the most fundamental finding). This mandate was established in the setting of an expanded pool of biomedical, newly minted Ph.D. researchers through the doubling of the NIH budget in the early part of this century. As an increased number of Ph.D.s began to seek support from a significantly constrained pool of NIH funds, they found that they also now needed to justify their proposals based on potential translation to clinical application, no matter the topic. As a result, growing concern arose in the basic biomedical community about society’s commitment to support research without express practical purpose. For those members of the community who have always believed, if not insisted, on the age-old hierarchical distinctions between basic and applied biomedical research, the ascendancy of translational medicine gave them pause. Furthermore, this distinction has now led some members of the basic community to argue for the need to enhance support for basic studies so that fundamental scientific investigation not be lost in translation ( 9 ). The push for (rapid) clinical application is viewed by some as overvaluing translation ( 10 ) and, furthermore, has led to conflation of the more clinical end of the translational research spectrum with the more fundamental end of that spectrum ( 11 ). Thus, this transition has had the unintended consequence of promoting the age-old, hierarchical distinctions between intellectually and methodologically equivalent research strategies in domains called basic and translational research, inapplicable, though they now are, for the reasons delineated above.
Thus, in a most unfortunate way, the excessive emphasis on translational research has led to a reversion to outdated approaches as to how biomedical investigators relate to one another. We feel strongly that there is no place for these artificial distinctions in today’s biomedical research enterprise. They are intellectually limiting and in the worst case, create artificial barriers to sharing ideas and resources.
Summary interpretation of bases for categorizations
Based on the foregoing analysis, we think it is fair to conclude that use of the terms basic or translational biomedical research should not be dependent on the following criteria: 1 ) whether the scientist pursuing it is motivated by a desire for the work to have practical impact; 2 ) the scientific importance of the work; 3 ) the level of inquiry ( e.g. , atomic, molecular, cellular, physiologic, community); or 4 ) the name of the department in which the work is pursued. Yet, the question remains: is there an unambiguous use of the terms that can be widely accepted and, most important, advance the cause of the scientific enterprise?
The most meaningful distinctions that arise when describing and categorizing biomedical research relate to two specific issues: the precise question being asked and the approach used to address the question. The scientific questions can be broad in scope, ranging from studies of single molecules to populations of human subjects and from normal mechanisms to mechanisms of disease pathogenesis. Likewise, the methodological approaches applied can be equally broad, ranging from structural studies of single molecules to statistical genetic analysis of variant allele frequencies in populations of human subjects, with or without a disease phenotype. Each line of inquiry and experimental strategy can produce scientific results that are more or less important, with their importance only reliably ascertainable with the passage of time; can be motivated by a desire to produce practical/applied results or not; and can be performed by individuals conducting research in what we now call basic or clinical departments. Thus, the formation of distinctions between basic and translational research is not especially useful in the current era. This distinction is an anachronism that can best be appreciated by understanding its historical origins.
HISTORICAL AND CULTURAL PERSPECTIVES UNDERLYING THE CATEGORIZATION OF BIOMEDICAL RESEARCH
For much of history, and, importantly, in 19th century Europe, a bright line was drawn between fundamental scientific inquiry and applied research. Whereas the former was carried out by professors in universities, the latter was often conducted in industry. Some of the greatest scientists and discoveries arose at the interface between fundamental and applied research—witness the discoveries of Pasteur, Langmuir, and those related to atomic energy. In prior years, when medicine had an extremely circumscribed, scientific basis, the limited research that existed was conducted by university professors, whereas clinicians pursued their work in a relative scientific vacuum. As biomedical science developed in the 20th century and especially following World War II, with the growth of research within academic health centers and hospitals, the line between basic and applied biomedical research, as defined in the preceding century, became much less relevant.
Over the past 50 years, the growth of funding for biomedical research and the opportunities created by research advances in cell and molecular biology stimulated clinical departments to expand their research operations. This growth was especially robust during periods with clinical financial surpluses and increasing levels of NIH funding. Both M.D. and Ph.D. researchers were recruited into clinical departments, and research techniques used there progressively included approaches similar or identical to those used in basic science departments, such as gene cloning, transgenic animal studies, etc . Whereas, much of this work might also have been situated within traditional basic science departments of medical schools and universities, many hospitals and clinical departments aggressively pursued it as well, although the faculty typically remained associated with departments bearing clinical names. Depending on the school or university, such faculty may or may not also have appointments in basic science departments.
The role of the biotechnology and modern pharmaceutical industries in the historical evolution of biomedical research categorization also warrants comment. With the passage of the Bayh-Dole Act and the explosion of biotechnology and pharmaceutical industry interactions with academic institutions, another aspect of basic research must be reconsidered. Scientists who might previously have viewed their work as unrelated to practical outcomes ( e.g. , fundamentals of cloning and eukaryotic gene expression) realized the potential for their discoveries to be applied to human therapeutics, and many of these basic scientists turned their attention aggressively to pursuit of these goals, including moving into the commercial sphere. In this way, another barrier between basic and translational research collapsed. Among faculty in basic science departments who pursue research in cell biology, genetics, or neuroscience, some continue to pay little attention to the practical implications of their work. Others, doing identical or substantially similar work, are constantly on the hunt for the practical implications of their studies that might be patented and exploited, within or outside of the academy, for eventual diagnostic and therapeutic uses. Whereas the former scientists might prefer that their work be described as basic (and devoid of practical use), the latter might proudly describe their (substantially similar) work as having translational or even clinical implications.
CONCLUSIONS
In the end, does it matter whether we call some biomedical research basic or translational? If we understand what the particular research is about and grasp its content, context, and implications, probably not. However, current use promotes confusion and hampers efforts to promote scientific understanding and collaborations across our diverse, creative academic biomedical research enterprise. We should clarify or modify the use of these terms and consider a new, cohesive classification nomenclature that realistically reflects the contemporary biomedical research environment. The descriptive analysis of biomedical research should focus on perceived quality as objectively assessed as possible ( 12 ), rather than the promotion of a self-affirming, anachronistic distinction. Perhaps, instead of use of the term basic to describe particular entities of biomedical research, our descriptive terminology should reflect one of the alternate meanings of the term, namely, essential or vital. These terms could apply to all biomedical research that is effective in creating new knowledge, because such research is essential for the growth of the biomedical enterprise for the benefit of society, either directly or remotely. The elimination of the unnecessary distinction between basic and translational biomedical research (and researchers) would improve the effectiveness of the scientific process and its potential benefits for the society to which its practitioners are ultimately obligated.
Discovery is optimall promoted by reliance on talented investigators, whatever their philosophical scientific persuasion ( 13 ). Particularly in an era of big data and interdisciplinary research, a nonhierarchical atmosphere of collegiality and mutual support is required for optimal success. The mandate that all research be framed as offering a potential for translation to clinical benefit is misguided, misleading, and disingenuous. Legislators should be educated about the nature, natural history, and goals of modern biomedical research, wherein anyone with a good idea and the skills necessary to realize it has the potential to add value to the enterprise and benefit to society.
ACKNOWLEDGMENTS
This work was supported, in part, by U.S. National Institutes of Health (NIH) National Heart, Lung, and Blood Institute Grants HL61795, HL119145, and NIH National Institute of General Medical Sciences Grant GM 107618 (to J.L.). The authors thank Stephanie Tribuna (Brigham and Women’s Hospital) for expert secretarial assistance.
AUTHOR CONTRIBUTIONS
J. Flier and J. Loscalzo both conceived of the ideas presented, and jointly wrote and edited this article.

Pennington Biomedical Researcher Dr. Carrie Elks Awarded $1.4 Million to Study Fat Cell Communication Cycle
Dr. elks and her matrix biology laboratory at pennington biomedical recently discovered a unique fat cell to immune cell communication loop .
August 5, 2024 · Baton Rouge, LA
The National Institutes of Health’s National Institute of Diabetes and Digestive and Kidney Diseases, or NIDDK, along with the National Institute of General Medical Sciences, or NIGMS, awarded Pennington Biomedical researcher Dr. Carrie Elks a grant of $1.4 million to explore insulin resistance and inflammation in fat cells. Dr. Elks will apply the grant toward her Matrix Biology Laboratory’s project “Adipocyte cytokine signaling as a coordinator of adipose tissue function.”
The proposed project is based on a communication loop that was recently discovered by Dr. Elks and her team. The bidirectional communication loop is between fat cells and immune cells. Interruptions to this loop promote obesity and reduces function of fat tissues. The loop is governed by a small protein called Oncostatin M, or OSM, and the project will further explore how the protein and its communication loop operate in maintaining body weight.
“I’m grateful that the NIDDK has evaluated this discovery and our planned research to be worthy of this generous grant,” said Dr. Elks, assistant professor-research at Pennington Biomedical Research Center and LSU alumna. “The communication between fat cells and immune cells is crucial for fat tissues to work properly, and the ways in which fat cells respond to immune cells can change, resulting in inflammation. The results of this study will ideally reveal new patterns in cell communication that can be further evaluated to treat obesity and other metabolic diseases.”
The OSM protein is produced by immune cells in fatty tissue, but the lab’s research shows that when fat cells lack the receptor for OSM, this leads to fat tissue inflammation and insulin resistance. The lab’s findings suggest that signals from the OSM protein are needed for fat tissues to function properly, but how such an interruption can trigger inflammation and insulin resistance remains to be seen.
Dr. Elks and her team will test if fat cells regulate the amount of free cytokine proteins to maintain homeostasis in fat tissue. They will test to see if continuous exposure of fat cells to OSM makes them less responsive to OSMR, thus causing them to become resistant to insulin.
“I congratulate Dr. Elks on her lab’s recent discovery and the subsequent award to continue exploring it,” said Dr. John Kirwan, executive director of Pennington Biomedical. “This research is a perfect example of our ‘cells to society’ mantra, as we seek to explore the roots of obesity and open new avenues for how to best prevent and treat it.”
Dr. Elks’ Matrix Biology lab studies communication networks on the cellular level that have been linked to insulin resistance, type 2 diabetes and a worsening of Crohn’s Disease in humans. The lab works to uncover methods to regulate adipose tissue function with the ultimate goal of developing better treatments for patients with metabolic diseases.
For more information contact:
Joe Coussan, Media Relations Manager, [email protected] , 225-763-3049 or Ernie Ballard, Senior Director of Communications & Marketing, [email protected] , 225-263-2677 .
About the Pennington Biomedical Research Center
The Pennington Biomedical Research Center is at the forefront of medical discovery as it relates to understanding the triggers of obesity, diabetes, cardiovascular disease, cancer and dementia. The Center conducts basic, clinical, and population research, and is a campus of the LSU System. The research enterprise at Pennington Biomedical includes over 530 employees within a network of 44 clinics and research laboratories, and 13 highly specialized core service facilities. Its scientists and physician/scientists are supported by research trainees, lab technicians, nurses, dietitians, and other support personnel. Pennington Biomedical is a state-of-the-art research facility on a 222-acre campus in Baton Rouge. For more information, see www.pbrc.edu .
Pennington Biomedical Research Center 6400 Perkins Road Baton Rouge, LA 70808
Centers & Institutes

Metabolic Basis of Disease Center
Institute for Dementia Research & Prevention
Louisiana Clinical & Translational Science Center
Nutrition Obesity Research Center
Metamor Institute
Center for Military Performance & Resilience
Childhood Obesity and Diabetes Research
Human Research Protections Program
Research Kitchen + Recipes
Business Development
LAUNCHED Program
Pennington Biomedical Research Foundation
Our Partners
Obesity Society
American Diabetes Association

Sign Up For Updates
6400 Perkins Rd. Baton Rouge, LA 70808 ph: (225) 763-2500 fax: (225) 763-3022
© Copyright Pennington Biomedical Research Center
Share Options
- Share to Facebook
- Share to Linkedin
UVA Engineering Professor Lands $1.9 Million NIH Award To Maximize Biomedical Research
Cai’s Lab Is Pioneering a Path To Repairing Cells, Building Organ Structures
University of Virginia assistant engineering professor Liheng Cai learned Tuesday that he had earned the Maximizing Investigator’s Research Award from the National Institutes of Health.
Cai’s work holds significant implications for the future of personalized biomedicine, including the possibility of repairing living tissue, perhaps even replacing whole organs.
The NIH honor, bestowed by its National Institute of General Medical Sciences, signals its support for researchers performing breakthrough research. The associated grant will infuse his lab at the UVA School of Engineering and Applied Science with $1.9 million over the next five years.
The grant will allow Cai to further his study of tissue repair and disease progression through the advanced living cellular structures his team in the Soft Biomatter Lab creates. Cai holds a joint appointment in the Departments of Chemical Engineering and Materials Science and Engineering, and a courtesy appointment in the Department of Biomedical Engineering..
The mechanical properties of the modules will mimic the mechanical properties of human tissue.
For now, the structures exist outside of the human body. But the lab will continue to improve upon how the structures mimic human biology. One of Cai’s major aspirations is to someday integrate healthy, bio-printed cells into the human body.
“One of the major components of the award is to develop biomaterials that can be constructed from tiny, basic modules,” said Cai, who joined the UVA Engineering faculty in 2018. “The mechanical properties of the modules will mimic the mechanical properties of human tissue. This approach is built on a class of polymers — bottlebrush polymers — my lab has been pioneering.”
He added, “I would like to thank the Institute for this very substantial investment and show of faith in our lab’s potential. I credit the superb, supportive environment here at UVA for making this award possible.”
The award builds on his National Science Foundation CAREER Award and ACS Petroleum Research Fund Doctoral New Investigator Award . He also received the UVA Research Excellence Award last year.
The new announcement raises Cai’s sponsored funds at UVA to over $4 million.
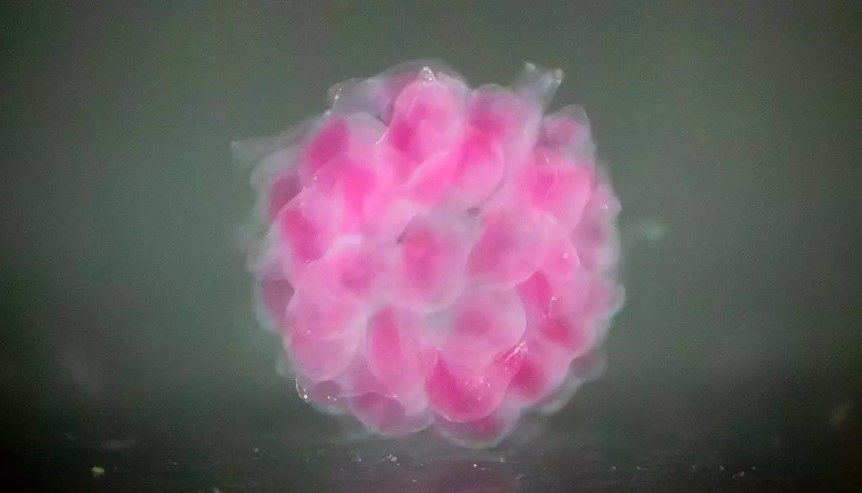
Bioprinting Inspired by Minecraft®
His lab’s new polymer — named for its special architecture resembling a bottlebrush for cleaning glassware — can be chemically or physically linked to form extremely soft material that mimics biological tissues.
These tissue mimics are also part of the team’s strategy to make 3D printing of biological materials possible.
Cai’s lab recently proved the concept for “voxelated bioprinting ." It’s a form of programmable cell assembly inspired by the online game Minecraft®, Cai noted.
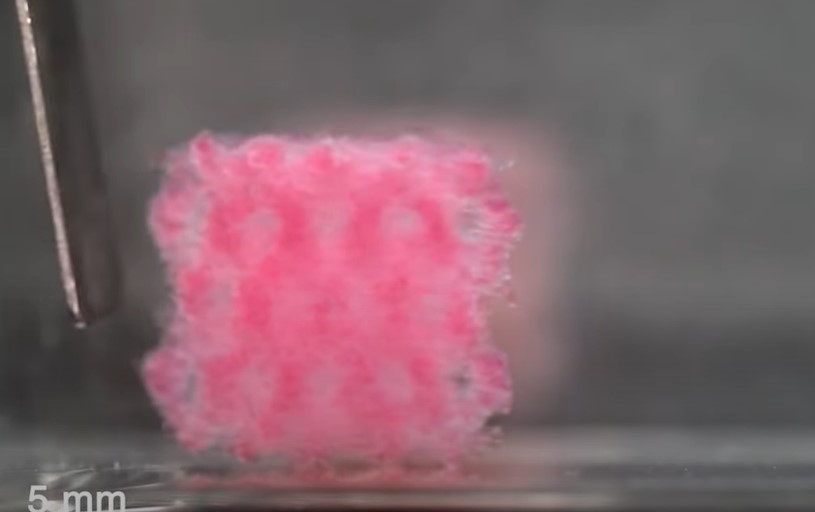
“The popular video game uses individual 3D cubes as ‘voxels’ to create a virtual world,” he said. “We developed voxelated bioprinting technologies in the real world. Our technology assembles cell-encapsulated droplets into a specially designed printer. During the printing process, we create 3D cell assemblies with the exact architecture and functions that we programmed.”
Cai believes that this technology could pave the way for printing organs on demand — truly personalized medicine.
He said he and his team are “extending our design strategy to engineer modular cell-instructive biomaterials.” They believe the bottlebrush architecture holds the potential for encoding the complexities that exist in our bodies’ own various tissues.
En route to that goal, the lab aspires to print 3D cell-based models that function similar to human tissue, so as to study disease progression. In one project, the team is creating what Cai calls a “micro-human airway device” to capture the geometric and biological features of human airways.
“We are investigating the interactions between mucus and three indispensable components of the microenvironment: cilia, cells and bacteria,” he said. “The idea is to exploit this biologically similar device to study human lung defense, without having to cause harm to living beings.”
First Organs Might Be Vocal Cords
Cai’s current career journey has been built on his other explorations into the fundamental nature of polymers, which can be defined by their source (natural or synthetic), chemical structure or method by which they’re made.
Natural polymers are integral to our bodies. They are essential for maintaining the structure, function and regulation of various tissues and organs. Their ability to self-assemble and interact with other molecules and cells allows them to perform complex biological functions that are crucial for life.
A goal of Cai’s has been to learn how cells best respond over time to precision treatments using his specially engineered polymers. He and his team recently developed “ soft-yet-dry elastomers ,” which are very stretchable and soft polymers.
Cai also noted the striking similarity between the material and human vocal cords , which currently can’t be replaced if they are too severely damaged.
Soft-yet-dry elastomers could potentially have many uses though, he said, including as more durable and compliant medical devices, and in wearable electronics and soft robots.
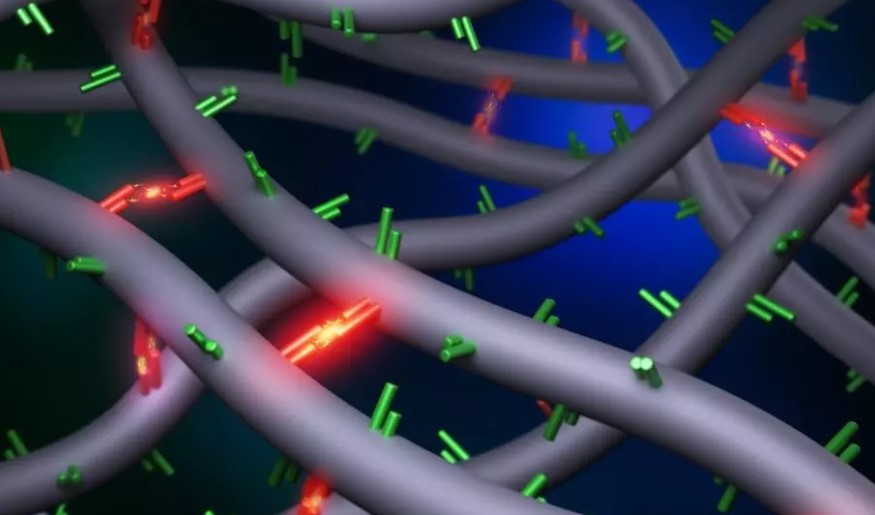
Creating ‘Self-Healing’ Polymers
Cai has also extensively researched associative polymers, which can be water-soluble and are found within the natural biopolymers in the extracellular matrix that surrounds and supports cells and tissues in the body. They carry many “stickers,” or chemical structures that form easy-to-break, reversible bonds, Cai explained.
Researchers have long been in pursuit of understanding the stickiness attribute because they believed this function held the key to recreating biomaterials that match the behavior of living tissue.
In a related research project, Cai and colleagues recently discovered through experiments that such stickiness is controlled by the density of the bonding structures, upending more than three decades of theory .
Polymer physicists had previously believed that the mechanical behavior of associative polymer networks — including their stickiness and reversibility — was governed by the sticker bond strength. The scientists thought so because of what they observed. When bonding associations are maintained, a shape change called a “rubbery plateau” occurs.
Cai’s team had to create new classes of associative polymers, “carrying unprecedentedly high number of stickers,” he said, to prove their own theory.
“We experimentally showed that reversible bonds significantly slow down the polymer dynamics but hardly change shape as they relax over time ,” Cai said related to the influential June 2023 paper in Physical Review Letters . He collaborated with Shiwang Cheng of Michigan State University and others on the paper.
“This new discovery is critical to the development of biomaterials that match how biological tissues respond to stress over time,” Cai added.
Putting It All Together
Cai’s department heads said they have been excited to see the elements of his MIRA research come together: a modular bottlebrush structure underlying new biomaterials and a bioprinting technology that can precisely assemble the materials to create 3D tissue mimics.
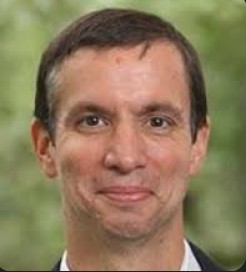
There’s no doubt in my mind that Liheng’s research in polymers and soft matter will impact, and ultimately lead to, the development of designer biomaterials that guide cell function.
William Epling , chair of the Department of Chemical Engineering , congratulated Cai on his award and shared his thoughts on the significance.
“There’s no doubt in my mind that Liheng’s research in polymers and soft matter will impact, and ultimately lead to, the development of designer biomaterials that guide cell function,” Epling said.
Epling is an expert in materials characterization and surface and interface science, among other facets of chemical engineering.
Elzabeth J. Opila , chair of the Department of Materials and Science Engineering , said, "Professor Cai is doing transformative work that bridges materials science, soft materials and biomedical engineering. I'm looking forward to the breakthrough science enabled by this prestigious award.”
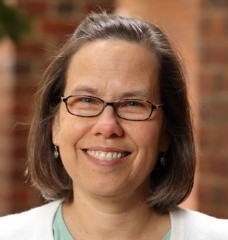
I'm looking forward to the breakthrough science enabled by this prestigious award.
The award follows a “Rising Star” designation Cai and fellow UVA Engineering assistant professor of chemical engineering Rachel Letteri received in March. ACS Polymers Au featured Cai and Letteri as just two of 14 early-career researchers from around the world who are leaders in polymer science and engineering.
He also recently received the 2024 PMSE Early Investigator Award and the 2020 Soft Matter Emerging Investigator Award .
Cai’s research dovetails with the Precision Medicine/Health component of the University’s “ Grand Challenges ,” which exist to help solve some of the world’s most urgent problems.
Explore the UVA Soft Biomatter Lab
Read more about liheng cai's work.
- Medicine >
- Research >
- Research Highlights >
UB Awarded $3.6 Million NIH Grant to Address Western New York Health Inequities

Timothy F. Murphy, MD, speaks during a news conference announcing a $3.6 million National of Institutes grant awarded to the University at Buffalo to address health inequities in Western new York.
Early Career-Faculty Will Address Root Causes of Inequities in Buffalo Area Communities
By Ellen Goldbaum
Published August 6, 2024
BUFFALO, N.Y. – The University at Buffalo has been awarded a highly competitive, $3.6 million grant from the National Institute on Minority Health and Health Disparities to train early-career faculty members to address health inequities in Western New York.
Both UB’s strong community partnerships and the university’s range and breadth of expertise were instrumental in getting the award, university leaders said.
The five-year grant from the institute, part of the National Institutes of Health, aims to inspire and mentor early-career faculty researchers from a wide range of academic disciplines to address the entire range of social determinants of health. This includes poverty, substandard housing, unequal access to health care, lack of educational opportunity, racism and more.
UB will use the award to establish the Center of Excellence in Investigator Development and Community Engagement, which will be embedded in the university-wide Community Health Equity Research Institute . The center will encourage and support research that benefits people who experience health inequities caused by adverse social determinants of health.
“Today’s $3.6 million grant will help ensure all Western New Yorkers have access to the quality health care they need and deserve, no matter their means or background,” U.S. Rep. Timothy M. Kennedy said. “It will provide UB with the resources it needs to recruit and develop the next generation of researchers to better understand the social disparities that impact the health care and services people rely on in order to level the playing field. This is just another example of the ways Western New York is becoming a better and more equitable place to live, work, and raise a family — for everyone.”
Allison Brashear, MD, MBA , vice president for health sciences and dean of the Jacobs School of Medicine and Biomedical Sciences , said ”This award underscores UB’s unwavering dedication to enhancing the health of the Buffalo community. Leveraging UB’s robust expertise in health sciences and our extensive research capabilities, we are fully committed to advancing health outcomes.”
She added: ”Through our strong partnerships with numerous community organizations, we believe we can transform the health landscape of our region. Although this award is centered at UB, its true purpose is to empower and transform the health of Buffalo and Western New York.”
New York State Assembly Majority Leader Crystal Peoples-Stokes said, “In too many cases, patients in our community needlessly suffer not because there’s a lack of medical knowledge, but because the patient’s voice wasn’t heard. Ultimately, I see the work of this center as raising up the voices and experiences of patients so that they can live happier, healthier lives.”

From left, Kelly Wofford, Rev. George F. Nicholas, NYS Assembly Majority Leader Crystal Peoples-Stokes, U.S. Rep. Timothy M. Kennedy, Allison Brashear, MD, MBA; and Timothy F. Murphy, MD, at the Aug. 6 event.
Problems Go Beyond Health Care Access
The grant, titled “Igniting Hope in Buffalo, New York Communities: Training the Next Generation of Health Equity Researchers,” provides UB with resources to attract early-career faculty researchers and postdoctoral fellows from health care disciplines, as well as non-health care fields, to work on problems that impact the social determinants of health. The center will utilize a “community-based participatory research” approach, where community members are a partner in the research, helping to design, plan and conduct the research so that they can gain the greatest benefit from it.
While advances in medical interventions have dramatically accelerated in recent years, such interventions cannot overcome the systemic inequities that are so deeply rooted in complex social systems. For that reason, the center will prioritize attracting investigators working in fields outside of health care to address, for example, inequities in the criminal justice system, substandard housing, access to healthy food and many other issues.
“Adverse health outcomes are a result of these social determinants of health” said Timothy F. Murphy, MD , principal investigator on the grant, SUNY Distinguished Professor of medicine and director of UB’s Community Health Equity Research Institute. “If we could solve all health care access problems, we’ll still only change health outcomes in our at-risk communities by about 15%. What we are trying to do with this grant is to attract researchers in non-health-care disciplines, who are working in urban planning or education or law or management, and to make them aware that their work is absolutely critical to solving health disparities in Western New York.”
“Health inequity remains a serious and complicated issue in Buffalo and its surrounding communities,” said state Sen. Sean M. Ryan. “To solve this crisis, we must understand its root causes and develop innovative solutions. I am pleased to support the University at Buffalo and community leaders who are addressing this urgent problem, and look forward to building stronger and healthier communities in Western New York.”
Strong Community, Government Partnerships
In 2019, UB established the Community Health Equity Research Institute with strong community involvement. In 2021, Erie County established its Office of Health Equity. That same year, UB’s Center for Urban Studies partnered with the Community Health Equity Research Institute, linking the institute to urban planning and neighborhood development.
These connections have further cemented local, coordinated university-community-government efforts to address health inequities, some of which may depend on legislative and policy changes.
In a reflection of those connections, Rev. George F. Nicholas, CEO of the Buffalo Center for Health Equity is associate director of the UB Center of Excellence in Investigator Development and Community Engagement. He said that without research, many well-intentioned efforts don’t have the expected benefit.
“The work of health equity has got to be data-driven,” he said. “A lot of work that is done to address social problems often gets tied up in theoretical approaches or philosophical approaches and history has taught us while they may do some good, they don’t bring real change. Research gives us a clear picture on the depth of the problems and what is driving them. When we better understand the scope of the problems and what’s driving them, then we can develop approaches and remedies to solve them.”
Center to Support Community-Focused Projects
With the grant, UB will provide faculty researchers and postdoctoral fellows with pilot funding for projects with community partners that have the potential to generate transformational change. These changes will not be quick fixes, Murphy cautioned.
“A Black person in Buffalo dies 10 to 12 years younger than a white person in Buffalo,” Murphy said. “That’s a tragedy and unfortunately it’s not one that’s going to change anytime soon. But what if a researcher develops a green infrastructure project that actually reduces pollution in a neighborhood and that then causes a drop in the number of kids with asthma who end up in the emergency room. That’s going to have a bigger outcome down the road, kids won’t be missing school, their education improves. That’s the kind of change we’re going to see with this new research.”
The center could even change how research is viewed in the community, Nicholas added, and it will encourage people in at-risk communities to get involved.
“You don’t hear a lot of young people say, ‘Hey, I want to be a researcher,’” he said. “But we want to make being a researcher not only a possibility but make it attainable and desirable, to say ‘This is something you could do that will have an impact on your community, on the neighborhood you live in.’”
Media Contact Information
Ellen Goldbaum News Content Manager Medicine Tel: 716-645-4605 [email protected]
Core Facilities

- Policy Guidance
- Submitting Data
- Accessing Data
- About the Genomic Data Sharing (GDS) Policy
- Key Documents
- Preparing Genomic Data
- Extramural Grantees
- Non-NCI Funded Investigators
- Intramural Investigators
- Accessing Genomic Data
- Genomic Data Sharing Policy Contact Information
- Data Standards Services
- Cloud Resources
- Data Commons Framework
- Repositories
- ARPA-H BDF Toolbox
- Accelerating Therapeutics for Opportunities in Medicine (ATOM)
- NCI-DOE Collaboration AI/ML Resources
- NCI-DOE Publications
- U.S.-EU Artificial Intelligence Administrative Arrangement
- NCI Data Catalog
- CDISC Terminology
- FDA Terminology
- NCPDP Terminology
- Pediatric Terminology
- Metadata for Cancer Research
- Informatics Technology for Cancer Research (ITCR) Tools
- Generating and Collecting Data
- Cleaning Data
- Exploring and Analyzing Data
- Predictive Modeling
- Visualizing Data
- Sharing Data
- Cancer Data Science Course
- Training Guide Library
- Cancer Data Science Pulse Blog
- Data Science Seminar Series
- Jobs and Fellowships
- Contact CBIIT
- Organization
- CBIIT Director
- Staff Directory
- Application Support
- Genomic Data Sharing
- NCI-Department of Energy Collaborations
- Cancer Vocabulary
- Learn About Cancer Data Science
- Improve My Data Science Skills
Get an NIH Salary Award So You Can Develop Research Software
If you’re currently in a non-tenure track position at an academic or research institution, you can apply for funding to develop your career as a Research Software Engineer (RSE). As the grant recipient, you’ll serve as the principal investigator (PI) for up to three years. You’ll have the opportunity to expand your knowledge in the development and dissemination of biomedical, clinical, behavioral, or health-related research software, tools, and algorithms.
To be an ideal candidate, you must:
- have held a full-time, non-tenure track position for at least one year.
- demonstrate outstanding software engineering research expertise with relevant publications, peer-reviewed conference proceedings, and links to code samples.
- commit to a minimum effort of six “person-months” (i.e., number of people multiplied by the number of months they work).
- not have served as the contact PI for any independent NIH grant applications in the past five years.
NIH aims to update the data ecosystem by building sustainable software foundations for open software and tools in biomedical, clinical, behavioral, and health-related research. This effort aligns with the NIH Strategic Plan for Data Science and encourages the adoption of solid software development practices, new data science technologies, cloud and hybrid computing, artificial intelligence, and open science guidelines.
SUBSCRIBE TO UPDATES

IMAGES
COMMENTS
Biomedical research is the broad area of science that looks for ways to prevent and treat diseases that cause illness and death in people and in animals. This general field of research includes many areas of both the life and physical sciences. Utilizing biotechnology techniques, biomedical researchers study biological processes and diseases ...
The University of Florida Cancer and Genetics Research Complex is an integrated medical research facility. Medical research (or biomedical research ), also known as health research, refers to the process of using scientific methods with the aim to produce knowledge about human diseases, the prevention and treatment of illness, and the promotion ...
Basic biomedical research, which addresses mechanisms that underlie the formation and function of living organisms, ranging from the study of single molecules to complex integrated functions of humans, contributes profoundly to our knowledge of how disease, trauma, or genetic defects alter normal physiological and behavioral processes. Recent advances in molecular biology techniques and ...
What is Biomedical Research? Biomedical research is the pursuit of answers to medical questions. These investigations lead to discoveries, which in turn lead to the development of new preventions, therapies and cures for human and veterinary health. Biomedical research generally takes two forms: basic science and applied research.
Biomedical sciences are a set of sciences applying portions of natural science or formal science, or both, to develop knowledge, interventions, or technology that are of use in healthcare or public health. [1] Such disciplines as medical microbiology, clinical virology, clinical epidemiology, genetic epidemiology, and biomedical engineering are ...
Biomedical Research: The area of science devoted to the study of the processes of life, the prevention and treatment of disease, and the genetic and environmental factors related to disease and health. Basic or "pure" Research: Research conducted to increase the base knowledge and understanding of the physical, chemical, and functional ...
The goal of basic biomedical research is to provide comprehensive and detailed understanding of the mechanisms that underlie the development and normal function of humans and other living organisms and thereby gain insights into the pathological and pathophysiological mechanisms that cause disease. A detailed understanding of these mechanisms and pathways is essential for identifying potential ...
Biomedical scientists bridge the gap between the basic sciences and medicine. The PhD degree is the gateway to a career in biomedical research. Biomedical scientists: Think outside the box and are innovators. Are critical and analytical thinkers. Get excited by discovering new things. Look at biology and see previously unrecognized patterns.
Long description. Biomedical research focuses on understanding how every part of the human body works—right down to our cells. By studying the normal and abnormal workings of the body at the molecular, cellular, organ system, and whole-body levels, biomedical research leads to new: Interventions to prevent illness in the first place.
WHAT IS BIOMEDICAL RESEARCH? Biomedical research focuses on understanding how every part of the human body works— right down to our cells. By studying the normal and abnormal workings of the body at the molecular, cellular, organ system, and whole-body levels, biomedical research leads to new: Ways of identifying and diagnosing disease
What is a Biomedical Scientist? Biomedical scientists uses scientific methods to investigate biological processes and diseases that affect humans and animals. They conduct experiments, analyze data, and interpret findings to improve our understanding of diseases and develop new treatments and cures. They also ensure the safety and efficacy of drugs and medical devices through clinical trials ...
In the history of biomedical sciences, almost all of the medical breakthroughs occurred in past hundred years that have remarkably revolutionized this field. The ultimate goals of biomedical research is to discover advanced approaches that help in making human health better, lessening the existing ailments and increasing life spans.
To achieve its goal of turning discovery into health and to maintain its role as the world's premier biomedical research agency, NIH must support the best scientific ideas and brightest scientific minds. That means looking to the future and ensuring that we have a strong and diverse workforce to catalyze discoveries in all fields of biomedicine including emergent areas like data science.
As the nexus among health, behavior, and medicine, the brain is vital in extending human life and enhancing well-being. Intensive study of the cellular and molecular processes that control our thoughts and actions, combined with the use of animal models in neurological research, have produced key insights into how the brain works and the development and treatment of neurological disorders.
Our North Carolina Affiliate, NCABR, has compiled a useful overview explaining what biomedical research is and how it works. Basic questions and answers, information on protection of human subjects, and overview articles about clinical trials. Summarizes what a clinical trial is, how trials proceed, and other related topics from the Multiple ...
Biomedical Research. Biomedical research is research with the goal of understanding normal and abnormal human functioning, at the molecular, cellular, organ system and whole body levels, including development of tools and techniques to be applied for this purpose; developing new therapies or devices that improve health or the quality of life of ...
Biomedical research is the broad area of science that involves the investigation of the biological process and the causes of disease through careful experimentation, observation, laboratory work, analysis, and testing. Scientists expand this knowledge base to discover ways to prevent ill-health, and to develop beneficial products, medications ...
Biobanks and biomedical research. Biomedical research is a key activity, both in terms of advances in basic knowledge of diseases and in the development of methods of diagnosis, prevention, and treatment to improve people's quality of life and life expectancy. Due to the incalculable value of biological samples of human origin in this type of ...
What is Biomedical Research Biomedical research is the study of human physiology and the treatment or understanding of disease.
Clinical biomedical researchers analyze biological material in laboratories in hospitals, clinics or as part of research teams. Biomedical engineers design equipment that can interface with the human body, including prosthetics. At Rush all these professionals and more work together on integrated teams to jointly solve important health problems.
Motivated by a recent trend that advocates a reassessment of the aim of medical science and clinical practice, this paper investigates the epistemic aims of biomedical research. Drawing on contemporary discussions in epistemology and the philosophy of science, along with a recent study on scurvy, this paper (1) explores the concept of understanding as the aim of scientific inquiry and (2 ...
Biomedical researchers are at the forefront of scientific innovation, seeking answers to the world's most pressing questions in human health. For 37 years, The Pew Charitable Trusts has encouraged these pursuits by supporting promising, early-career biomedical scientists tackling these challenges and driving medical breakthroughs.
Biomedical research is the broad area of science that looks for ways to prevent and treat diseases that cause illness and death in people and in animals. This general field of research includes many areas of both the life and physical sciences. Utilizing biotechnology techniques, biomedical researchers study biological processes and diseases ...
This program, crafted jointly by the Denmark-based NNF and Cleveland Clinic, is dedicated to integrating quantum technologies and AI into biomedical research and patient care, propelling the application of these technologies in medical science and healthcare.
Such is the case for the term "translational research," which is defined by the European Society of Translational Medicine as an interdisciplinary branch of biomedical science supported by 3 main pillars: benchside, bedside, and community ( 1 ). Defined in this way, translational research involves the application of scientific observations ...
The National Institutes of Health's National Institute of Diabetes and Digestive and Kidney Diseases, or NIDDK, awarded Pennington Biomedical researcher Dr. Carrie Elks a grant of $1.4 million to explore insulin resistance and inflammation in fat cells. Dr. Elks will apply the grant toward her Matrix Biology Laboratory's project "Adipocyte cytokine signaling as a coordinator of adipose ...
UVA Engineering Professor Lands $1.9 Million NIH Award To Maximize Biomedical Research UVA Engineers Make the World a Better Place: Pursuing better health care, designing the future of technology and creating sustainable solutions to society's challenges.
The University at Buffalo has been awarded a highly competitive, $3.6 million grant from the National Institute on Minority Health and Health Disparities to train early-career faculty members to address health inequities in Western New York.
The funding opportunity aims to support Research Software Engineers in developing and disseminating biomedical, behavioral, or health-related software, tools, and algorithms.
When is Lifecore Biomedical's next earnings announcement? View the latest LFCR earnings date, analysts forecasts, earnings history, and conference call transcripts.